
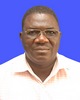
- Open Access
- Authors : Seydou Ouedraogo , Komlan Lolo , Kodjo Attipou , Ayite Senah Akoda Ajavon, Sonnou Tiem
- Paper ID : IJERTV9IS030301
- Volume & Issue : Volume 09, Issue 03 (March 2020)
- Published (First Online): 21-03-2020
- ISSN (Online) : 2278-0181
- Publisher Name : IJERT
- License:
This work is licensed under a Creative Commons Attribution 4.0 International License
Assessment of Wind Potential in the Perspective of Water Pumping in Sahelian Area of Burkina Faso
Seydou Ouedraogo*1, Komlan Lolo2, Kodjo Attipou2, Ayite Senah Akoda Ajavon3, Sonnou Tiem2
1 Laboratoire de Recherche en Sciences de lIngénieur (LARSI), Département de Génie Électrique, Institut Universitaire de
Technologie, Université Nazi BONI, 01 BP 1091 Bobo-Dioulasso 01, Bobo-Dioulasso, Burkina Faso.
2 Équipe de Recherche en Mécanique (ERM), Département de Génie Mécanique, École Nationale Supérieure dIngénieurs (ENSI), Université de Lomé, 01 BP 1515 Lomé 01, Lomé, Togo.
3 Laboratoire de Recherche en Sciences de lIngénieur (LARSI), Département de Génie Électrique, École Nationale dIngénieurs (ENSI), Université de Lomé, 01 BP 1515 Lomé 01, Lomé, Togo.
Abstract:- This paper focuses on the prospects for wind pumping installations in the Sahelian and Sudano-Sahelian zones of Burkina Faso. Three localities were chosen for the study namely, Dori, Ouahigouya and Ouagadougou. A statistical study allowed the determination of average wind speed. Wind speed average at the height of the tower on the three sites varies between 2.4 and 4.2 m/s. The availability of groundwater resources in the sahel, combined with the wind regime, makes it possible to determine the prospects of installing a pumping wind turbine at each site. Usable outout power of chosen wind turbine calculation made it possible to determine daily flows drawn off, using a mechanical pumping wind turbine and an aerogenerator of different nominal powers, depending on the total manometric height. The advantages and disadvantages of each pumping system are highlighted. The results show that Ouahigouyas and Ouagadougous sites are suitable for such installations while Doris site requires hybrid systems such as solar photovoltaic/wind or solar photovoltaic/generator.
Keywords: Livestock watering, Wind energy potential, Pumping wind turbine, Total manometric height, rate of flow
-
INTRODUCTION
In recent decades, marked by droughts in Sahelian countries, many pastoralists have relentlessly moved in search of water and pasture. In Burkina Faso, pastoralists and their livestock leave the Sahelian zone, considered to be the first breeding region of the country, and migrate to agricultural areas in the south, especially during the hottest months of the year [1]. The overcrowding of farm animals in the reception areas creates conflicts between farmers and herders. Furthermore, livestock occupies a strategic place in the economy of Burkina Faso. According to the Ministry of Animal Resources of Burkina Faso, livestock contributes 10% to the Gross Domestic Product and occupies the second place in value of exports after cotton. Animal husbandry is a source of income for more than 80% of the population [2]. Given the importance of the livestock sector in the national economy and social conflicts created by the migration of livestock to agricultural areas, there is an increasing urgency to address the issue of livestock water supply in the Sahel of Burkina Faso.
Field surveys show that the total demand for water for watering large herds of livestock in Burkina Faso Sahel is estimated at 72,000,000 m3 per year [3]. Watering livestock with surface water is the method most used by breeders [4]. Because of the significant potential evapotranspiration in the Sahel, surface waters of the last rainy season are quickly evaporated. In addition, Burkina Faso Sahel groundwater resources map shows that the chosen sites are characterized by the abundance of groundwater, with a tank roof ranging from 10 to 100 meters deep [5]. Several attempts have been made to make water available in the Sahel of Burkina Faso in dry season. "Christine" drilling, which pump is powered by a generator allows several hundred animals and people to access drinking water, precious and scarce during non-rainy periods [6]. However, this pumping system has experienced technical breakdowns resulting in its inoperability.
