
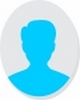
- Open Access
- Authors : Belay Abakuma Getachew , Tie-Gang Wang
- Paper ID : IJERTV9IS060088
- Volume & Issue : Volume 09, Issue 06 (June 2020)
- Published (First Online): 09-06-2020
- ISSN (Online) : 2278-0181
- Publisher Name : IJERT
- License:
This work is licensed under a Creative Commons Attribution 4.0 International License
Effect of Modulation Period on the Structure and Properties of AlTiN/AlTiSiN Multilayers
Belay Abakuma Getachew1 Professor Tie-Gang Wang2*
-
Tianjin University of Technology and Education, Tianjin 300222 China,
-
Tianjin key laboratory of high-speed cutting and precision manufacturing, Tianjin University of Technology and Education,
Tianjin 300222 China
AbstractThe AlTiN/AlTiSiN multilayer composite coating was deposited on cemented carbide by arc ion plating. The influence of different modulation periods on the structure and mechanical properties of AlTiN/AlTiSiN multilayer composite coating was studied systematically.
Scanning electron microscopy (SEM), electron probe microanalysis (EPMA), X-ray diffraction (XRD), nanoindentation and scratch tester were used to study the microstructure and properties of AlTiN/AlTiSiN multilayer coating. The friction coefficient and wear resistance of the coating was evaluated by Anton Paar. The results show that the multilayer coating is composed of FCC – (Ti, Al) n and HCP AlN crystals. The average friction coefficient of the coating decreases with the decrease of the modulation period. When the modulation period is 300 nm, the friction coefficient of the coating reaches 0.771. With the decrease of modulation period from 750 nm to 300 nm, the critical load of multilayer composite coating decreased from 99.3 n to 108.9 n. The higher the critical load, the stronger the adhesion of the coating to the substrate. When the modulation period is 300nm, the hardness of AlTiN/AlTiSiN multilayer composite coating is about 30gpa, and the wear rate is as low as 6.71 × 10-3 m3N-1m-1.
Keywords- Arc ion plating; AlTiN/AlTiSiN multilayered coatings; modulation period; microstructure; critical load.
-
INTRODUCTION
Cutting tools that are not coated could be used in the unserious working condition such as low cutting speed and free cutting materials. However, the demand to increase productivity by raising cutting speed and the occurrence of difficult to machine materials makes the service condition much tougher which has a significant impact on cutting life of the tool. [1]
At present, coated tools are widely used and most of the machine cutting tools used by manufacturers are deposited a layer of hard coatings on their surfaces. Hard coatings are generated worldwide by chemical vapor deposition (CVD) or physical vapor deposition (PVD). Chemical vapor deposition (CVD) or physical vapor deposition (PVD) is two of the most important technologies for hard coating preparation, which are widely used in the world. [2-3]. CVD coatings have the benefits of being able to deposit coating under the condition of low vacuum, simple tools, large suppleness, and good wrapping, etc., which is good for the coating of parts with composite form and deposition of an internal wall, internal hole, etc. [4-8]. PVD (physical vapor deposition) may be defined as the concentration of a flux of atoms or clusters of atoms in a vacuum. The flux of atoms may either be neutral or ionized. The deposition of the PVD coatings was carried
out by the use of the arc evaporation technology utilizing a conventional unit of arc evaporation [8]. The film arranged by PVD technology has the benefits of great hardness and strength, good thermal stability, good wear resistance, chemical stability, low friction coefficient, compact structure, and so on. [11-14]
A good way to increase the properties of the protective coating is to deposit a multilayer made of two different materials. Multilayer structure can effectively improve the mechanical and tribological properties of the coating because they have high hardness, corrosion resistance, wear- resistance, and adhesion properties, which can effectively improve the efficiency of industrial processing, and to a certain extent meet the needs of people for high-speed and high-precision. The TiN coating has been studied for a long time and its technology is mature. It can effectively improve the requirements of machining tools on hardness, chemical inertness, wear resistance, low friction, heat resistance, and oxidation resistance. However, its poor high-temperature oxidation performance limits its further application. To solve this problem, the Al element is doped into the coating to form the TiAlN coating. At high temperature, the Al element is easy to react with O element on the surface of the coating to form Al2O3 thin film to protect the internal coating and substrate, Besides the solid solution strengthening effect further improves the comprehensive performance of the coating [9]. Adding the Si element to the coating to further improve the coating performance [10]. This is because doping Si in TiAlN monolayer can effectively inhibit the growth of coating grains and increase the compactness of the coating. Si is solid soluble in (Ti, Al) N to further improve the mechanical properties of the coating. According to the Hall- Petch effect, the smaller the grains, the better the hardness of the coating [9].
