
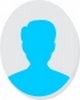
- Open Access
- Authors : Abeer M. Kutby , Hassan S. Al-Zahrani , Khalid R. Hakeem
- Paper ID : IJERTV9IS060188
- Volume & Issue : Volume 09, Issue 06 (June 2020)
- Published (First Online): 15-06-2020
- ISSN (Online) : 2278-0181
- Publisher Name : IJERT
- License:
This work is licensed under a Creative Commons Attribution 4.0 International License
Role of Magnetic Field and Brassinosteroids in Mitigating Salinity Stress in Tomato (Lycopersicon Esculentum L.)
Abeer M. Kutby, Hassan S. Al-Zahrani and Khalid R. Hakeem
Department of Biological Sciences, Faculty of Science, King Abdulaziz University, Jeddah, Saudi Arabia
Abstract:- This study was carried out to assess and evaluate the treatment of tomato seeds (var. Lycopersicon esculentum L.) with magnetic field (MF), and exogenous foliar application of 24-epibrassinolide (EBR) hormone on amelioration and alleviation of the harsh impact of salt stress on tomato growth, yield and biochemical parameters. A factorial experiment was designed with three replications. The experiment was carried out in pots inside the green house of the Faculty of Biological Science in King Abdulaziz University, in Jeddah. Plants were watered with saline water with (50-150-250mM) NaCl concentration. The seeds were pretreated with static magnetic field (SMF) with strengths of (50-100-150 mT) for (1h) period of time. The EBR hormone was applied as foliar spray on the leaf surfaces of tomato plants at three levels (0.5 -1-3 µM). The foliar application of EBR was done once a week during the experiment period. Salt stress markedly reduced all tomato plant studied growth parameters, protein content, and increased contents of proline and lipid peroxidation. After treating tomato seeds with MF and applying foliar or spraying of hormone on tomato seedling leaves significant enhancement and improvement in all studied tomato growth, yield, biochemical parameters and improvement in content of protein and proline occurred in plants grown under salt stress conditions. It is recommended to co- apply magnetic field with strength of 150 mT for 1 h to tomato seeds with foliar application of 24-epibrassinolide (EBR) at level of 3 µM.
Key words: Salt stress, Tomato ( Lycopersicon esculentum L), magnetic field (MF), 24-epibrassinolide (EBR), oxidative stress, SOD, APX, CAT, GR, proline, lipid peroxidation, MDA , mineral elements.
I.INTRODUCTION
Salinity is one of the most important abiotic stresses, limiting crop production in arid and semi-arid regions, where soil salt component is naturally high and precipitation can be insufficient for leaching (Shrivastava and Kumar, 2015). Salinity stress is a term related to the accumulation of excessive concentrations of salts, especially NaCl in soil or water and negatively affects the growth and development of many morphological and physiological characteristics of plants (Jamil et al., 2011). Salinity leads to ion toxicity followed by disruption of osmotic balance. NaCI in soil reduces absorption of water and (K+) ions in plant cells, and increases accumulation of Na+ to toxic levels and produces reactive oxygen species (ROS) thus reducing plant growth and yield (Munns and Tester, 2008; Bojrquez Quintal et al., 2012).
Magnetic field usually measured in terms of its magnetic induction B whose unit is given in Tesla (T). Krylov and Tarakonova (1960) were among the rst to report on magnetic field effects on plants. Magnetic fields augmented the germination percentage in many seeds, and the magnetic fields -treated plants grow earlier than untreated ones (Shine et al. 2017). The auxin-like effect of MF was also suggested to explain ripening of tomato fruits (Boe and Salunkhe, 1963). Magnetic treatment was found one of the significant techniques known to reduce various abiotic and biotic stresses, including drought stress (Selim and El-Nady, 2011) and salinity stress (Thomas, et al., 2013; Kataria et al. 2017). Magnetic field is known to alleviate salt stress in plants without harming their environmental status, and at the same time influence the increase of yield in crops and improve organogenesis, growth, biomass accumulation under salt stress (Radhakrishnan and Kumari, 2013; Rathod and Anand, 2016; Baghel, et al., 2016). To counter the problems of salinity, plants modify themselves by different means including detoxification of ROS through antioxidant enzymes and molecules, and induction of Phyto-hormones (Munns and Tester, 2008; Ruiz-Lozano et al., 2012). Phytohormones, are plant growth regulators which can mediate growth and development responses under ambient and stressful conditions (Peleg and Blumwald 2011). Growth and development in the sessile plants is regulated by the activity of some Phyto-hormones like brassinosteroids (BRs), which control many physiological and bio-chemical processes (Iqbal et al. 2014). Endogenous application of Phyto-hormones modulates physiological responses in plants that ultimately lead to adaptation as is the case under salinity (Khan, et al., 2013), and exogenous application of Phyto- hormones copes with salt stress (Iqbal, et al., 2012). Brassinosteroids (BRs) are a new group of Phyto-hormones with significant growth promoting properties and natural compounds of steroidal structure which have demonstrated to protect plants under saline stress. Brassinosteroids are involved in many other development processes, including flowering, leaf senescence, pollen development and male sterility, chloroplast development, and root growth and development (Yu et al., 2011; Ye et al., 2010; Mussig et al., 2003). The aim of the study is to evaluate the effect of tomato seeds pretreatment with magnetic field and foliar spray of (Brassinosteroids) hormone in reducing the negative impact of salt stress conditions in growth of tomato plant.
-
MATERIALS AND METHODS
2.1 Effect of Magnetic Field (MF) treatments and foliar spray of brassinosteroids on tomato growth parameters under salinity:
The experiment was conducted in pots, in the green house of Department of Biological sciences at King Abdulaziz University, Jeddah, KSA Factorial design was used in this experiment. MF at strength of 50 mT, 100 mT and 150 mT and foliar spray of brassinosteroids at different levels of 0.5 µM, 1 µM and 3 µM were experimented on tomato growth parameters growing under different NaCl concentrations 50 mM, 150 mM and 250 mM. The seedlings separated into five groups for different treatments as follows:
(1) Control. (2) Seedlings under salinity 50, 150, 250 mM NaCl without MF or hormone treatment. (3) Pre- treatments with magnetic field divided into 9 subgroups of 50, 100 and 150 mT, watered with saline water. (4) Seedlings treated with EBR hormone divided into 9 subgroups with 0.5, 1, 3 µM concentrations, irrigated with saline water. And (5) seedlings treated with both MF and hormone, and watered by saline water, to give a total of 08 pots and 618 plants. Plants were watered regularly with 1/2 strength Hoaglands nutrient solution 0, 50, 150 and 250 mM NaCl, respectively.
-
Magnetic Field treatment:
An electromagnetic field generator Testron EM-20 was used and seeds were primed with variable horizontal magnetic field strength (50500 mT) and a gap of 5 cm between pole pieces (Vashisth and Nagarajan 2008). The pole pieces were in cylindrical shape, with a diameter of 9 cm and 16 cm in length. The number of turns per coil was 3,000 and the resistance of the coil was 16 ohms. A DC power supply (80 V / 10 A) with continuously variable output current was used for the electromagnet. The digital Gauss meter (model DGM-30-Testron) measured the magnetic field strength produced between the poles. The probe is made up of indium arsenide crystal and encapsulated to a nonmagnetic sheet of 5 × 4 × 1 mm; it could measure 02 T with the full- scale range in increments of 5 mT. The variation in temperature during the course of seed exposure was 25 ±1°C. Under a uniform magnetic field, hundred seeds kept in the plastic container were placed etween both poles of the electromagnet (Shine, et al. 2011).