Solar, wind and biomass energy potentials in the Sahelian zone show that it is favorable to renewable energies systems. Solar energy is mainly used in photovoltaic pumping systems for supply drinking water and for small-scale irrigation [7]. Biomass and wind energy are not exploited.
Wind energy has experienced very strong growth over the past decade, thanks to technologies development and benefits for environment [8]. Among possibilities for harnessing wind energy, there is water dewatering. Technical choice for a type of wind pumping installation on landlocked sites and distant, mainly in Sahelian and Sudano-Sahelian zones, depends on parameters such as site wind potential, water need, water point nature and distance from wind turbine to place where water should be brought [9], [10].
The main objective of this article is feasibility study of wind pumping system installation on three sites in Sahelian zone of Burkina Faso, allowing to permanently supply water to breeders and their herds.
-
MATERIAL AND METHOD
For wind regime and the pumping rates study, year is divided into three periods depending on climatic specificities: dry and hot period, from March to June, rainy period from July to October and harmattan period from November to February. Study is conducted for four months, March, April, May and June, representing dry and hot periods, during which water need is highest and surface water is completely evaporated.
Wind potential assessment
For wind potential assessment, it is necessary to express wind speed frequency distribution on the site [11]. Weibull distribution is the most widely used and recommended in literature for expressing wind speed frequency distribution [12]. In Weibull distribution, wind speed variations are characterized by two functions: probability density function and cumulative distribution function [13]. Probability density function f(v) indicates time fraction (or probability) for which wind have the speed v. It is given by equation (1).
k v k 1
v k
f v
c c
exp c
(1)
where: c is scale factor (m/s), k is form factor (without unit), v is wind speed.
Cumulative distribution function gives time fraction (or probability) for which wind speed is less than or equal to speed v . Corresponding cumulative frequency is given by equation (2).
v
v k
F v f v dv 1 exp c
(2)
0
Weibull parameters estimation
There are several methods for estimating Weibull law parameters from raw wind speed data during a given period. But, power density method is the most accurate method for windy sites like those in this study [14]. Energy pattern factor EPf is defined by equation (3).
3 1 n 1 n 3
Epf
V 3 V
v3
i
i
n n
vi
(3)
i1 i1
where: V is the average wind speed, n intervals number.
Parameter k value is determined using according to equation (4).
E
E
pf
pf
k 1 3,69 2
(4)
Then c is determined with equation (5).
c V
11 k
(5)
where: is the gamma function defined by equation (6).
v t z1et 1dt
0
(6)
Average wind speed
According to Weibull distribution, average wind speed V is calculated with equation (7).
V vf vdv [m/s] (7)
0
where: v is wind speed rated, f (v) occurrence frequency.
Wind rse
A wind rose allows to know, for a given point, wind frequencies observation, according to direction and force criteria [15]. Wind direction is wind origin. Winds origin is determined according to following directions: between 0° and 90°, winds come from north-east (N-E) ; between 90° and 180°, winds come from Southeast (S-E) ; between 180° and 270°, winds come from southwest (S-O) ; between 270° and 360°, winds come from northwest (N-O).
Wind speed vertical extrapolation
Wind speed data for this study are measured at 10 meters above ground. It is necessary to estimate Weibull parameters and wind speed at different altitudes [16]. Weibull parameters extrapolation is obtained by equations (8), (9) and (10).
k1 k0
1 0,00881lnz 10
(8)
n
n
c1 c0 z z0
(9)
n 0.37 0.0088 ln c0
(10)
1 0.0088 ln z
10
where: k0 is shape parameter at measurements height; k1 shape parameter at wind turbine hub height; c0 scale parameter at measurements height; c1 scale parameter at wind turbine hub height; z0 measured height (10 m); z wind turbine hub height.
Wind speed extrapolation for different altitudes is obtained with equations (11) and (12) [16].
v v0 z z0
aJ
(11)
aJ 1 ln zg
r0
g
g
z
z0 z
(12)
where: v is estimated velocity at height z; v0 wind speed at measured height; z wind turbine hub height; z0 measured height; aJ empirical factor expressing surface roughness influences and atmospheric stability; zg geometric mean height and r0 soil roughness.