In order to further improve the mechanical and tribological properties and expand the microstructure and properties of AlTiN / AlTiSiN coating by arc ion plating, a sequence of full studies on the surface morphology, hardness, surface section morphology and wear resistance of AlTiN / AlTiSiN coating were carried out and the effects of different modulation periods on the microstructure, mechanical properties and tribological properties of the coatings were systematically studied, to obtain the ideal wear resistance and high hardness of the film deposition parameters of AlTiN/AlTiSiN, and be more widely used in potential engineering fields.
-
RELATED WORK
In this paper [15] the effect of modulation period on the microstructure and properties of TiN/TiAlN multilayer coatings was investigated. The XRD analysis showed that a strong preferred orientation (111) plane in all TiN/TiAlN multilayer coatings. It gives the reason for this result that might be caused by the increasing inner strain with the coating growth. The energetic ion bombardment enhanced the atom migration rate and let them readily align along the densely packed (111) direction. On the other hand, at a modulation period of 164 nm, the nano hardness of TiN/TiAlN multilayer coatings reached the maximum value of 38.9 GPa. Compared to the TiN and TiAlN monolithic coatings, the TiN/TiAlN multilayered coatings exhibited higher hardness values between 28.9 and 38.9 GPa. This enhancement can be attributed to the nanoscale multilayer architecture. Because of the similar shear modulus of the individual layers of TiN and TiAlN, the coherent interface was formed by the epitaxial growth of TiAlN and TiN, there existed an alternating strain field in the multilayers due to lattice misfit, which could block the motion of dislocations and induce the strengthening of multilayers According to this paper the best adhesion strength of the TiN/TiAlN multilayer coatings was at modulation period of 54 nm.
In another study, The HfN/HfB2 multilayers with different modulation periods ranging from 20 to 150 nm were synthesized by magnetron sputtering. The results indicated that the multilayers at smaller modulation periods showed weak XRD textures, a low degree of crystallization, and a clear columnar microstructure. On the contrary, the high crystallization appears at a larger modulation period and the microstructure becomes more fine-grained due to the existence of B-rich components. [16]
In this paper [17] the authors investigated the influence of the modulation periods and ratios on the structure and mechanical properties of the iBN/CrN multilayers. The XRD analysis of the TiBN/CrN multilayer coatings indicated a strong preferred orientation (2 0 0) plane which could strengthen the hardness of coatings. At the modulation period of 11.7 nm and modulation ratio of 5:1, a maximum hardness of 38.6 ±
2.2 GPa and elastic modulus of 477 ± 20 GPa have been obtained. According to this experiment, the reason for is that this coating has a relatively sharp interface, which minimizes the intermixing of the two-component layers and hence exhibits higher hardness.
In this work [18], NbN/NbB2 multilayers are synthesized with different modulation ratios at the same modulation period of 10 nm, by using the magnetron sputtering system. The results showed that all multilayers show a clear multilayer structure in which the diffraction peaks corresponded to the typical cubic NbN structure and hexagonal NbB2 structure. Compared to tNbN:tNbB2 = 1:1, both the modulation ratio with the thicker NbB2 or thicker NbN can enhance the mechanical properties. According to this experiment, the preferential orientation occurs due to the lower surface atomic diffusivity on a particular crystallographic plane, which gives rise to faster growth of nanocrystallites in that orientation. The competition of the surface energy and strain energy affects NbN (111) and (200) textures in polycrystalline NbN layers. This improves the hardness to some extent. However,
the maximum hardness (36.86 GPa) and elastic modulus (412.31 GPA), highest critical load (87.93 mN) of NbN/NbB2 multilayers are obtained at tNbN:tNbB2=1:4. Additionally, in this experiment, all the residual stress of the multilayers coatings are lower than the monolithic NbN (2.067 GPa)and NbB2's (3.562 GPa). This is because when the NbN/NbB2 multilayer coating is deposited, the interfaces of NbN and NbB2 could act as barriers to prevent the motion of dislocation glide.