-
Brassinosteroids Treatment:
The brassinosteroids 24-epiBL (90% in purity; PANPAN Industry, Zhengzhou, China) was prepared by first dissolving 0.1 mg 24-epiBL in 10 mL absolute ethanol followed by diluting the solution with Milli-Q water [ethanol: water (v/v) = 1:10,000] (Ahammed et al. 2013). The hormone was sprayed on tomato leaves at concentration of (0.5,1,3
µM).
-
Soluble Protein Content:
The total soluble protein content of the leaves was estimated following the method of Bradford (1976). A 0.5 g of chopped fresh leaf material was homogenized in 5 mL of 0.1M phosphate buffer. The homogenate was centrifuged at
5000 rpm for 10 min at 4 0C.1.0 mL chilled 10% TCA was added to 1.0 mL of the supernatant, which was again centrifuged at 3300 rpm for 10 min. The supernatant was discarded and the pellet left was washed with acetone. It was then dissolved in 1 mL of 0.1N NaOH. To a 1.0 mL of aliquot, 5 mL of the Bradfords reagent was added and vortex mixed. The tubes were left for 10 min for optimal color development. The absorbance was then recorded at 595 nm on a UV-Vis spectrophotometer (BIO 20, Perkin Elmer, Germany). The soluble protein content was quantified with the help of a standard curve prepared from the standard of Bovine Albumin Serum (BSA). The protein content was expressed in mg g-1 FW.
-
Lipid Peroxidation:
The level of lipid peroxidation in the leaves was determined as malondialdehyde (MDA) content by the method of Heath and Packer (1968). Fresh tissue of leaf was ground in 0.1 % TCA and centrifuged at 10, 000 rpm for 5 min. 1.0 mL of supernatant was taken in a separate test tube, to which 4.0 mL of 0.5 % TBA was added. The mixture was heated at 95 C for 30 min. It was then quickly cooled in an ice bath and re-centrifuged at 5000 rpm for 5 min to suspend the turbidity. The absorbance of the supernatant was read at 532 nm and 600 nm, corrected for unspecific turbidity by subtracting the value at 600 nm. The blank was 0.5 % TBA reagent. The concentration of MDA was calculated using an extinction coefficient () of 155 mM-1 cm-1 as given in the following formula:
MDA (nmol g1 FW) = (A532 – A 600) x V ( x W) x 1000
Where, A532 = Absorbance at 532 nm; A600 = Absorbance at 600 nm; V = Extraction volume; W = Fresh weight of tissue.
-
Proline content:
The proline levels of the leaves were estimated following the method of Bates et al (1973). 500 mg of fresh leaf material were homogenized in 10 ml of 3 % aqueous sulphosalicylic acid. The homogenate was centrifuged at 10,000 rpm for 10 min. 2 mL of supernatant, 2 mL of glacial acetic acid and 2 mL of acid ninhydrin were taken in a test tube. This mixture was incubated for 1 h at 100 0C to complete the reaction. The reaction was terminated by putting the tubes in ice bath. After the completion of incubation, the mixture was extracted with 4 mL of toluene. The extract was vigorously vortex for 20s. The chromatophore containing fraction was then aspirated from the aqueous phase and the absorbance determined at 620 nm using spectrophotometer ( BIO 20, Perkin Elmer, Germany). L- proline was processed in the same manner to prepare the standard curve. The amount of proline was expressed as nmol g1 FW.
-
Statistical analysis:
One-way NOVA using Graph Pad Prism 6.0 software by Tukeys post hoc test (p<0.05). The experiment was carried out in triplicates (n=3) except for growth parameters (root and shoot length, fresh weight, dry weight and RWC, where n=3).
Two-way analysis of variance were used to examine the effects of two independent variables (factors), both individually and together.
-
-
RESULTS
3.1 Effect of different concentrations of NaCl, MF strengths and Foliar Spray of 24-epibrassinolide on growth of tomato:
Table (1) shows that tomato plants present high significant differences (P 0.01) in growth parameters (plant height, shoot and root fresh and dry weight) under NaCl, MF and EBR hormone different concentrations. Plant height, growth of shoot and root were significantly reduced under all NaCl concentrations, and highly significantly increased under all MF strengths and all levels of the hormone. Plant height decreased at rates of (15.82, 30.81, 42.99%), shoot fresh and dry weight significantly decreased at rates of (13.28, 45.89, 58.52% in fresh, and 41.56, 60.07, 74.45 % in
dry weight) respectively. Root fresh and dry weight significantly decreased at rates of (9.11, 40.02, 53.18 % in fresh weight and 22.52, 58.36, 67.23 % in dry weight) respectively under NaCl concentrations (0, 50, 150, 250
mM). Where (plant height, shoot and root fresh and dry weight) showed successive and significant high (P 0.01) increases and improvement with application of MF and EBR hormone. Plant height increased at rate of (84.59, 71.15, 85.86 %), under MF and at rates of (76.38, 78.02, 87.20 %) under hormone effect. Shoot fresh and dry weight increased at rates of (261.92, 262.92, 262.70 % in fresh weight and 312.03, 320.81, 352.60 % in dry weight). Root fresh and dry weights increased at rates of (220.77, 236.36, 212.23 % and 190.62, 194.79, 210.41 %) respectively under MF levels (50,
100, 150 mT). And under EBR (0.5, 1, 3 µM) sprayed on the leaves increase in shoot fresh weight reached (155.14, 185.81, 213.38 %) and shoot dry weight (114.70, 131.16,
152.59 %) while in root fresh and dry weight the increases were at rates of (152.03, 198.31, 242.33 % and 92.7, 125, 150
%) respectively at the different EBR concentrations (0.0, 0.5, 1, 3 µM). It can be seen that the significantly highest reductions in all studied parameters occurred under 250 mM NaCl concentration. And the significant highest percentage increases of plant height was under 100 mT MF and 1 µM EBR hormone, and for shoot and root fresh and dry wt. the highest values were under 150 mT MF and 3 µM EBR hormone.
TABLE (1): EFFECT OF DIFFERENT CONCENTRATIONS OF NACL, MF STRENGTHS AND FOLIAR SPRAY OF 24-EPIBRASSINOLIDE ON PLANT HEIGHT, SHOOT AND ROOT FRESH AND DRY WEIGHT ON TOMATO.