In present study, wind speed measurements are carried out in airports and ground roughness is rural type: r0 = 0,07 m [17].
Wind available power density
Wind power density represents the amount of energy produced by wind. Power density available in wind (Pav), per area unit swept by wind turbine blades is given by expression (13) [18].
Pav
1
2
v3 f vdv [W/m2] (13)
0
where is air density (kg/m3), v is wind speed,
f v probability density function.
Wind recoverable power density
According to Betz limit, the maximum wind recoverable power density (Prec) on a site becomes equal to 16/27 of available power density. Equation (14) translates this relation [18].
P 16 P
[W/m2] (14)rec 27 dispo
Wind recoverable energy, per area unit and for one year, according to Betz limit is expressed by equation (15).
Erec 8,76Prec [kWh/m2.an] (15)
Wind turbine average power supplied
Power provided by a wind turbine (Pu) is given by equations (16) and (17) [18].
0 pour
v Vstart
a bvk
pour Vstart vVrat
P pour V
vV
(16)
n
0
pour
rat
v Vstop
stop
with:
PV k P
V
V
a n start et b n
(17)
V
V
V
V
V
V
k start
k rat
k rat
k stat
where: Vstart is starting speed, Vrat is rated speed, Vstop is machine stop speed and Pn is nominal power.
Electrical output power average (Pel) is given by expressions (18) to (20).
Pel Pu f v, k, cdv
0
Vrat
(18)
Pel a bv f v, k, cdv
k
Vstart
Vstop
(19)
-
Pn f v, k, cdv
Vrat
Pel Pn FC
(20)
Where: Vstart is starting speed, Vrat is rated speed, Vstop is machine stop speed and Pn is the nominal power.
FC is factor form given by equation (21).
Vstart k Vrat k
k
k
e c
-
e c
Vstop
(21)
FC
V
k V
k
c
start rat
c c
Usable wind power
Useful power average is related to electrical output power Pel by a ratio expressing transformation efficiency. Considering aerogenerator efficiency , usable wind power average Pu is given by equation (22).
Pu Pel
(22)
Wind turbine efficiency is given by equations (23) and (24).
Pn Ptheo
(23)
with :
PTheo
1
2
air
3
SV
SV
rat
(24)
where: Pheo is aerogenerator theorical power; Pn aerogenerator nominal power; air air density; S wind turbine blades surface.
Pumped flow estimation
Two pumping systems are discussed in this study namely: wind turbine mechanical pumping and wind turbine electric pumping.
Mechanical pumping
Considering a mechanical pumping system of overall efficiency and total head of height Hmt, daily flow Qd is given by equation (25).
Qd 3600 24
Pmeca
water gHmt
(25)
Where: Hmt is total manometric height; g gravity acceleration; water water density; pumping system overall efficiency; and
Pmeca mechanical power provided by wind.
Electric pumping
Considering an electric pumping system of overall efficiency and total manometric height Hmt, daily pumped water flow Qj is given by equation (26) [19].
Qj 3600 24
Pel
water gH mt
(26)
Hmt is total manometric height; g gravity acceleration; water water density; pumping system overall efficiency and Pel electrical power.
Studied sites choice
Feasibility study covers Dori site in Northeast, Ouahigouya site in northwest and Ouagadougou site in central Burkina Faso. All sites are located in Sahelian zone of Burkina Faso, whose hot and dry climate from March to June, rainy from July to October and cold and dry from November to February is characterized by long periods of drought. In Sahel, rainfall is erratic, lasts only about 3 months and average annual rainfall is between 300 and 600 mm. Rains poor distribution in this area has resulted in increased water requirements for livestock. Table 1 gives sites geographic coordinates.
Table 1. Studied sites geographical coordinates
Country
Site
Longitude
Latitude
Altitude
Burkina Faso
Dori
0°03 West
14°03 North
282 m
Ouagadougou
1°32 West
12°21 North
299 m
Ouahigouya
2°25 West
13° 35 North
339 m
Data used in this study are provided by Burkina Faso National Directorate of Meteorology [20]. These are results of tri-hour observations of wind force (FF_XX) and direction (DD_XX), from 0 to 24 hours and 10 meters above ground level, from the weather station of three sites (Table 1). They correspond to a collection period of 10 years, from 2004 to 2013. Data recordings are made daily, 3 hours apart, for a total of 29,224 measurements collected over ten (10) years (2004 – 2013) for each site.