-
EXPERIMENTAL DETAILS
-
Preparation for the AlTiN/AlTiSiN coatings
The AlTiN/AlTiSiN multilayered coating was deposited on cemented carbide, monocrystalline silicon, and steel by changing the modulation period (double layer thickness) from 300 nm to 750 nm by arc ion plating system. The size of the cemented carbide sheet is 25mm × 25mm × 4mm. Before the deposition process, the substrate should be ground out by mp- 2d metallographic grinding machine on the chamfered corners of the cemented carbide, then by using a polishing cloth and diamond grinding paste, grind the surface of the cemented carbide to a smooth surface without scratches. The grinding paste and adhesive left on the substrate during the whole grinding process were removed by immersion in acetone. Then the samples were washed in deionized water and ethanol for 15 minutes. After then the samples were dried and mounted on a rotary sample holder in the chamber, which the rotation speed is kept at 40 rpm through the whole deposition process. The distance between the target and substrate was kept at approximately 285 mm. The diameter of the vacuum chamber is 800 mm and height is 1000 mm which was vertically equipped with one AlTi target and one AlTiSi target at alternative positions on the sidewalls of the chamber so that the rotary substrates could face each kind of cathodes alternatively. The holder was rotated to ensure the uniform growth of the films, to confirm the film thickness consistency in the vertical direction and to provide wide coating area so they are settled characteristically in the vertical direction. A large number of substrates can be coated consistently along the circumference while the table is kept rotating.
The chamber was Pumped until the vacuum degree of the cavity is up to 2.0×10-3pa, and it was heated to 430 when the vacuum degree was lower than 2.0×10-3pa, argon at a speed of 400sccm for sputtering cleaning was injected to the chamber, to remove the oxide and pollutant layer on the substrate. Then a bias voltage of – 800V was applied, and set the frequency to 10kHz, set the pulse width to 6 s, set the throttle valve to 0 °, and then conduct glow cleaning on the substrate for 20 minutes, to remove contaminants and improve adhesion between coating and substrate. adjust the substrate bias to -800V, -600V, -400V, -200V, and adjust the argon flow rate to 100sccm. Under different bias conditions, bombard and clean the substrate for 2 minutes respectively to remove other dirt on the surface of the substrate and improve the adhesion of the film/substrate.
To improve the bonding strength between the working layer and the matrix, TiN film was prepared as the transitional layer. Adjust the arc current of titanium target to 90A, pulse bias amplitude to 100V, frequency to 50KHz, pulse width to
8 s (duty cycle to 60%), argon injection rate to 50sccm, nitrogen flow rate to 200sccm as the reaction gas, deposition temperature to 430, time to 20 minutes.
At last, the AlTiN/AlTiSiN multilayered coating was deposited by the alternate of AlTi targets and AlTiSi targets. With the arc current was 80A target, the pulse width was reduced to 4 s (duty cycle 80%) at the frequency of 50KHz, the argon flow rate was set as 50sccm, and the nitrogen flow rate was 600sccm. During the deposition process, the temperature was kept at 430 and the pulse bias amplitude was kept at 100V. In this experiment, on the premise of knowing the deposition rate of AlTiN and AlTiSiN, the overall film thickness is controlled to 3 m, and 300/375/500/750nm is taken as the modulation period of AlTiN/AlTiSiN multilayered coating.
-
Characterization of AlTiN/AlTiSiN coatings
The surface morphology of the AlTiN/AlTiSiN coating deposited on the monocrystalline silicon substrate was studied by the FE-SEM system of 20keV. The surface morphology and cross-section morphology of AlTiN/AlTiSiN coatings were observed by Hitachi s-4800 field emission scanning electron microscope (SEM) and Oxford ISIS energy dispersive spectrometer (EDS).
X-ray diffraction (EPMA, EPMA-1610, Shimazu, Japan) was used to characterize the crystal structure and phase composition of AlTiN/AlTiSiN multilayered coating. The specific parameters of the experimental instrument are: the maximum current of the copper target X-ray tube is 80 Ma, the maximum voltage is 60 kV, the maximum power is 2.2 kW, the scanning speed is 0.02 °/s, the step length is 0.01 °, the wavelength is 0.154 nm, and the scanning range of the diffraction angle (2) is 20°~80°. According to the XRD results, the crystallinity of the coating can be calculated. The specific calculation formula is as follows.