Treatments
Plant Height [cm)
Fresh Weight (g) plant1
Dry weight (g) plant1
shoot
root
shoot
root
Salt concentration (mM)
0
33.49 a
61.45 a
31.70 a
5.93 a
3.19 a
50
28.19 b
54.28 b
30.86 b
4.64 b
3.02 b
150
23.17 c
34.32 c
21.11 c
3.39 c
2.01 c
250
19.09 d
27.98 d
14.90 d
2.57 d
1.33 d
LSD
0.273
0.40
0.37
0.03
0.03
F
**
**
**
**
**
Magnetic field
0
15.71 c
14.87 c
7.11 d
1.54 d
0.96 d
50
29.00 b
53.80 b
29.36 c
4.94 b
2.79 c
100
30.03 a
54.67 a 29.92 b
5.18 a
2.83 b
150
29.20 b
54.68 a
32.18 a
4.87 c
2.98 a
LSD
0.38
0.43
0.37
0.03
0.02
F
**
**
**
**
**
Hormone
0
15.71 d
14.87 d
7.11 c
1.54 d
0.96 c
0.5
27.71 c
53.27 c
28.35 b
4.82 c
2.85 b
1
31.11 a
53.94 b
31.57 a
5.02 b
2.84 b
3
29.41 b
55.95 a
31.54 a
5.14 a
2.91 a
LSD
0.19
0.29
0.27
0.03
0.029
F
**
**
**
**
**
*: Means followed by the same letter are not significantly different according to RLSD. NS: Not significant. *: significant at P0.05. **: significant at P0.01
-
Effect of interaction between different NaCl concentrations and foliar spray of 24-epibrassinolide on tomato growth:
Foliar application of the hormone EBR gave successive and highly significant (P 0.01) improvement and enhancement in tomato growth parameters (plant height, shoot and root fresh and dry weights) under the influence of salinity with different NaCl concentrations (0, 50, 150, 250 mM; Table 2). Application of the hormone EBR at all rates (0.5, 1, 3 µM) significantly increased studied parameters of tomato plants under all NaCl concentrations, and increases
of plant height reached (34.34, 50.16, 74.43 %) at 250 mM NaCl, shoot fresh and dry weight under EBR 3 µM reached rates of (799.79, 1484.61 %) respectively at 250 mM NaCl. In addition, in root fresh and dry weight increase in rates under 3 µM EBR reached (1405 and 3550 %) at 250 mM NaCl respectively compared to control without EBR hormone. The increase in these parameters significantly increased with increasing NaCl concentrations to reach maximum percentage at 250 mM. The highest increase in plant height appear at 0 and 250 NaCl occurred under 0.5
µM hormone, and under 3 µM in the case of shoot and root fresh and dry wt.
TABLE (2): EFFECT OF INTERACTION BETWEEN DIFFERENT NACL CONCENTRATIONS AND FOLIAR SPRAY OF 24-EPIBRASSINOLIDE ON PLANT HEIGHT, SHOOT AND ROOT FRESH AND DRY WEIGHTS (MG PLANT1) ON TOMATO.
Treatments
Plant Height (cm)
Fresh Weight (g) plant1
Dry weight (g) plant1
shoot
root
shoot
root
Salt concentration (mM)
Hormone (µM)
0
0
25.13
27.26
12.40
2.80
1.72
0.5
38.60
72.62
34.91
6.92
3.74
1
37.94
72.03
39.03
6.90
3.56
3
32.31
73.90
40.45
7.10
3.74
50
0
17.46
18.93
10.40
2.03
1.54
0.5
30.90
64.85
35.08
5.24
3.37
1
32.11
65.50
39.54
5.64
3.54
3
32.31
67.84
38.44
5.66
3.62
150
0
11.03
10.06
4.50
1.23
0.53
0.5
25.76
40.17
23.84
3.75
2.38
1
28.21
42.93
27.86
4.36
2.57
3
27.68
44.13
28.25
4.23
2.55
250
0
9.23
3.23
1.16
0.13
0.04
0.5
20.74
35.43
19.57
3.38
1.88
1
21.88
35.31
19.84
3.17
1.67
3
24.51
37.94
19.03
3.58
1.74
LSD
0.67
0.51
0.48
0.06
0.05
F
**
**
**
**
**
*: Means followed by the same letter are not significantly different according to RLSD. NS: Not significant. *: significant at P0.05. **: significant at P0.01
-
Effect of interaction between different NaCl concentrations and strengths of MF on plant height, shoot and root fresh and dry weights (g) plant1 of tomato:
Magnetic field application gave successive and highly significant (P 0.01) improvement and enhancement in tomato growth parameters (shoot and root fresh and dry weights) under the influence of salinity with the different NaCl concentrations (0, 50, 150, 250 mM; Table 3). At 50 MF plant height increased at rates of (49.5, 76.97, 124.70
%); shoot fresh weight increased at rates of (209.22, 205.54 and 200.83 %); shoot dry weight increased at rates of (210.74, 317.13 and 320.80 %). Root fresh weight increased at rates of (155.84, 111.68 and 174.15 %) while root dry weight increased at rates of (114.79, 133.33, 161.16 %)
respectively at NaCl concentrations (50, 150, 250 mM). At 150 MF plant height increased at rate of (24.59, 85.05,
165.54 %); shoot fresh weight increased at rates of (521.35,
441.25 and 397.74 %) and shoot dry weight increased at rates of (244.44, 367.22 and 246.27 %). Root fresh weight increased at rates of (211.03, 224.67 and 212.33 %) and root dry weight increased at rates of (148.33, 208.33 and 154.58
%) respectively at NaCl concentrations (50, 150, 250 mM) compared to control.
It can be seen that under control, te highest plant height was attained at 50 mT MF, and highest shoot fresh and dry wt. was under 100 mT MF, while for root fresh and dry wt. the highest values were under 150 mT MF. Moreover, at 250 mM NaCl highest increases were under 150 mT MF for all these mentioned parameters.
TABLE (3): EFFECT OF INTERACTION BETWEEN DIFFERENT NACL CONCENTRATIONS WITH STRENGTHS OF MF ON PLANT HEIGHT, SHOOT AND ROOT FRESH AND DRY WEIGHTS (G PLANT1) ON TOMATO.
Treatments
Plant Height [cm)
Fresh Weight (g) plant1
Dry weight (g) plant1
shoot
root
shoot
root
Salt concentration (mM)
Magnetic field
0
0
25.13
27.26
12.40
2.80
1.72
50
38.60
73.67
37.06
7.43
3.68
100
37.94
76.30
35.72
7.72
3.55
150
32.31
68.57
41.61
5.76
3.81
50
0
17.46
18.93
10.40
2.03
1.54
50
30.90
64.80
34.57
5.27
3.16
100
32.11
64.82
36.88
5.51
3.56
150
32.31
68.57
41.61
5.76
3.81
150
0
11.03
10.06
4.50
1.23
0.53
50
25.76
40.14
25.92
3.75
2.63
100
28.21
42.06
27.71
4.08
2.44
150
27.68
45.03
26.33
4.51
2.44
250
0
9.23
3.23
1.16
0.13
0.04
50
20.74
36.61
19.87
3.30
1.67
100
21.88
35.52
19.38
3.40
1.77
150
24.51
36.55
19.18
3.45
1.85
LSD
0.67
0.75
0.66
0.05
0.04
F
**
**
**
**
**
*: Means followed by the same letter are not significantly different according to RLSD. NS: Not significant. *: significant at P0.05. **: significant at P0.01
3.4 Effect of interaction between different strengths of MF and foliar spray of 24-epibrassinolide on plant height, shoot and root fresh and dry weights (g) on plant1 of tomato:
Foliar application of the hormone EBR gave successive and highly significant (P 0.01) improvement and enhancement in tomato growth parameters (shoot and root fresh and dry weight) at all MF strengths (50, 100, 150 mT; Table 4). At 50 MF plant height increased at rates of (70.84, 92.36, 90.57 %); shoot fresh weight increased at
rates of (249.22, 255.54, 280.83%) and shoot dry weight increased at rates of (270.74, 337.13, 330.80 %). While root fresh weight increased at rates of (205.84, 211.68, 244.15 %) and root dry weight increased at rates of (194.79, 183.33,
191.16 %) respectively at levels of EBR hormone (0.5, 1, 3
µM). At 150 MF plant height increased at rate of (82.04,
91.66, 83.89 %); shoot fresh weight increased at rates of (821.35, 841.25, 797.74 %) and shoot dry weight increased at rates of (344.44, 367.22 and 346.27 %). Root fresh weight increased at rates of (211.03, 224.67, 212.33 %) and root dry weight increased at rates of (208.33, 208.33, 214.58 %) respectively at levels of EBR hormone (0.5, 1, 3 µM). compared to control without EBR hormone.