Pumping machines choice
Wind energy exploitation principle in water pumping is based on kinetic energy transformation contains into mechanical energy, once in contact with wind machine blades.
For electrical pumping, mechanical energy is converted into electrical energy by alternator which supplies motor pump system installed in well. Used pump is usually centrifugal pump with asynchronous motor, which operates at variable power and speed [21].
For mechanical wind pumping, mechanical energy activates piston pump via connecting rod, mounted on rotor shaft which converts rotary motion into reciprocating rectilinear motion which controls the piston pump installed in well [22]. Starting speeds of mechanical wind turbines are order of 2.5 to 3 m/s. Converter-pump efficiency is order of 60% [23]. These wind turbines re generally equipped with propeller and whose diameters can reach 9 meters. However, because of mechanical stress, stem can not exceed heights of 20 meters. This type of installation is reserved for wells whose depth is less than 15 meters [23].
-
-
RESULTS AND DISCUSSIONS
Figure 1 shows wind speed measured distribution at Dori, Ouahigouya and Ouagadougou sites.
Distribution of wind speed-Dori site
March April
0.3 May
Jun
0.25
Frequency
Frequency
0.2
0.15
0.1
0.05
0
1 2 3 4 5 6 7 8 9 10
Wind speed [m/s]
0.25
0.2
Frequency
Frequency
0.15
0.1
0.05
0
Wind speed distribution – Ouahigouya site
March April May
Jun
1 2 3 4 5 6 7 8 9 10 11 12 13 14 15 16 17 18 19 20
Wind speed [m/s]
Wind speed distribution – Ouagadougou site
March
0.2 April
0.18
0.16
May Jun
0.14
Frequency
Frequency
0.12
0.1
0.08
0.06
0.04
0.02
0
1 2 3 4 5 6 7 8 9 10 11 12 13 14
Wind speed [m/s]
Figure 1. Wind speed dsitibution at the three sites.
Observation in figure 1 is that calm winds (speed less than 1 m/s) are frequent on three sites. Calm winds frequency is 0.35 for Dori site, 0.20 for Ouahigouya site and 0.15 for Ouagadougou site. Considering the frequency of calm winds, Ouagadougou site has the best wind distribution among three studied sites. Weibull's distribution shows that studied sites are not enough windy. Table 2 shows Weibull shape and scale parameters monthly variation, estimated by power density method, for studied sites.
Table 2. Estimated shape and scale factors monthly by power density method
Site
Dori
Ouagadougou
Ouahigouya
Month
k [-]
c [m/s]
k [-]
c [m/s]
k [-]
c [m/s]
March
1.0380
1.8432
1.8031
3.9067
1.6922
3.2770
April
1.1933
2.3711
1.8932
4.0377
1.3981
3.9846
May
1.2369
2.5159
1.7343
3.8825
1.7410
4.4263
Jun
1.3024
2.5615
1.6798
3.9115
1.3323
5.0467
Observation is that shape parameter k varies between 1.038 in Dori (in March) and 1.8932 in Ouagadougou (in April). So, wind speed is more uniform in Ouagadougou during April month, while it is less uniform in Dori during March month. Scale parameter c varies between 1.8432 m/s in Dori (in March) and 5.0467 m/s in Ouahigouya (in June), which shows that Ouahigouya site is windiest for this period. Monthly wind speeds average at 10 m height at three sites are shown in figure 2.
Dori site Ouagadougou site Ouahigouya site
Dori site Ouagadougou site Ouahigouya site
9
8
Average wind speed [m/s]
Average wind speed [m/s]
7
6
5
4
3
2
1
0
0 20 40 60 80 100 120
Height [m]
Figure 2. Monthly wind speed average at 10 m height at three sites.
Wind speed has 4.63 m/s maximum value in Ouahigouya site (in June), while minimum wind speed of 1.26 m/s is recorded in November in Dori site. For Dori site, monthly wind speed averages are below 2.5 m/s. Ouagadougou site presents practically constant monthly speed averages, around 3.5 m/s. At Ouahigouya site, monthly speeds averages vary from 2.20 m/s in November to 4.63 m/s in June. Studied sites are not sufficiently windy.