Area of crystalline peaks
KLA-Tencor D300, and the wear rate is calculated according to the formula V = A/(n·F) (n is the number of friction rings, F is the normal load). The probe pressure was 2.0mg, scanning speed was 0.05mm/s; scanning range was 10 m, scanning distance was 1.2mm, and finally, the wear rate was obtained.
-
-
RESULTS
-
Phase analysis of AlTiN/AlTiSiN coating
As mentioned earlier Film crystalline and phase structure were characterized using X-ray diffraction (XRD) X-ray with diffraction (EPMA, EPMA-1610, Shimazu, Japan). So, the scanning was performed from 20° to 90° at an incident angle of 1°. (Fig1.) shows the X-ray diffraction pattern of the AlTiN/AlTiSiN multilayered composite coating prepared under different modulation periods. It can be seen from the (Fig1.) that all the AlTiN/AlTiSiN coatings contain FCC-(Ti, Al) N and HCP-AlN phases, with 2 at 42.77 ° and 58.681 °, respectively. With the change of modulation period, no new diffraction peaks appear in the coating, and with the decrease of modulation period, the diffraction peaks of the coating are slightly smooth and the intensity slightly reduced, in other words, the broadening of the diffraction peak indicates that the coating grains are refined. [19,20]
This is because, with the decrease of modulation period, the number of coating layers becomes more, the thickness of the single-layer decreases, and grain growth is inhibited. It is alo clear from the (Fig1.) that all the curves are rough, on the one hand, it is due to the large proportion of the amorphous phase in the coating; on the other hand, the multilayer structure restricts the grain growth of the coating. There is no diffraction peak of Si or Si compound in the diagram, which indicates that the Si element may exist in the form of amorphous or solid solution in (Ti, Al) N phase lattice.
Crystallinity =
Area of all peaks (crystalline + amorphous)
× 100
The pitting of AlTiN/AlTiSiN coating was carried out by Anton Paar tri Tec SA type pitting instrument (speed 500 rpm, time 600 s). The thickness of the coating can be obtained by observing the shape of the pits with a three- dimensional microscope.
The hardness (H) and elastic modulus (E) of the AlTiN/AlTiSiN coatings were measured by the continuous rigidity method with G300 nanoindentation instrument, and the H/E and H3/E*2 values were calculated; The nanoindentation instrument is used to test the hardness of the prepared coatings with a load of 10mN and taking an average of 10 values. The selected load of our scratch experiment is 120N, the scratch length is 6mm, and we use the scratch tester (Anton Paar RST3) to test our samples.
The High-temperature friction and wear tester (Anton Paar THT) was used to test the average friction coefficient of the film at room temperature. Al2O3 ball was selected as the pair of wear pairs. The applied load was 2N, the radius was 8mm, and 2500 cycles were set. The average value was taken three times for each coating type experiment.
After the friction test of the coating, blow off the generated debris. The cross-sectional area of wear mark is measured by
Fig 1. X-ray diffraction patterns of AlTiN/AlTiSiN multilayer coatings at different modulation period
Crystallinity is the degree of structural order in a solid. We can obtain crystallinity from our XRD data. Our experiment shows that a high degree of crystallinity 3.08 was obtained at a modulation period of 500 nm. Degree of Crystallinity has a high influence on hardness density, transparency, and diffusion. In various orientations separated by grain boundaries, they also contain other crystallographic defects, such as dislocations. Dislocation effects that reduce the degree of structural perfections of our coatings. The calculation of crystallinity by XRD is based on the
presumption that the sharp peak comes from the crystal phase and the broad peak comes from the amorphous phase. So, we should distinguish these two kinds of peaks on an XRD pattern at first. the sharp peaks become slightly broad.
-
Surface and section morphology analysis
The surface morphologies and cross-sectional topography of the AlTiN/AlTiSiN multilayered composite coatings were investigated under different modulation periods using SEM are shown in Fig2. As can be seen from Fig2. (a-d), the surface of the coatings was smooth and dense, this is because the target is used alternately under the multi-layer structure mode, so the surface temperature of the target is lower due to the short use time of the target, so the number of droplets evaporated from the target is greatly reduced, and the surface quality of the coating is greatly improved. It can be seen from the surface morphology that there are still large particles and pits, which is also a typical feature of the coating prepared by arc ion plating technology, and the number of surface defects increases with the increase of modulation period.
