
behaviors of polyethylene glycol-b-poly(β-benzyl
L-aspartate), INTERNATIONAL JOURNAL OF ENGINEERING RESEARCH & TECHNOLOGY (IJERT) Volume 09, Issue 02 (February 2020),
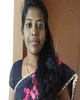
- Open Access
- Authors : Delphine , M. Murugan , M. Sivanantham
- Paper ID : IJERTV9IS020420
- Volume & Issue : Volume 09, Issue 02 (February 2020)
- Published (First Online): 12-03-2020
- ISSN (Online) : 2278-0181
- Publisher Name : IJERT
- License:
This work is licensed under a Creative Commons Attribution 4.0 International License
Effects of phenolic resin on the self-assembly
behaviors of polyethylene glycol-b-poly(β-benzyl
L-aspartate)
Delphine, M. Murugan, M. Sivanantham*
Department of Physics,
Centre for Research and Development, PRIST Deemed to be University, Vallam, Thanjavur – 613403, Tamil Nadu, India
Abstract: Effects of phenolic resin on the self-assembly behaviors of polyethylene glycol-b-poly(-benzyl L-aspartate) (PEG-b-PBLA) were investigated. Successful formation of different PEG-b-PBLA block copolymers was confirmed by nuclear magnetic resonance measurements. Gel permeation chromatography studies showed that PEG-b-PBLA block copolymers have narrow molecular weight distribution. Differential scanning calorimetric studies showed that the melting point of PEG block slightly increased when degree of polymerization of PEG was increased from 125 to 273 with almost fixed PBLA block length. FTIR measurements were used to explore the effect of phenol on the self-assembly behavior of PEG-b-PBLA and it was revealed that the presence of hydrogen bonding interaction between block copolymers and phenolic resin. The influence of phenolic resin on the surface morphologies of block copolymer thin films were explored by scanning electron microscopy.
Keywords Block copolymer; Self-assembly; Thermal annealing; Phenolic resin; Hydrogen bonding.
-
INTRODUCTION
Block copolymers are made up of two or more chemically different monomers linked together by covalent bonds. They are capable of producing nanostructures either in the solution state or in the thin films (melts) [1]. These block copolymer nanostructures can be used in different applications such as biomedical (drug delivery) [2] templates to prepare porous materials for energy storage applications [3] and water permeable membranes [4].
Synthesis and self-assembly behavior of poly(ethylene glycol)-block-poly(-benzyl L-aspartate) (PEG-b-PBLA) was extensively reported in the area of drug delivery related fields. For example, earlier studies on PEG-b-PBLA suggested that anionic block copolymers, PEG-b-polyaspartic acid (PAsp) can be prepared after hydrolyzing PEG-b-PBLA [5]. Then nanometer sized polyion complex micelles could be obtained via electrostatic interaction between these anionic block copolymers and suitable cationic polymers and these micelles serve as the drug delivery systems. Very recently, we have also explored the micellization behaviours of these block copolymers in the mixed solvents such as DMSO and distilled water [6]. Akashi et. al. investigated the relationship among the organizational performance, the thermal and mechanical properties of PBLA-b-PEG-b-PBLA triblock copolymer thin films [7]. It was found that the mechanical properties of the thin films are governed by thermal treatment. Floudas and
colleagues reported the self-assembly behavior of poly(- benzyl-L-glutamate)-b- PEG -b-poly(-benzyl-L-glutamate) (PBLG- b-PEG-b-PBLG) triblock copolymer melts [8]. They found that the self-assembly behavior was decided by different interactions such as crystallization, hydrogen bonding, liquid crystallinity and microphase separation. They have also identified that the peptide volume fraction was also another vital factor that controlling the nanostructures of the block copolymers.