Wind roses
Wind roses at Dori, Ouahigouya and Ouagadougou sites are shown in figure 3.
N
330
300
0 150
30
100
60
50
300
330
N
0 800
30
600
400 60
200
O 270 90 E
O 270 90 E
240 120
240
120
210
180
S
150
210
180
S
150
-
b)
N 0
330
600
30
400
300 60
200
O 270 90 E
240 120
210
180
S
c)
150
Figure 3. Wind roses at three studies sites: a) at Dori site, b) at Ouahigouya site, c) at Ouagadougou site
Wind statistics observation and analysis show predominance in direction: Northeast, with an inclination to North of geometric angle in third sector (between 60° and 90°) for Dori's site; Southeast, with an inclination to North of geometric angle varying between fifth and sixth sector (between 120° and 180°) for Ouagadougou's site; South West, with an inclination to North of geometric angle being in ninth sector (between 240° and 270°) for Ouahigouya's site. Annual speed averages are evaluated at 10 m to 130 m heights in 10 m steps, with soil roughness r0 = 0.07 m. Figure 4 shows extrapolated wind speeds.
Dori site Ouagadougou site Ouahigouya site
Dori site Ouagadougou site Ouahigouya site
9
8
Average wind speed [m/s]
Average wind speed [m/s]
7
6
5
4
3
2
1
0
0 20 40 60 80 100 120
Height [m]
Figure 4. Annual average wind speed at different height at three sites.
We notice that at 60 m height, minimum annual average wind speed of 3.29 m/s is recorded at Dori, while average annual maximum speed of 5.54 m/s is recorded in Ouagadougou. Annual speed average in Ouahigouya is 5.25 m/s. At 130 m height, wind speed annual minimum average of 4.20 m/s is recorded at Dori, while speed annual maximum average of 6.76 m/s is recorded in Ouagadougou. Wind speed annual average in Ouahigouya is 6.39 m/s. From 60 m height, Ouagadougou and Ouahigouya sites have wind speed average higher than 5 m/s, which is sufficient for interesting electricity production with wind turbines. But for Dori site, it is necessary to go beyond 130 m height to obtain wind speeds greater than 5 m/s. Figure 5 shows recoverable power density at three studied sites.
120
100
Recoverable power density
Dori site Ouahigouya site Ouagadougou site
80
Power[kW/m 2]
Power[kW/m 2]
60
40
20
0
Jan Feb Mar Apr May Jun Jul Aug Sep Oct Nov Dec
Month
Figure 5. Recoverable wind power density at three studies sites
Three commercial wind turbines of nominal power equal to 3.5, 10 and 35 kW were chosen for the simulation of electric pumping. Figure 6 represents useful and usable power for 10 kW wind turbine, respectively at Dori, Ouahigouya and Ouagadougou sites.
0.25
Power [kW]
Power [kW]
0.2
0.15
0.1
0.05
0
Wind turbine power – Dori site
10 kW wind turbine useful output power 10 kW wind turbine usable output power
Jan Feb Mar Apr May Jun Jul Aug Sep Oct Nov Dec Month
1.5
1.4
1.3
1.2
1.1
1
Power [kW]
Power [kW]
0.9
0.8
0.7
0.6
0.5
0.4
0.3
0.2
0.1
0
Wind turbine output power – Ouahigouya site
10 kW wind turbine useful power 10 kW wind turbine usable power
Jan Feb Mar Apr May Jun Jul Aug Sep Oct Nov Dec Month
1.5
1.4
1.3
1.2
1.1
1
Power [kW]
Power [kW]
0.9
0.8
0.7
0.6
0.5
0.4
0.3
0.2
0.1
0
Wind turbine output power – Ouahigouya site
10 kW wind turbine useful power 10 kW wind turbine usable power
Jan Feb Mar Apr May Jun Jul Aug Sep Oct Nov Dec Month
Figure 6. Useful and usable power of wind turbine at three studies sites
Water flow rates pumped by aerogenerator vary according to three sites and periods of year. Factors influencing water flow are highlighted in results summarized in tables 3 to 5, respectively for Dori, Ouahigouya and Ouagadougou sites.