Fig2. (e-h) shows the cross-section morphology of the coatings, which show that the coatings are dense and uniform without obvious columnar crystal structure, on the one hand, the Si element in the coating can inhibit the columnar structure of the coating; on the other hand, the multilayer structure can effectively inhibit the grain growth. The transition layer TiN has a good combination with surface AlTiN/AlTiSiN working layers and monocrystalline silicon substrate and the highest thickness of coatings was about 2.57 m is obtained at the modulation period of 300 nm.
b c d
g h
Fig 2. Surface and cross sectional morphologies of the AlTiN/AlTiSiN coatings deposited at (a,e)=300nm,(b,f) =375nm, (c,g)=500nm,(d,h)=750nm modulation period.
-
Adhesion analysis of AlTiN/AlTiSiN coating Many factors affect the critical load, such as the adhesion between the film and substrate, the adhesion between the transition layer and the substrate, the adhesion between the transition layer and the coating, as well as the thickness and structure of the coating. Therefore, the tangential force, the scratch morphology of the coating, and the load when the coating and the substrate are completely peeled off are taken into account. The good adhesion of the films is due to high metal ion energy. In this experiment, the critical load of the coating was tested by the scratch tester and select the position where the coating and the substrate begin to peel as the critical load (Lc2). The experiment indicates that the critical load of AlTiN/AlTiSiN multilayered coatings decrease from 108.9N to 99.3N as the modulation period increases from 300nm to 750nm. (Fig3.) This is because when the modulation period is 300nm, the coating contains more layers, the stress at the crack tip can be absorbed effectively and the crack growth can be prevented, thus the adhesion between the film and the substrate can be effectively improved. Besides, when the modulation period is 300nm, the grain size of the coating is minimum. Under the complex stress, the small-sized nanocrystals can slip or tilt slightly, which can buffer and release part of the stress at the interface, which is not conducive to crack initiation and film peeling, and is helpful to enhance the adhesion of the coating and at this time, the coating defects are less and the structure is compact, which is helpful to improve the critical load of the coating. On the whole, the adhesion of the coating is high, which is also the advantage of using arc ion plating technology.
Fig 3. Scratch morphology of AlTiN/AlTiSiN Coatings Deposited at different modulation period
-
Analysis of hardness and elastic modulus of AlTiN/AlTiSiN coating
Fig4. shows that with the decrease of the modulation period, the hardness of multilayers increased gradually. When the modulation period is 300 nm, the hardness is up to 30 GPa, and when the modulation period is 750 nm, the modulus of elasticity is up to 369.1 GPa. This is mainly due to the increase in the number of layers, the increase of the number of interlaminar interfaces, the repeated nucleation between interfaces. The grain size of the multilayer film is more refined than that of the monolayer film, which makes the density of the film increase, according to the theory, the smaller the grain is, the better the hardness of the coating is [ 21], finally leads to the increase of the hardness of the coating. Also, with the decrease in the number of layers, the number of droplet particles in the film increases. Because the droplet is a kind of combined metal particle, its existence will reduce the hardness of the film, and the number of defects will reduce the compactness of the coating. So, the hardness of AlTiN/AlTiSiN multilayered coatings is the highest when the modulation period is 300nm.
To increase the resistance to plastic deformation, it is desirable to obtain materials that possess high hardness but lower elastic modulus values. With higher hardness and lower elastic modulus, the plastic deformation is lower, because the external load is distributed over a larger area and the stress distribution is more uniform, this behavior is then expressed by the H3/E2. [22] A high H3/E*2 ratio (where E*= E/(1-V2) is the effective Youngs modulus and V is the Poissons ratio) is valuable since it shows the resistance of the coating to crack. The values of H/E and H3/E*2 of AlTiN/AlTiSiN multilayered coatings decrease from 0.093 to
0.071 and 0.26GPa to 0.132GPa respectively as the modulation priod increases from 300nm to 750 nm as shown in (Fig4.), and at this time, the coating has the strongest ability to resist elastic strain and plastic deformation, which is helpful to improve the service performance of the coating.
[23]The values of Young's modulus are normally different for coatings and base materials. Different values of Young's modulus are recognizable when elastic stresses of concentrated contacts are calculated. A high Young's modulus of the coating increases the stress. The fatigue limit strongly depends on the internal stress conditions of the surface regions of the material. The stresses can be altered by coating and additional heat treatments. They are caused by different thermal expansion coefficients of the coating and the base material.