It can be seen that the highest increase in tomato plant height was attained at 100 mT MF with 1 µM EBR hormone, and for shoot fresh and dry weight under 100 mT MF at 3
µM EBR, while for root fresh and dry wt. it was at 150 mT MF with 1 and 3 µM EBR respectively.
TABLE (4): EFFECT OF INTERACTION BETWEEN DIFFERENT MF STRENGTHS WITH FOLIAR SPRAY OF 24-EPIBRASSINOLIDE ON GROWTH OF PLANT HEIGHT, SHOOT AND ROOT FRESH AND DRY WEIGHTS (G PLANT1) ON TOMATO.
Treatments
Plant Height [cm)
Fresh Weight (g) plant1
Dry weight (g) plant1
shoot
root
shoot
root
Magnetic field
Hormone (µM)
0
0
15.71
14.87
7.11
1.54
0.96
50
0.5
26.84
51.93
26.36
4.71
2.83
1
30.22
52.85
31.08
4.80
2.74
3
29.94
56.63
30.63
5.30
2.80
100
0.5
27.69
53.07
27.10
4.97
2.76
1
33.00
53.50
30.40
5.24
2.81
3
29.41
57.45
32.27
5.32
2.92
150
0.5
28.60
54.80
31.60
4.79
2.95
1
30.11
55.46
33.22
5.01
2.96
3
28.89
53.78
31.73
4.81
LSD
0.33
0.51
0.48
0.06
0.05
F
**
**
**
**
**
**
*: Means followed by the same letter are not significantly different according to RLSD at P0.05.NS: Not significant at P0.05
*: significant at P0.05**: significant at P0.01
-
Effect of interaction between different NaCl concentrations with magnetic field and foliar spray of 24-epibrassinolide on growth parameters (plant height (cm), shoot length [cm], root length [cm] leave area [cm2] on tomato.
The results in (table 5) illustrate significant differences in the interactions between effects of NaCl concentrations, MF magnitudes and the hormone EBR on tomato growth parameters (plant height, shoot and root fresh and dry weight per plant (gm)]. MF and EBR hormone application resulted in significantly high percentage increasing growth parameters in tomato under all NaCl concentrations (0, 50, 150, 250 mM). Under 50 NaCl concentration the maximum value percentage were (49.97 %) in plant height under 100 MF and 1 µM EBR. Shoot fresh weight was (215.59 %) under MF 150 µT and EBR 1 µM, in shoot dry weight (298.07 %) under MF 150 µT and EBR 1 µM; root fresh weight (195.51 %) at MF 150 µT and EBR 1 µM; root dry weight (150.13
%) under MF 150 µT and EBR 3 µM. Under 150 NaCl concentration the maximum percent increase in plant height was (29.09%) under 100 MF and 3 µM EBR, shoot fresh wt. was (1446.11%) under MF 150 µT and EBR 1 µM, in shoot dry wt. (516.44%) under MF 150 µT and EBR 3 µM, root fresh wt. (260.16%) at MF 150 µT and EBR 1 µM, root dry wt. (385.13%) under MF 150
µT and EBR 3 µM. Under 250 NaCl concentration the maximum percent increase in plant height was (180.93%) under 150 MF and 1µM EBR, shoot fresh wt. was (350.89%) under MF 50 µT and EBR 3 µM, in shoot dry wt. (516.44%) under MF 50 µT and EBR 1 µM, root fresh wt. (260.16%) at MF 150 µT and EBR 1 µM, root dry wt. (4555.14%) under MF 150 µT and EBR 1 µM.
The highest percentage increases in plant height and shoot and root wt. were attained by the hormone at the highest salinity stress 250 mM under all MF strengths. It seems that the effect of the hormone was more pronounce under high salinity rate than under low rates. EBR hormone significantly enhanced tomato shoot and root growth and alleviated effect of salinity under the highest NaCl concentration 250 mM compared to the lower NaCl concentrations.
Table (5): Effect of interaction between NaCl concentration with magnetic field and foliar spray of 24-epibrassinolide on tomato growth.
Treatments
Plant Height (cm)
Fresh Weight (g) plant1
Dry weight (g) plant1
shoot
root
shoot
root
NaCl (mM)
MF
Hormone (µM)
0
0
0
25.13
27.26
12.40
2.80
1.72
50
0.5
35.06
76.26
33.03
7.43
3.83
1
38.76
70.80
38.56
7.13
3.63
3
41.96
73.96
39.60
7.73
3.60
100
0.5
36.66
73.10
30.23
7.60
3.53
1
41.03
76.23
35.40
7.70
3.36
3
36.13
79.56
41.53
7.86
3.76
150
0.5
34.03
68.50
41.46
5.73
3.86
1
31.96
69.06
43.13
5.86
3.70
3
30.93
68.16
40.23
5.70
3.86
50
0
0
17.46
18.93
10.40
2.03
1.54
50
0.5
28.96
61.63
30.63
4.60
3.10
1
33.20
64.13
37.03
5.43
3.26
3
30.53
68.63
36.06
5.80
3.13
100
0.5
30.16
64.43
33.16
5.40
3.16
1
35.00
63.30
38.46
5.63
3.66
3
31.16
66.73
39.03
5.50
3.86
150
0.5
34.03
68.50
41.46
5.73
3.86
1
31.96
69.06
43.13
5.86
3.70
3
30.93
68.16
40.23
5.70
3.86
150
0
0
11.03
10.06
4.50
1.23
0.53
50
0.5
24.16
36.10
22.56
3.36
2.56
1
26.76
40.46
27.16
3.80
2.53
3
26.36
43.86
28.03
4.10
2.80
100
0.5
22.86
39.06
25.20
3.46
2.43
1
32.26
42.10
29.13
4.53
2.63
3
29.50
45.03
28.80
4.26
2.26
150
0.5
24.70
45.36
23.76
4.43
2.16
1
30.60
46.23
27.30
4.76
2.56
3
27.76
43.50
27.93
4.33
2.60
250
0
0
9.23
3.23
1.16
0.13
0.04
50
0.5
19.16
33.73
19.23
3.46
1.83
1
22.16
36.03
21.56
2.86
1.53
3
20.90
40.06
18.83
3.56
1.66
100
0.5
21.06
35.70
19.80
3.43
1.93
1
23.73
32.40
18.63
3.10
1.60
3
20.86
38.46
19.73
3.66
1.80
150
0.5
21.66
36.86
19.70
3.26
1.90
1
25.93
37.50
19.33
3.56
1.90
3
25.93
35.30
18.53
3.53
1.76
LSD
0.33
0.51
0.48
0.06
0.05
F
**
**
**
**
**
*: Means followed by the same letter are not significantly different according to RLSD. NS: Not significant. *: significant at P0.05. **: significant at P0.0.
-
Effect of different NaCl concentrations with MF strengths and foliar spray of 24-epibrassinolide on number of leaves plant-1, leaf area, number of flowers and number of fruits plant1, on tomato.
Table (6) shows that tomato plants have undergone high significant differences (P 0.01) in growth parameters (number of leaves plant-1, leaf area, number of flowers/plants, number of fruits/plant) under NaCl concentrations, MF effects and EBR different concentrations. Number of leaves/plant reduced at rates of (24.07, 33.70, 49.38 %), leaf area reduced at rates of (17.83, 32.14, 47.22 %), flowers/plant significantly decreased at
rates of (2.04, 21.92, 43.6 %), fruits/plant reduced at rate of (7.82, 32.63, 44.34 %), respectively under salinity stress of 50, 150, 250 mM compared to control. All these parameters significantly highly increased (P 0.01) with increase in MF (50, 100, 150 mT). Number of leaves /plant increased at rate of (104.73, 108.64, 97.18 %), and leaf area increased at rates of (136.50, 143.34, 1219.65 %), number of flowers/plant
reached (112.63, 136.70, 128.49 %) and number of
fruits/plant (164.44, 156.09, 172.04 %) respectively at the different MF (50, 100, 150 mT) compared to control without MF.