Table 3. Pumping rates variation according to influencing factors at Dori site
Total manometric height Hmt (m)
Wind turbine tower height
H(m)
Daily water volume pumped (m3)
Dori site
March
April
May
Jun
20
20
0.0175
0.0226
0.0260
0.0225
25
0.0225
0.0275
0.0325
0.0274
30
0.0255
0.0350
0.0375
0.0350
30
20
0.0124
0.0170
0.0175
0.0160
25
0.0150
0.0200
0.0225
0.0195
30
0.0175
0.0226
0.0250
0.0226
Table 4. Pumping rates variation according to influencing factors at Ouahigouya site
Total manometric height Hmt (m)
Wind turbine tower height
H(m)
Daily water volume pumped (m3)
Ouahigouya site
Mars
Avril
Mai
Juin
20
20
0.05
0.09
1.45
2.70
25
0.05
0.09
1.70
3.00
30
0.05
0.10
2.00
3.20
30
20
0.02
0.05
0.30
0.55
25
0.025
0.05
0.35
0.60
30
0.025
0.075
0.4
0.65
Tableau 5 Pumping rates variation according to influencing factors at Ouagadougou site
Total manometric height Hmt (m)
Wind turbine tower height
H(m)
Daily water volume pumped (m3)
Ouagadougou site
Mars
Avril
Mai
Juin
20
20
0.050
0.050
0.96
1.05
25
0.050
0.050
1.110
1.275
30
0.090
0.090
1.375
1.500
30
20
0.028
0.028
0.190
0.200
25
0.030
0.030
0.230
0.250
30
0.070
0.070
0.275
0.300
Tables 3, 4 and 5 results snalysis shows site influence. We notice that for same period, same total manometric height and wind turbine tower height, daily flows obtained at Dori site for June month (0.0225 m3) are low, compared to those obtained at Ouahigouya and Ouagadougou sites which are respectively 2.70 m3 and 1.05 m3. This is explained by low wind potential at first site.
We note that, for Ouahigouya and Ouagadougou sites, water volumes pumped in June, with different total manometric heights and various wind tower heights are always high compared to those obtained in other months. This is explained by daily wind speeds average levels observed during June month of each year on these sites. For Dori site, May is month with the highest water pumped volumes compared to those obtained in other months.
Total manometric heights increase negatively affects water volumes pumped, whatever site and wind turbine height. Figure 7 illustrates well pumped flows decrease curves, according to total manometric height, corresponding to hottest periods of year, from March to June at three sites.
pumped water flow in electric mode – Dori site
0.04
March
3.5
Pumped water flow in electric mode – Ouahigouya site
March April
May
Jun
March April
May
Jun
0.035 April 3
May
0.03
Daily flow [m3/d]
Daily flow [m3/d]
0.025
0.02
0.015
0.01
0.005
0
Jun
20 30 40 50 60 70 80 90 100
Total manometric height [m]
2.5
Daily flpw [m3/d]
Daily flpw [m3/d]
2
1.5
1
0.5
0
20 30 40 50 60 70 80 90 100
Total manometric height [m]
5
4.5
pumped water flow in electric mode – Ouagadougou site
March April May
4 Jun
3.5
Daily flow [m3/d]
Daily flow [m3/d]
3
2.5
2
1.5
1
0.5
0
20 30 40 50 60 70 80 90 100
Total manometric heigth [m]
Figure 7. Pumped water flow in electric mode at three sites.
As an indication, for Ouahigouya site, in June, daily flow which was 2.70 m3, for 20 m total manometric height, drops to 0.55 m3, when manometric height increases by 10 m.
Generally, wind turbines have various heights of pylon. For selected wind turbine for this study, it can be mounted at three different pylon heights: 20 m, 25 m or 30 m. Pylon height influence is highlighted by tables 3, 4 and 5 results. By setting total manometric height at three sites, we see, that increasing wind turbine pylon height translates into increase in water flows pumped. For example, for Ouagadougou site, with 20 m total manometric height and 20 m elevation of machine propeller, flow is 0.96 m3 in May, whereas it increases to 1.315 m3, when height becomes 30 m; or 27% gain in flow for 10 m difference in height.