The ordered and disordered nanostructures can be fabricated from the self-assembly of amphiphilic block copolymers by selectively incorporating with thermosets [9]. The ordered mesoporous phenolic resins or carbon materials are found to have improved mechanical stability, higher surface areas and large pore volumes. Inspired by these interesting properties, they can be used in different applications, namely, photonics, adsorption, catalysis and separation. As a consequence, investigating the formation of nanostructures from the blends of block copolymers and phenolic resins has attracted significant interest. For instance, Ikkala and Ruokolainen and co-workers utilized poly(isoprene-b-2-vinyl pyridine) (PI-b-P2VP) and poly(styrene-b-4-vinyl pyridine) (PS-b-P4VP) diblock copolymers as templates for the fabrication of mesoporous phenolic resins using hexamethylenetetramine (HMTA) as the curing agent [10]. Further, Zheng and colleagues developed the thermosets with phenolic nanostructures upon curing novolac and poly(styrene-b-ethyleneoxide) (PS-b-PEO) diblock copolymer along with HMTA [11]. All the above mentioned reports revealed that except PS or PI, other polymers were miscible with phenolic resin and stabilized through hydrogen bonding. Earlier report on the interaction between PEG-b-PCL and phenolic resin showed that the addition of phenolic resin with PEG-b-PCL, induced the formation of ordered gyroid nanostructures [12]. It was also observed that disordered structures were formed in the absence of phenolic resin.
As far as we are aware that none of the above mentioned reports related to the preparation of the ordered phenolic resin based thermostat from the PEG- b- PBLA block copolymers. In this scenario, it is of interest to study the effects of phenolic resin on the self-assembly behavior of PEG- b-PBLA thin films in the present work.
-
EXPERIMENTAL
-
Synthesis and characterization of PEG-b-PBLA diblock copolymers
Synthesis of PEG-b-PBLA diblock copolymers were carried out using the ring-opening polymerization of -Benzyl L-aspartate N-carboxy anhydride (BLA-NCA) using – methoxy–amino polyethylene glycol (CH3O-PEG-NH2) as a macroinitiator. Block copolymers were purified by precipitation of the final product using diethyl ether and then dried under vacuum for overnight. Degrees of polymerization and molecular weight distribution were measured respectively by NMR and GPC experiments.
-
Preparation of thin films
The thin films were prepared, from PEG-b-PBLA along with four different wt% of phenolic resin containing 0, 25, 50 and 70 wt% phenol, by solution casting method. The polymer concentration used was 30 mg/ml. The glass substrates of area of 1 cm2 were used for the preparation of thin films. The solutions containing above mentioned combinations were poured over the different glass substrates and subjected to dry at room temperature. After overnight drying, the thin films were annealed at 100°C for 24 h in vacuum furnace.
-
Characterization of thin films
Thermal properties of block copolymers (PEG-b-PBLA) were studied by differential scanning calorimetry (NETZSCH DSC 214 Polyma) measurements from -30 °C to 400 °C with the increment of 5 °C/min. KBr pellet method was adapted to record FTIR spectra of PEG-b-PBLA/phenolic resin thin films were recorded on a Perkin-Elmer Spectrum 2 model FTIR spectrophotometer. Scanning electron microscope (Carl-Zeiss, EVO-18) was employed to observe the surface morphologies of PEG-b-PBLA/phenolic resin thin films.
-
-
RESULTS AND DISCUSSION
-
Characterization of block copolymers
(b) 4 6
3
1 2 5
Fig. 1. (a) Molecular structure of PEG-b-PBLA, (b) NMR spectrum of EG273-b-BLA73 in DMSO-d6.
In order to check whether the PEG-b-PBLA block copolymers have been formed or not, 1H NMR measurements were conducted on the block copolymers. Figure 1 shows the molecular structure of PEG-b-PBLA block copolymer and 1H NMR spectrum of EG273-b-BLA73. 1H NMR spectrum of another block copolymer (EG125-b-BLA80) is not shown in the figure 1 since its spectrum is almost same as other one. The spectrum confirmed that block copolymers were successfully prepared. The degrees of polymerization were determined from the NMR peak ratios and the values are listed in the Table .
GPC experiments were performed on the PEG-b- PBLA block copolymers to determine their molecular weight distribution. The GPC chromatograms of block copolymers are plotted as shown in the Figure 2. As expected, it is noticed from these chromograms that block copolymers with shorter chain length was eluted slower than that of longer chain block copolymers. This study also showed that the
6
-
6
4 3
5
2 1
block copolymers synthesized in this study are having narrow molecular weight distribution (Table 1).