All these parameters significantly increased (P 0.01) with increasing concentration of EBR (0.5, 1, 3 µM) sprayed on the leaves. Number of leaves /plant increased at rates of (100.0, 101.23, 108.78 %), and leaf area increased at rates of (128.13, 133.16, 147.90 %), number of flowers/plant
reached (47.88, 70.56, 70.56 %) and number of fruits/plant
reached (81.23, 100.09, 115.57 %) respectively at the
different EBR concentrations (0.5, 1, 3 µM) compared to
control without EBR hormone. It can be seen that MF application at 100 level gave the highest significant increases in number of leaves/plants, leaf area and flowers/plant, compared to 50 and 150 levels, while MF 150 exceeded in number of fruits/plant. As for the hormone, concentration 3 µM dominated giving the highest increases of all these studied parameters compared to the other concentrations.
TABLE (6): EFFECT OF DIFFERENT NACL CONCENTRATIONS AND MF LEVELS WITH FOLIAR SPRAY OF 24-EPIBRASSINOLIDE ON NUMBER OF LEAVES PLANT-1, LEAF AREA, NUMBER OF FLOWERS AND NUMBER OF FRUITS PLANT1, OF TOMATO.
Treatments
Number of Leaves-1 plan
Leaf area cm2)
Number of Flowersplant1
Number of Fruits plant1
Salt Concentration (mM)
0
99.88 a
7.03 a
35.69 a
28.94 a
50
75.83 b
5.78 b
35.69 a
26.72 b
150
66.22 c
4.77 c
30.52 b
21.19 c
250
50.55 d
3.71 d
21.30 c
18.30 d
LSD
0.52
0.041
0.34
0.41
F
**
**
**
**
Magnetic Field
0
41.16 d
2.63 d
15.83 d
10.66 d
50
84.27 b
6.22 b
33.66 c
28.19 b
100
85.88 a
6.40 a
37.47 a
27.30 c
150
81.16 c
6.04 c
36.25 b
29.00 a
LSD
0.36
0.03
0.41
0.39
F
**
**
**
**
Hormone
0
41.16 d
2.63 d
15.83 d
10.66 d
0.5
82.36 c
6.00 c
34.13 c
26.75 c
1
82.83 b
6.14 b
36.41 b
28.38 b
3
86.13 a
6.52 a
36.83 a
29.36 a
LSD
0.27
0.04
0.31
0.25
F
**
**
**
**
*: Means followed by the same letter are not significantly different according to RLSD at P0.05. NS: Not significant at P0.05
*: significant at P0.05**: significant at P0.01
-
Effect ofinteraction between different NaCl concentrations with magnetic field on number of leaves plant-1, leaf area, number of flowers and number of fruits plant1, of tomato.
Application of MF highly significantly (P 0.01) improved and enhanced tomato growth parameters (number of leaves/plant and leaf area, number of flowers/plants, number of fruits/plant) under salinity of NaCl concentrations (0, 50, 150, 250 mM) (Table 7). Application of MF at
strengths of (50, 100, 150 mT) significantly increased all parameters under all NaCl concentrations. Under 50 mM NaCl percentage increase in number of leaves/plants reached (67.79, 64.11, 87.06 %) and leaf area (100.94, 109.17, 121.89 %), and number of flowers/plants reached
(106.95, 140.50, 117, 89 %), fruits/plant (179.43, 164.78,
199.03 %), under MF (50, 100, 150 mT) respectively. Moreover, under 250 mM NaCl concentration number of leaves/plants increased and reached (666.06, 800.36, 800.36
%) and leaf area (308.07, 331.42, 295.53 %), number of flowers/plants reached (142.00, 153.3, 156.6 %), number of
fruits/plant (130.94, 152.65, 161.66 %), under MF 50, 100,
150 mT respectively compared to control without MF. Application of MF ameliorated the harsh salinity impact on tomato number of leaves/plants, leaf area, number of flowers/plants, number of fruits/plants, particularly number of leaves/plants, leaf area, number of flowers/plants at all NaCl concentrations 50, 150, 250 mM. The best MF strength is 100 and 150 mT.
TABLE (7): EFFECT OF INTERACTION BETWEEN DIFFERENT NACL CONCENTRATIONS WITH MAGNETIC FIELD ON NUMBER OF LEAVES PLANT-1, LEAF AREA, NUMBER OF FLOWERS AND NUMBER OF FRUITS PLANT1, OF TOMATO.
Treatments
number of leaves plant1
Leaf area (cm2)
Number of Flower plant1
Number of Fruits plant1
Salt concentration (mM)
Magnetic field
0
0
68.66
4.13
21.66
12.33
50
118.00
8.42
37.77
36.66
100
121.22
8.58
42.66
32.88
150
91.66
7.01
40.66
33.88
0
49.00
3.16
18.66
11.33
50
50
82.22
6.35
38.55
31.66
100
80.44
6.61
44.88
30.00
150
91.66
7.01
40.66
33.88
150
0
38.66
2.13
13.00
10.33
50
73.00
5.53
34.11
24.44
100
76.88
5.70
37.00
24.44
150
76.33
5.73
38.00
25.55
250
0
8.33
1.12
10.00
8.66
50
63.88
4.57
24.22
20.00
100
65.00
4.72
25.33
21.88
150
65.00
4.43
25.66
22.66
LSD
0.64
0.06
0.72
0.69
F
**
**
**
**
*: Means followed by the same letter are not significantly different according to RLSD. NS: Not significant. *: significant at P0.05. **: significant at P0.01
-
Effect of interaction of different NaCl concentrations with foliar spray of 24-epibrassinolide on number of leaves plant-1, leaf area, number of flowers and number of fruits plant1, of tomato.
Foliar application of the hormone EBR gave successive and highly significant (P 0.01) improvement in tomato growth parameters (number of leaves/plant, leaf area, number of flowers/plant, number of fruits/plant) under salinity of different NaCl concentrations (0, 50, 150 , 250 mM) and the lowest values were at level 250 mM NaCl (Table 8). Application of the hormone EBR at all rates (0.5, 1, 3 µM) significantly increased all these parameters of tomato plants under all NaCl concentrations. Under control percentage increased in number of leaves number/plant
reached (39.81, 52.92, 544.17 %) and leaf area increased at rates of (53.75, 77.72, 190.17 %), flowers/plant reached
(34.34, 57.43, 43.58 %), number of fruits/plant reached
(105.43, 124.33, 132.44 %), under EBR levels of 0, 0.5, 1, 3
µM respectively. Under 250 mM NaCl concentration increased in leaves number/plant reached rates of (40.12, 51.02, 564.22 %) and leaf area increased at rates of (73.36, 72.46, 223.21 %), number of flowers/plant reached (56.6,
80.0, 93.3 %), number of fruits/plant (42.37, 74.36, 88.56
%), under EBR levels of 0.5, 1, 3 µM respectively compared to control without EBR hormone. Hormone application enhanced and increased all these studied parameters, with hormone concentration 3 µM dominating at all NaCl concentrations.
TABLE (8): EFFECT OF INTERACTION BETWEEN DIFFERENT CONCENTRATIONS OF NACL WITH DIFFERENT CONCENTRATIONS OF FOLIAR SPRAY OF 24-
EPIBRASSINOLIDE ON NUMBER OF LEAVES/PLANTS, LEAF AREA, NUMBER OF FLOWERS AND FRUITS PLANT1, OF TOMATO.