Mechanical pumping simulation is done with machines of similar power to wind turbines used in electric mode. Simulation results with a 10 kW power wind turbine, during hot season, from March to June are shown in figures 8.
600
500
Pumped water flow in mechanical mode – Dori site
March April May Jun
3200
March April
May
June
March April
May
June
3100
2600
Pumped water flow in mechanical mode – Ouahigouya site
Daily flow [m3]
Daily flow [m3]
400
300
200
100
2100
Daily flow [m3]
Daily flow [m3]
1600
1100
600
20
30
40
50 60 70
80
90
100
100
10
20
30
40 50 60 70 80
90
100
Total manometric height [m]
Total manométric height [m]
Pumped water flow in mechanical mode – Ouagadougou site
1000
900
800
March April MAy Jun
Daily flow [m3/d]
Daily flow [m3/d]
700
600
500
400
300
200
100
20 30 40 50 60 70 80 90 100
Total manometric height [m]
Figure 8. Pumped water flow in mechanical mode at three sites
Different curves examination shows that pumped flows are more important in mechanical mode than by electric mode. But well depth constraints of less than 35 m, penalize the mechanical pumping mode. Pumped flows with large wind turbines are more important and offer great possibilities. However, the wind regimes on studied sites mean that, to obtain pumped water flows comparable to those of mechanical mode, it would require large wind turbines, for tower heights up to 130 m.
-
-
CONCLUSION
Wind energy potentials study on installation sites went through wind data statistical processing, which allowed available wind potential assessment. Really usable wind potential was used to estimate daily flow rates pumped for several total manometric height. For electric pumping, daily flow average estimation, according to total manometric height pumping was carried out considering three commercial wind turbines characteristics, supposedly installed on three Sahelian sites, namely, Dori, Ouahigouya and Ouagadougou. Results showed that, across all three sites studied, water flows pumped in electric mode are low, compared to livestock water requirement. Same study was made considering mechanical pumping mode and taking into account limits of system. It has been observed that pumped water flows are greater in mechanical mode than in electric mode.
Ouahigouya and Ouagadougou sites can well house wind pumping installations. However, for Dori site, wind pumping is not efficient. For Dori site, we recommand use of other renewable energies in combination with wind energy, such as: photovoltaic solar energy and biogas produced from livestock waste, used as gaseous fuel in generators. Similarity of studied problem in several areas of Burkina Faso, require that solution approach developed, be applied to all farming areas of Burkina Faso and in Africa Sahelian area.
-
ACKNOWLEDGEMENTS
The authors want to thank the University Nazi BONI and the University of Lomé for providing enabling environment during the research.
REFERENCES
-
Ministère de lenvironnement et de leau, "État des lieux des ressources en eau du Burkina Faso et de leur cadre de gestion", Ouagadougou, Burkina Faso, 2001.
-
Ministère des Ressources Animales, "Plan dactions et programme dinvestissements du secteur de lélevage au Burkina Faso", Ouagadougou, Burkina Faso, 2000.
-
DREA Direction régionale de lEau et de lAssainissement du Sahel, "La problématique de leau souterraine dans le sahel burkinabè", Ouagadougou, Burkina Faso, 2019.
-
L. Ouedraogo, B. Ouedraogo, O. Kaboré, P. I. Yanogo, T. P. Zoungrana et I. B. MOUSSA, "Localisation des zones d'accès à l'eau en saison sèche par analyse multicritère dans le bassin versant du Goudébo, Yakouta, Burkina Faso", in Revue Physio-Géo, vol. 7(2013), pp. 49-66, 2013.
-
N-D. Yameogo, N. Kibi et T. Thiombiano, "Analyse socio-économétrique de la demande deau et de pâturage pour le bétail : cas du département de Loumbila au Burkina Faso", Rapport, Université de Ouagadougou, Burkina Faso, 2002.
-
K. A. Karama, " Elevage dans le Sahel : Christine ou le symbole de lhydraulique pastorale", éditions Sidwaya, Mai 2009 .
-
C. Pezon, Fabrice Agognon, Richard Bassono, "Coût, performance et régulation des petits réseaux de distribution deau potable au Burkina Faso Étude de six AEPS dans la région du Sahel", hal-02012147, 2013.