Polymer
M a n
(g/mol)
PDIb
Degree of polymerization
Volume fraction
m
n
PEG
PBLA
EGm-b- BLAn-1
25,500
1.08
125
80
0.22
0.78
EGm-b- BLAn-2
30,200
1.13
273
73
0.397
0.603
Polymer
M a n
(g/mol)
PDIb
Degree of polymerization
Volume fraction
m
n
PEG
PBLA
EGm-b- BLAn-1
25,500
1.08
125
80
0.22
0.78
EGm-b- BLAn-2
30,200
1.13
273
73
0.397
0.603
Table 1. Molecular characteristics of the PEG-b-PBLA.
Fig. 2. GPC chromatograms of EG125-b-BLA80 and EG273-b-BLA73.
DSC measurements were conducted on the block copolymer powder samples to explore their thermal properties. DSC thermograms of block copolymers, PEG-b- PBLA are shown in the Figure 3. From this figure, it is clear that block copolymers having a sharp peak around 57 °C and two more broad peaks between 200 and 300 °C. The peak at 57 °C is attributed to the melting point of PEG crystals. The broad peaks between 200 and 300 °C might be due to the melting point of PBLA. This observation is similar to the earlier reports [7] and it was mentioned that the melting point of PBLA was found to above 250 °C. It was also found that these peaks shift slightly towards high temperature upon increasing the degrees of polymerization of PEG block from 125 to 273.
Fig. 3. DSC thermograms of EG125-b-BLA80 and EG273-b-BLA73.
-
-
Effects of phenolic resin on the self-assembly behavior of block copolymers
(d)
(c)
(b)
Fig. 4. Effect of phenolic resin on the FTIR spectra of EG273-b-BLA73. (a) 0 wt% phenol,(b) 25 wt% phenol, (c) 50 wt% phenol and (d) 70wt% phenol.
As per the theory and phase diagram of block copolymers, bicontinuous gyroid nanostructures can be fabricated from the block copolymers when the volume of fraction of one of its block is around 0.4 [13]. In this study, in the case of EG273-b-BLA73, volume fraction of PEG block is
-
whereas for the case of EG125-b-BLA80, volume fraction of PEG block is 0.22. Further our interest is to fabricate the gyroid nanostructure. Hence, we have chosen EG273-b-BLA73 rather than EG125-b-BLA80 to investigate the effects of phenolic resin on the self-assembly behaviours of EG273-b-BLA73.
(a)
(a)
1m
1m
(b)
(b)
2m
2m
nfluence of different concentration of phenolic resin on the s
nfluence of different concentration of phenolic resin on the s
(c)
(c)
(d)
(d)
2m
2m
1m
1m
Fig. 5. I urface
morphologies of EG273-b-BLA73. (a) 0 wt% phenol (b) 25 wt% phenol (c) 50 wt% phenol and (d) 70 wt % phenol.
It is known that FTIR spectroscopy is the appropriate technique for examining the secondary structures of the polypeptide blocks as well as interaction between the PEG-b-PBLA block copolymer and phenolic resin. In view of this, FTIR studies have been performed on the block copolymer/phenolic resin thin films and their spectra are plotted as shown in the Figure 4. From this figure it is obvious that the sharp FTIR peaks at 1657 cm-1 and 1554 cm- 1 are attributed respectively due to presence of amide I and amide II indicating the -helix conformations of the PBLA block in the untreated PEG- b-PBLA. Earlier report suggested that PBLA block showed similar conformations [7, 14]. Upon adding the phenolic resin, the peak at 1554 cm-1 gradually decreases with increase in concentration of phenolic resin.
FTIR peak at 1740 cm-1 indicate the presence of carbonyl group of PBLA in the case of untreated PEG-b PBLA. After treating the block copolymer, PEG-b-PBLA with phenolic resin, this peak shifts towards lower wave number at 1709 cm-1 for the 50 wt% phenolic resin treated PEG-b-PBLA. At the same time, 70 wt% phenolic resin treated PEG- b-PBLA showed two peaks at 1710 and 1738 cm-1.The peak around 1710 cm-1 arised because of hydrogen bonded carbonyl stretching whereas the peak at 1738 cm-1 is due to the presence of carbonyl functional group without hydrogen bonding. The similar observations were noticed for the case of the mixture of PEO-b-PCL and phenolic resin [15].