Treatments
number of leaves plant1
Leaf area (cm2)
Number of Flower plant1
Number of Fruits plant1
Salt concentration (mM)
Hormone (µM)
0
0
68.66
4.13
21.66
12.33
0.5
107.44
7.63
39.11
33.11
1
107.11
7.66
41.55
34.77
3
116.33
8.72
40.44
35.55
50
0
49.00
3.16
18.66
11.33
0.5
84.66
6.54
39.77
30.77
1
85.44
6.72
42.11
31.77
3
84.22
6.70
42.22
33.00
150
0
38.66
2.13
13.00
10.33
0.5
73.44
5.50
35.22
23.44
1
75.66
5.67
36.33
24.33
3
77.11
5.79
37.55
26.66
250
0
8.33
1.12
10.00
8.66
0.5
63.88
4.33
22.44
19.66
1
63.11
4.50
25.66
22.66
3
66.88
4.88
27.11
22.22
LSD
0.47
07.
0.55
0.44
F
**
**
**
**
*: Means followed by the same letter are not significantly different according to RLSD. NS: Not significant. *: significant at P0.05. **: significant at P0.01.
-
Effect of interaction between different MF strengths with different concentrations of foliar Spray of 24- epibrassinolide on number of leaves plant-1, leaf area, number of flowers and number of fruits plant1, of tomato plants.
Foliar application of EBR hormone gave successive and highly significant increasing (P 0.01) in tomato growth parameters (number of leaves plant-1, leaf area, number of flowers/plant, number of fruits/plant) together with the influence of the different MF strengths (50, 100, 150 mT; Table 9), and parameters increased with increase in hormone concentration levels and with MF strengths. Under MF 50 percentage increase in number of leaves/plant increased at rates of (93.95, 107.50, 112.77 %) and leaf area increased at
rates of (114.06, 135.73, 159.31 %), flowers/plant reached
(96.20, 111.41, 129.50 %), number of fruits/plant reached
(138.36, 152.43, 193.15 %), under EBR levels of 0.5, 1, 3
µM respectively. And under MF 150 percentage increase in number of leaves/plant increased at rates of (95.77, 92.70,
102.25 %) and leaf area increased at rates of (128.51, 127.75, 132.69 %), flowers/plant reached (116.36, 142.64,
127.92 %), number of fruits/plant reached (172.04, 173.54,170.26 %), under EBR levels of 0.5, 1, 3 µM respectively compared to control without EBR hormone. It can be seen that highest increase in number of leaves/plants, leaf area, number of fruits/plants under 50 mT MF with 3
µM hormone level, while number of flowers/plants was at 100 mT MF with 3 µM.
TABLE (9): EFFECT OF INTERACTION BETWEEN DIFFERENT MF STRENGTHS WITH DIFFERENT CONCENTRATIONS OF FOLIAR SPRAY OF 24-EPIBRASSINOLIDE ON NUMBER OF LEAVES PLANT-1, LEAF AREA, NUMBER OF FLOWERS AND NUMBER OF FRUITS PLANT1, OF TOMATO.
Treatments
number of leaves plant1
Leaf area (cm2)
Number of Flower plant1
Number of Fruits plant1
Magnetic field
Hormone (µM)
0
0
41.16
2.63
15.83
10.66
50
0.5
79.83
5.63
31.16
25.41
1
85.41
6.20
33.50
27.91
3
87.58
6.82
36.33
31.25
100
0.5
86.41
6.36
37.00
25.83
1
83.66
6.23
37.33
28.08
3
87.58
6.63
38.08
28.00
150
0.5
80.83
6.01
34.25
29.00
1
79.41
5.99
38.41
29.16
3
83.25
6.12
36.08
28.83
LSD
0.47
0.07
0.55
0.44
F
**
**
**
**
*: Means followed by the same letter are not significantly different according to RLSD at P0.05. NS: Not significant at P0.05
*: significant at P0.05**: significant at P0.01
3.10 Effect of interaction between different NaCl concentrations with magnetic field and different concentrations of foliar spray of 24-epibrassinolide on number of leaves plant-1, leaf area, number of flowers and number of fruits plant1, of tomato plants.
The results in table (10) show that application of the hormone EBR sprayed on tomato leaves resulted in high significant increases in growth parameters (number of leaves/plant, leaf area, number of flowers/plant, number of fruits/plant) under all interactions with MF strengths (50, 100, 150 mT) and salinity stresses represented in NaCl
concentrations (0, 50, 150, 250 mM). Application of the hormone and MF mitigated the negative effects caused by NaCl salinity on these studied parameters, and at the same time, these enhancement effects are gradually decreased with increase in salinity up to 250 mM NaCl. Under control the highest percentage increase in number of leaves/plants was 86.78 % at 3 µM EBR and 100 mT MF; leaf area was
131.71 % at 3 µM EBR and 50 mT MF; for flowers/plant was under 1 µM EBR hormone level and 100 and 150 mT MF (107.75 %). The highest increase in number of fruits/plant was at 3µM hormone level with 50 mT MF (218.51 %).
TABLE (10): EFFECT OF INTERACTION BETWEEN DIFFERENT NACL CONCENTRATIONS OF NACL WITH MAGNETIC FIELD AND FOLIAR SPRAY OF 24-
EPIBRASSINOLIDE ON NUMBER OF LEAVES PLANT-1, LEAF AREA, NUMBER OF FLOWERS AND NUMBER OF FRUITS PLANT1, OF TOMATO.
Treatments
number of leaves plant1
Leaf area (cm2)
Number of Flower plant1
Number of Fruits plant1
Salt concentration (mM)
Magnetic field
Hormone (µM)
0
0
0
68.66
4.13
21.66
12.33
50
0.5
110.00
7.28
38.00
33.33
1
118.00
8.42
35.66
37.33
3
126.00
9.57
39.66
39.33
100
0.5
121.00
8.69
42.00
31.33
1
115.33
7.59
45.00
34.66
3
127.33
9.47
41.00
32.66
150
0.5
91.33
6.94
37.33
34.66
1
88.00
6.97
44.00
32.33
3
95.66
7.12
40.66
34.66
50
0
0
49.00
3.16
18.66
11.33
50
0.5
77.33
5.95
37.00
29.33
1
88.00
6.25
38.66
31.00
3
81.33
6.85
40.00
34.66
100
0.5
85.33
6.73
45.00
28.33
1
80.33
6.95
43.66
32.00
3
75.66
6.15
46.00
29.66
150
0.5
91.33
6.94
37.33
34.66
1
88.00
6.97
44.00
32.33
3
95.66
7.12
40.66
34.66
150
0
0
38.66
2.13
13.00
10.33
50
0.5
68.33
5.15
30.33
21.33
1
72.33
5.51
34.66
24.00
3
78.33
5.94
37.33
28.00
100
0.5
73.66
5.45
38.00
24.33
1
77.33
5.74
35.66
21.66
3
79.66
5.92
37.33
27.33
150
0.5
78.33
5.91
37.33
24.66
1
77.33
5.76
38.66
27.33
3
73.33
5.52
38.00
24.66
250
0
0
8.33
1.12
10.00
8.66
50
0.5
63.66
4.15
19.33
17.66
1
63.33
4.64
25.00
19.33
3
64.66
4.92
28.33
23.00
100
0.5
65.66
4.56
23.00
19.33
1
61.66
4.63
25.00
24.00
3
67.66
4.98
28.00
22.33
150
0.5
62.33
4.28
25.00
22.00
1
64.33
4.25
27.00
24.66
3
68.33
4.75
25.00
21.33
LSD
0.47
0.07
0.55
0.44
F
**
**
**
**
*: Means followed by the same letter are not significantly different according to RLSD at P0.05. NS: Not significant at P0.05
*: significant at P0.05**: significant at P0.01
3.11 Effect of different concentrations of NaCl Stress, magnetic field and different concentrations of foliar spray of 24-epibrassinolide on biochemical parameters (soluble proline content, lipid peroxidation and proline content) on tomato.