-
R. Maouedj, S. Bousalem et B. Benyoucef, "Optimisation dun système de pompage éolien : Application aux sites sahariens", in Revue des Energies Renouvelables, vol. 11, no 2, p. 239 250, 2008.
-
S. Ouedraogo, A. S. A. Ajavon, A. A. Salami, M. K. Kodjo, and K-S. Bédja, "Evaluation of wind potential in the sahelian area: case of three sites in Burkina Faso", in Research Journal of Engineering Sciences vol. 6, Issue 11, p. 43-53, 2017.
-
B. Bambara, "Atlas des énergies renouvelables du Burkina Faso", Mémoire de master, Institut International dIngénierie, Ouagadougou – Burkina Faso, 27 Octobre 2015.
-
A. Mostafaeipour, M. Jadidi, K. Mohammadi and A. Sedaghat, "An Analysis of Wind Energy Potential and Economic Evaluation in Zahedan, Iran", in Renewable and Sustainable Energy Reviews, Vol. 30, p. 641-650, 2014.
-
B. Safari, "Modeling wind speed and wind power distributions in Rwanda", in Renewable and Sustainable Energy Reviews, vol. 15, no 2, p. 925- 935, 2011.
-
D. K. Kidmo, R. Danwe, S. Y. Doka and N. Djongyang, "Statistical analysis of wind speed distribution based on six Weibull Methods for wind power evaluation in Garoua, Cameroon", in Revue des Energies Renouvelables, vol. 18, no 1, p. 105-125, 2015.
-
M. A. Houekpoheha, B. Kounouhewa, B. N. Tokpohozin and N. Awanou, "Estimation de la puissance énergétique éolienne à partir de la distribution de Weibull sur la côte béninoise à Cotonou dans le Golfe de Guinée", in Revue des Energies Renouvelables, vol. 17, no 3, p. 489-495, 2014.
-
M. Abdraman, A. Tahir, D. Lissouck, M. Kazet and R. Mouangue, "Wind Resource Assessment in the City of N'djamena in Chad", in International Journal of Renewable Energy Research (IJRER), vol. 6, no 3, p. 1022-1036, 2016.
-
Drissa BORO, Willy Magloire NKOUNGA, Mouhamadou Falilou NDIAYE, MamadouLamine NDIAYE, Florent P. KIENO & Joseph D. BATHIEBO, "Statistical analysis of wind data and assessment of wind potential in BurkinaFaso", IJESRT, vol. 8, Issue 2, February 2019.
-
S. Ouedraogo, A. S. A. Ajavon, A. A. Salami, M. K. Kodjo, "Optimality sizing of hybrid electrical power plant composed of photovoltaic generator, wind generator and biogas generator", in Research Journal of Engineering Sciences, vol. 7, Issue 11, p. 20-53, 2018.
-
G. Al Zohbi, P. Hendrick et P. Bouillard, "Evaluation du potentiel dénergie éolienne au Liban", in Revue des énergies renouvelables, vol. 17, no 1, p. 83 96, 2014.
-
N. K. Merzouk, M. Merzouk et B. Benyoucef, "Perspectives du pompage éolien dans le sud algerien", Algerian Scientific Journal Plateform, vol. 1, no 17, p. 27-37, 2015.
-
Direction Générale de la Météorologie du Burkina Faso, "Données climatologiques de stations météorologiques (20042013)", Standards and Reports, Ouagadougou, Burkina Faso, 2015.
-
A. Adjiri, H. Daaou Nedjari et S. Haddouche, "Estimation du potentiel éolien pour la région dIn Salah", in Revue des Energies Renouvelables, vol. 16, no 4, p. 657 666, 2013.
-
S. Baillargeon, "Les-éoliennes-de-pompage", Récupéré de http://www.economiesolidaire.com/les-eoliennes-de-pompage, 2019.
-
M. Benabdelkader, A. Malek et B. Draoui, "Perspective du pompage éolien appliqué à lirrigation du palmier dattier dans la région de Béchar", in Revue des Energies Renouvelables, vol. 14, no 3, p. 381 395, 2011.