Fig 4. Loading and unloading curve and H/E and H3/E*2 at different modulation period
-
Friction analysis of AlTiN/AlTiSiN coating Fig5. shows the change curve of the average friction coefficient and wear rate of AlTiN/AlTiSiN multilayered coatings with different modulation periods. It can be seen from the (Fig5.) that when the modulation period is 300nm, the average friction coefficient and wear rate of the coating are the lowest 0.771 and 6.71×10-3 m3N-1m-1 respectively, and the two curves are gradually increasing with the increase of the modulation period, the friction curve is as shown in the (Fig6.). The friction process is very complex and affected by many factors. When the modulation period is 300 nm, the coating structure is compact, the hardness and the critical load are the highest, which are helpful to improve the tribological properties of the coating. Besides, H3/E*2 can effectively characterize the wear resistance of the coating. When the modulation period is 300 nm, the two values get the maximum value, indicating that the coating has high toughness and fracture resistance.
Fig7. shows the wear scar morphology of AlTiN/ AlTiSiN multilayered composite coatings deposited under different modulation periods. Through careful observation, it can be found that micro furrow exists in all the wear marks, which is because the exfoliated wear particles participate in the friction process in the initial wear stage and transfer to the contact interface between the AlTiN/AlTiSiN coatings and the Al2O3 friction pair, which are produced after they are squeezed and worn against each other. [24] At the same time, it can be seen that when the modulation period is 300 nm, the width of the wear scar is slightly smaller, and the coating wear rate is the lowest, so the wear mark depth is the shallowest. This is because when the modulation period is 300 nm, the coating hardness is the highest, and the contact area between the worn pair and the coating is small when the friction experiment is carried out, so the wear mark width and depth generated after repeated cycles of friction are narrower and shallowest. It is found that when the modulation period is 750 nm, there are the bluest and black wear debris in the wear marks. This is because the coating is soft and there are many defects in the coating, which aggravate the wear of the coating, and the oxidation reaction is intense. In conclusion, when the modulation period is 300 nm, the wear resistance of the coating is the best.
Fig 5. Friction coefficient and wear rate at different modulation period
decreased gradually, which was opposite to the increasing trend of hardness, critical load, H/E, and H3/E*2. when the modulation period was 300 nm, the values were 0.771 and 6.71×10-3 m3N-1m-1, respectively.
ACKNOWLEDGMENTS
This work was supported by the National Nature Science Foundation of China (51301181 and 51875555), the Tianjin Major Science and Technology Project (18ZXJMTG00050), and the Tianjin Natural Science Foundation (No.19JCYBJC17100).
Author contribution: Belay Abakuma Getachew performed the experiment and wrote the paper; Prof. Tie-gang Wang did guidance, advice, and constant support.
Conflict of interest: The author declares no conflict of interest
Fig 6. Friction curves at different modulation period
Fig 7. wear scar morphology of the AlTiN/AlTiSiN coating deposited at (a)
=300, (b)=375,(c)=500,(d)=750 modulation period
-
-
CONCLUSION
-
In this work, the microstructure, mechanical properties, and tribological properties of AlTiN/AlTiSiN multilayered coatings with different modulation periods were studied systematically. The results show that different modulation periods have an important influence on the structure and properties of the coating.
-
With the change of modulation period, no new phase is produced in the coating, all the AlTiN/AlTiSiN coatings contain fcc- (Ti, Al) N and hcp-AlN phases. With the decrease of the modulation period, the diffraction peak intensity of the coating decreases gradually, and the grains are refined gradually.
-
With the decrease of the modulation period, the hardness and critical load of the coating increased gradually. When the modulation period was 300nm, the values were 30GPa and 108.9N, respectively, and the H/E and H3/E*2 of the coatings were the highest.
-
With the decrease of the modulation period, the average friction coefficient and wear rate of the coating
REFERENCES
-
Architecture of PVD coatings for metal cutting applications: A review Aharon Inspektor a, Paul A. Salvador
-
Chemical vapor deposition and physical vapor deposition coatings: Properties, tribological behavior, and applications K.H. Habig.
-
, . [J]. , 2007(07):29-32.
-
Guo Zheng, Chen Qiang, Wang Zhengduo, et al. Research progress of copper precursor by CVD / atomic layer deposition [J]. Journal of Vacuum Science and Technology, 2015, 35 (10): 1282-1290.
-
Xie Wenfeng, Liu Jiajia, Hernandez Karla, et al. Research progress of molybdenum disulfide film by chemical vapor deposition [J]. Journal of Wuhan University of technology, 2016, 38 (4): 31-37.