Tomato plants have undergone high significant differences (P 0.01) in the biochemical parameters (Soluble Protein Content, Lipid Peroxidation and Proline content) under NaCl, MF and EBR hormone different concentrations Table (11). Soluble protein content was significantly reduced with increase in NaCl concentrations, while Lipid peroxidation and proline content were significantly increased with increase in NaCl levels (0, 50, 150, 250mM). On the other hand, application of MF and EBR hormone caused significant increases in soluble protein content, and significant reduction in lipid peroxidation and proline content under all MF strengths (50, 100, 150 mT) and hormone levels (0,0.5,1,3 µM) compared to controls. The
significantly highest reduction in soluble protein content (50.44%), and the significantly highest increase in lipid peroxidation (147.28%), and proline content (107.8%) compared to control were under the highest NaCl concentration (250 mM). Application of MF and EBR hormone significantly enhanced and increased soluble protein content, and reduced lipid peroxidation and proline content. The significantly highest increase in soluble protein content (701%) was at MF 100 mT, highest reduction in lipid peroxidation (30.09%) was at MF 150 mT, and in proline content (15.42%) was under MF 50 mT. The significantly highest increase in soluble protein content (73.88%), and the significantly highest reduction in lpid peroxidation (24.87%), and proline content (22.60%) compared to control, were under the highest application of EBR hormone at (3 µM) levels sprayed on the leaves compared to control.
TABLE (11): EFFECT OF DIFFERENT CONCENTRATIONS OF NACL STRESS, MAGNETIC FIELD AND DIFFERENT CONCENTRATIONS OF FOLIAR SPRAY OF 24- EPIBRASSINOLIDE ON BIOCHEMICAL PARAMETERS (SOLUBLE PROTEIN CONTENT (MG G-1 FW), LIPID PEROXIDATION (NMOL G1 FW) AND PROLINE CONTENT (NMOL G1 FW)) ON TOMATO.
Treatments
Soluble Protein Content (mg g-1 FW)
Lipid Peroxidation (nmol g1 FW)
Proline content (nmol g1 FW)
Salt Concentration (mM)
0
71.03 a
27.97 d
2.73 d
50
60.84 b
31.19 c
2.97 c
150
53.18 c
50.24 b
4.12 b
250
40.88 d
67.96 a
4.84 a
LSD
0.28
0.56
0.04
F
**
**
**
Magnetic Field
0
38.25 d
57.05 a
3.89 a
50
62.00 b
41.60 b
3.29 c
100
65.09 a
38.83 d
3.58 b
150
60.60 c
39.87 c
3.89 a
LSD
0.42
0.41
0.03
F
**
**
**
Hormone
0
38.25 d
57.05 a
3.89 a
0.5
61.16 c
41.88 b
3.72 b
1
62.73 b
39.54 c
3.53 c
3
63.79 a
38.88 d
3.52 c
LSD
0.29
0.26
0.019
F
**
**
3.89 a
*: Means followed by the same letter are not significantly different according to RLSD. NS: Not significant. *: significant at P0.05. **: significant at P0.01.
-
-
DISCUSSION
Results obtained on reduction of plant height, shoot and root fresh and dry weights by salinity in this research agree with other research findings. Salt stress caused significant reduction in sugar beet plant biomass (Ghoulam et al., 2002); Ajwain (Ashraf and Orooj 2006); Mentha pulegium (Oueslati et al. 2010), Cumin (Rebey et al. 2017) and in Schizonepeta tenuifolia (Zhou et al. 2018). This decrease in growth under salinity may be because salinity affects stomatal closure and ultimately photosynthetic activity is reduced (Taârit et al. 2012) and causes nutritional disorders (Läuchli and Epstein, 1990; Anuradha and Rao, 2003). Plant dry weight significantly decreased by increasing NaCl concentration (Khalid and Cai, 2011). Atak et al. (2003) applied MF on soybean and corn and found significant increases in fresh and dry weight of the seedlings. Application of MF enhanced growth and yield of wheat, barley, maize, soybean, and mung bean under abiotic stresses like salt and drought, (Hozayn et al., 2018). Martinez, et al. (2000) exposed barley seeds to a static magnetic field of 125 mT for different periods, and observed increases in lengths and weights of seedlings. Kataria et al., (2017), reported similar results. The role of MF may be it causes an increase in the absorption of water and nutrients (AlKhazan et al., 2011); or MF might send signals for protein synthesis immediately after the salt stress that protects the plasma membrane (Santoro et al., 1992). Karimi et al., (2012) found that MF adjusted osmotic process and improved water availability to plants. As for the role of EBR hormone (Dong, et al., 2017) suggested that it works on the root by increasing root activity and H+-ATPase activity. Moreover, (Khripach et al., 2000) have demonstrated that
EBR could regulate cell elongation and division by activating cell wall loosening enzymes. (López-Gómez et al., 2016) demonstrated that foliar application of 24-EBL increased plant fresh and dry biomass of Medicago truncatula under saline conditions, and in strawberry (Karlidag et al., 2011).
The results obtained regarding number of leaves/plants, leaf area, number of flowers/plant and number of fruits/plants are compatible with research findings by others. The immediate response to salt stress is a reduction in the rate of leaf area as the salt concentration increases (Wang and Nil, 2000). 80 % reduction in plant growth under high salinity is due to the reduction of leaf area (LA) and 20
% of the growth reduction is most likely due to decrease in stomatal conductance (Parida and Das, 2005). In addition, Baghel et al., (2016) found significant increase in leaves number and leaf area of soybean, and (Baghel et al., 2019) in maize when subjected to MF. After application of the hormone EBR there were significant increases in number of leaves/plant and leaf area in a number of plant species, Triticum aestivum (Shahbaz et al. 2008), Capsicum annuum (Houimli et al., 2010), Solanum melongena (Wu et al., 2012), Cajanus cajan (Dalio et al., 2011), Phaseolus vulgaris (Rady, 2011), Zea mays (Agami, 2013). Çelik et al. (2008) mentioned that MF activation comes from the fact that magnetic field affected cell division and protein synthesis in paulownia node cultures. Maheshwari, (2009) said that magnetic treatments may affect Phyto-hormone production causing increase in plant growth and cell activity. Blits and Gallagher, (1991) found reduction in the number of inflorescences due to salinity effects. Flowering is delayed in wild mustard (Sinapis arvensis) and in Arabidopsis
(Stanton et al., 2000; Achard et al., 2006) as effect of salinity. Flowering of rice is delayed by salinity, even at low NaCl concentrations (2050 mM) (Lutts et al., 1995). Qaryouti et al., (2007) and Magan et al., (2008) reported reduction in total yield of tomato at salinity. Magan et al., (2008) found reduction in tomato fruit number of 2 % with an increase of 1 dS m-1 beyond the threshold. Hajiboland et al., (2010) said that salinity effects on tomato growth and yield reduction might be due to variation in photosynthetic products translocation toward root, decrease of leaves, partial or total enclosed of stomata, direct effect of salt on photosynthesis and ion balance. EBR hormone improved activity of enzymes included in photosynthesis, thus increasing number of flowers and number of fruits/plant under salt stress (Xia et al., 2009; Cheng et al., 2015).