For phenolic resin treated PEG-b-PBLA, the peak was found at 1384 cm1 and assigned to the presence of the phenol O-H in plane bend. The intensity of this peak increases with increase in content of phenolic resin. In the case of untreated PEG-b-PBLA diblock copolymer, two strong peaks were found at 1015 and 1110 cm-1. These peaks imply that presence of ether group of the PEG segment and also sensitive to the H-bonding interaction. After blending phenolic resin with PEG-b-PBLA, intensity of peak at 1015 cm-1 decreased with increase in concentration of phenolic resin. This observation might be due to H-bonding of ether group of PEG with phenolic resin.
SEM observations were conducted on the block copolymer thin films to reveal the effect of phenolic resin and annealing on their surface morphologies. SEM images of thermally annealed PEG-b-PBLA /resol films on glass slide surfaces are shown in Figure 5. It was noticed phenolic resin dependent surface morphologies were observed. Micrometer sized particles were noticed.
-
-
CONCLUSIONS
Comprehensive study was conducted to explore the influence of phenolic resin on the self-assembly behaviors of the block copolymer, PEG-b-PBLA were investigated. Successful formation of PEG-b-PBLA was confirmed by 1H NMR measurements. Gel permeation chromatography studies showed that PEG-b-PBLA block copolymers have narrow molecular weight distribution. DSC studies revealed that melting peaks correspond to PEG and PBLA block in the block copolymer. DSC studies also showed that thermal properties of the block copolymers were found to vary slightly upon increasing the degree of polymerization of PEG block for fixed chain length of PBLA. FTIR studies revealed that addition of phenolic resin results in hydrogen bond inateraction between PEG-b-PBLA block copolymers and phenolic resin. SEM observations confirmed that micrometer sized particles were present in all the cases. Cross-sectional SEM observations are underway to explore the more details about the micrometer sized particles.
ACKNOWLEDGEMENTS
Authors gratefully acknowledge Department of Science & Technology – Science and Engineering Research Board under Early Career Research scheme (file no. ECR/2017/000760/PMS) for funding.
REFERENCES
-
H. Feng , X. Lu, W. Wang , N.G. Kang and J. W. Mays, Polymers 9, 494, (2017); doi:10.3390/polym9100494.
-
H. Cabral, Y. Matumoto, K. Mizuno, Q. Chen, M. Murakami, M. Kimura, Y. Terada, M. R. Kano, K. Miyazono, M. Uesaka N. Nishiyama and K. Kataoka, Nature Nanotechnology, 6, (2011).
-
J.G. Li, Y.F. Ho, M. M. M. Ahmed, H.C. Liang and S. W. Kuo,
Chem. Eur. J., 25, 1 9, (2019).
-
T. Yamamoto, T. Kimura, M. Komura, Y. Suzuki, T. Iyoda, S.Asaoka, and H. Nakanishi, Adv. Funct. Mater., 21, 918926 (2011).
-
A. Harada and K. Kataoka, Soft Matter, 4, 162167 (2008).
-
S. Padmavathi S, M. Murugan, M. Sivanantham, IOSR Journal of Applied Physics, 11, 40-43 (2019).
-
S. Tanaka, A. Ogura, T. Kaneko, Y. Murata, and M. Akashi,
Macromolecules, 37, 1370-1377 (2004).
-
G. Floudas, and P. Papadopoulos, H.-A. Klok, G. W. M. Vandermeulen, and J. R. Hernandez, Macromolecules 36, 3673-3683 (2003).
-
J. Guang Li, C. Y. Chung and S.W. Kuo, J. Mater. Chem., 22, 18583-18595 (2012).
-
H. Kosonen, J. Ruokolainen, P. Nyholm, and O. Ikkala,
Macromolecules, 34, 3046-3049 (2001).
-
D. Hu, Z. Xu, K. Zeng, and S. Zheng, Macromolecules, 43, 2960 2969 (2010).
-
W.C. Chu, Bishnu Prasad Bastakoti, Y. V. l. Kaneti, J.G. Li, H. R. Alamri, Z. A. Alothman, Y. Yamauchi, and S. W. Kuo, Chem. Eur. J., & 23, 1 9 (2017).
-
M. W. Matsen and M. Schick, Phys. Rev. Lett, 72, 2660 (1994).
-
C. T. Yang, Y. Wang, and Y. C. Chang, Biomacromolecules, 11, 13081313 (2010).
-
J. G. Li, Y. D. Lin, and S. W. Kuo, Macromolecules, 44, 92959309 (2011).