-
Liu Zhihong, Zhang Shuying, Liu Zhiyong, et al. Principle and research progress of powder materials prepared by chemical vapor deposition [J]. Powder metallurgy materials science and engineering, 2009, 14 (6): 359-364
-
Aydin O I, Hallam T, Thomassin J L, et al. Interface and strain effects on the fabrication of suspended CVD graphene devices [J]. Solid-State Electronics, 2015, 108: 75-83.
-
Wei Q P, Yu Z M, Ashfold M N R, et al. Effects of thickness and cycle parameters on fretting wear behavior of CVD diamond coatings on steel substrates [J]. Surface & Coatings Technology, 2010, 205 (1): 158-167.
-
Mechanical properties and failure modes of TiAl(Si)N single and multilayer thin films O. Durand-Drouhina, A.E. Santanaa, A. Karimia,*, V.H. Derflingerb, A. Schu¨tzeb.
-
Chen H W, Chan Y C, Lee J W, et al. Oxidation behavior of Si-doped nanocomposite CrAlSiN coatings[J]. Surface and Coatings Technology, 2010, 205(5):11891194.
-
. [J].
2011, (4): 214-216.
-
Bouzakis K D, Michailidis N, Skordarisa G, et al. Cutting with coated tools: Coating technologies, characterization methods, and performance optimization [J]. CIRP Annals-Manufacturing Technology, 2012, 61(2): 703-723.
-
Strnad G, Buhagiar J. Lastest developments in PVD Coatings for Tooling [J]. Scientific Bulletin of the Petru Major University of Targu Mures, 2010, 7 (XXIV) (1): 32-37.
-
Bobzin K, Brögelmann T, Kalscheuer C, et al. Hybrid dc MS/HPPMS PVD nitride and oxynitride hard coatings for adhesion and abrasion reduction in plastics processing [J]. Surface and Coatings Technology, 2016, 308: 349-359.
-
Characterization and mechanical properties of TiN/TiAlN multilayer coatings with different modulation periods Wei Yongqiang & Zong Xiaoya1 & Jiang Zhiqiang1 & Tian Xiubo
-
The influence of modulation peiods on the evolution of microstructure and mechanical properties of nanoscale HfN/HfB2 multilayers
Jiangang Yu, Lei Dong, Chun Li Yupeng Pan, Rongxin Wan, Hanqing Gu, Dejun Li
-
Influence of modulation period and modulation ratio on structure and mechanical properties of TiBN/CrN coatings deposited by multi-arcion platingS.Y. Zhou, S.J. Yana B. Han, B. Yang, B.Z. Lin, Z.D. Zhang
Z.W. Ai, V.O. Pelenovich D.J. Fu
-
Significant impact of individual surface and modulation structure on mechanical properties of NbN/NbB2 multilayer Na Liu, Lei Dong, Shangxiao Jin, Rongxin Wan, Hanqing Gu, Dejun Li,
-
S.Q. Wang, L. Chen, B. Yang, K.K. Chang, Y. Du, J. Li, T. Gang, Effect of Si addition on microstructure and mechanical properties of Ti-AlN coating, Int. J. Refract. Met. H. 28 (2010) 593-596.
-
L. Chen, S.Q. Wang, Y. Du, S.Z. Zhou, T. Gang, J.C. Fen, K.K. Chang,
Y.W. Li, X. Xiong, Machining performance of TiAlSiN coated inserts, Surf. Coat. Tech. 205 (2010) 582-586.
-
CHEN Y, DU H, CHEN M, et al. Structure and wear behavior of AlCrSiN-based coatings[J]. Applied surface science, 2016, 370: 176- 183.
-
Mechanical properties of gradient and multilayered TiAlSiN hard coatings Yin-Yu Chang, Shun-Jan Yang, Weite Wu, Yu-Chu Kuo ,
Jyh-Wei Lee , Chaur-Jeng Wang
-
Chen J, Li H, Beake B. Surface, and Coatings Technology[J], 2016, 308: 289// Wu Z L, Li Y G, Wu B Et al. Thin Solid Films[J], 2015, 597:197
-
Wang T G, Zhao S S, Hua W G, et al. Design of a separation device used in detonation gun spraying system and its effects on the performance of WC-Co coatings [J]. Surface and Coatings Technology, 2009, 203(12): 1637-1644.