Number of researchers observed reduction in protein content in plants after treatment with sodium chloride, in tomato (Jaleel et al., 2008), in barley Hordeum vulgare (Khosravinejad et al., 2009) and in Vigna unguiculata (Chen et al., 2007). Arora et al., (2008), found that EBR application enhanced protein content in Zea mays seedlings under salinity stress. The promoting effects of EBRs on the protein content could be attributed to activation of transcription and translational processes of specific stress tolerance genes (Kagale e al. 2007). Belyavskaya (2004) concluded formation of new protein bands in plants treated with magnetic field. Lipid increased in plants under salinity because lipid is formed as a result of oxidative damage that takes place in the tissues of plants suffering salt stress (Campo et al., 2014). Egbichi et al., (2013) reported that salt stress induced oxidative damage to membrane lipids. Magnetic field application initiates production of radicals acting as a signal to enhance production of antioxidants and cause a dramatic increase in radical-scavenging activity, thus preventing oxidative damage due to salinity and reduces lipid (Sahebjamei et al., 2007; Çelik et al., 2009). Chen et al. (2011) reported that MF reduces lipid peroxidation. The rise in proline content in tomato under salinity is because salt stress causes a remarkable increase in free proline content in plants, because it helps in alleviating the osmotic stress upon cell under stress conditions by reducing the uptake of toxic ions, and it interacts with cellular enzymes and stabilizes their structure as well as functions (Hayat et al. 2012). The accumulation of proline in plants under salinity stress is caused either by the induction of expression of proline biosynthesis genes (P5CS, P5CR) or by the repression of the genes of its degradation pathway (PDH silencing) (Marco et al. 2015). Application of EBR on leaves resulted in rise of proline in leaves and helped plants to tolerate salt stress, as in pepper plants (Abbas et al., 2013), Cucumis sativus plants (Fariduddin et al., 2013), P. vulgaris seedlings (Rady, 2011), and in Triticum aestivum (Talaat and Shawky, 2013).
-
CONCLUSION
The results obtained in this study documented that salinity exposed negative effects on morphological, physiological and biochemical parameters of tomato plants, initiating reactive oxygen species (ROS) in the cells of the plants thus causing oxidative stress, increasing antioxidant enzyme activities. Salt stress induces osmotic stress, ionic
stress and oxidative stress and reduced tomato growth and yield. Application of Magnetic Field on tomato seeds and spray of 24-Epibrassinolide hormone on tomato leaves significantly positively affected and improved all these mentioned tomato parameters and made tomato plants more tolerant to effects of salt stress. As a result of application of MF and EBR hormone on tomato growing under salinity stress conditions, there were significant increases in plant height, shoot and root fresh and dry weight, number of leaves/plant, leaf area, number of flowers/plant, number of fruits /plant, increase in protein content in leaves, decrease in lipid and proline.
Resistance to any kind of stress closely linked to detoxifying ability of plants to counteract the ROS. There is a close link between ROS and MF treatment. Our results indicated that MF has a dual beneficial impact on ROS; MF enhanced the ROS and later reduced the ROS in mature leaves to facilitate higher electron transport, efficiency of photosynthesis, soluble portion, MDA and proline content which ultimately results in higher yield of tomato under salt stress. In addition, application of 24-Epibrassinolide hormone mitigated harsh and inhibiting effects of salt stress on tomato plants.
Thus, the present study provides the evidence that MF pretreatment of seeds and foliar spray of EBL can enhance the tomato plant salt tolerance. MF seed pretreatment can be used in agriculture to obtain better growth and increased yield under adverse abiotic stress conditions. Pre-exposure of seeds to MF has potential to increase crop production per unit area of land without having any detrimental effects toward any environmental component. In future, it may be a good tool for improving agricultural production in several economically important crops since it mitigates the adverse effects of abiotic stresses. In addition, the present study revealed the ability of brassinosterois hormone in improving the yield of tomato plants under salt conditions; it has been used extensively in agricultural uses. In Addition, foliar application of these plant growth substances may be more cost-effective as it requires relatively lower concentrations compared to other substances.
REFERENCES
-
S. Abbas, H.H. Latif, E.A. Elsherbiny. Effect of 24-Epibrassinolide on the Physiological and Genetic Changes on Two Varieties of Pepper under Salt Stress Conditions. Pak. J. Bot., 45(4): 1273-1284. 2013.
-
P. Achard, H. Cheng, L. De Grauwe, J. Decat, H. Schoutteten, T. Moritz, D. van Der Straeten, J. Peng, N.P. Harberd. Integration of Plant Responses to Environmentally Activated Phytohormonal Signals. Science, 311: 91 94. 2006.
-
R.A. Agami. Alleviating the Adverse Effects of NaCl Stress in Maize Seedlings by Pretreating Seeds with Salicylic Acid and 24- Epibrassinolide. South African Journal of Botany, 88: 171-177. 2013.
-
G.J. Ahammed, S.P. Choudhary, S. Chen, X. Xia, K. Shi, Y. Zhou, et al. Role of Brassinosteroids in Alleviation of Phenanthrene-cadmium Co-contamination-induced Photosynthetic Inhibition and Oxidative Stress in Tomato. J. Exp. Bot., 64: 199213. doi: 10.1093/jxb/ers323. 2013.
-
M. Al-Khazan, B.M. Abdullatif, N. Al-Assaf. Effects of Magnetically Treated Water on Water Status, Chlorophyll Pigments and Some Elements Content of Jojoba (Simmondsia chinensis L.) at Different Growth Stages. African Journal of Environmental Science and Technology, 5(9): 722-731. 2011.
[6] |
S. Anuradha, S.S.R. Rao. Effect of Brassinosteroids on Salinity Stress Induced Inhibition of Seed Germination and Seedling Growth |
Activities and Proline Content of Cucumis sativus under Salt and/or Copper Stress. Environmental monitoring and assessment, 185(9), |
|
of Rice (Oryza sativa L.). Plant Growth Regul., 33:151153. 2003. |
7845-7856. 2013. |
||
[7] |
N. Arora, R. Bhardwaj, P. Sharma, H.K. Arora. Effects of 28- Homobrassinolide on Growth, Lipid Peroxidation and Antioxidative |
[28] |
C. Ghoulam, A. Foursy, K. Fares. Effects of Salt Stress on Growth, Inorganic Ions and Proline Accumulation in Relation to Osmotic |
Enzyme Activities in Seedlings of Zea mays L. under Salinity Stress. |
Adjustment in Five Sugar Beet Cultivars. Environmental and |
||
[8] |
Acta Physiol Plant., 30: 833839. 2008. M. Ashraf, A. Orooj. Salt Stress Effects on Growth, Ion |
[29] |
experimental Botany, 47(1), 39-50. 2002. R. Hajiboland, A. Aliasgharzadeh, S.F. Laiegh, C. Poschenrieder. |
Accumulation and Seed Oil Concentration in an Arid Zone |
Colonization with Arbuscular Mycorrhizal Fungi Improves Salinity |
||
Traditional Medicinal Plant Ajwain (Trachyspermum ammi [L.] Sprague). Journal of Arid Environments, 64(2): 209-220. 2006. |
Tolerance of Tomato (Solanum lycopersicum L.) Plants. Plant Soil, 331: 313-327. 2010. |
||
[9] |
Ç. Atak, Ö. Emirolu, S. Alikamanolu, A. Rzakoulieva. |
[30] |
S. Hayat, Q. Hayat, M.N. Alyemeni, A.S. Wani, J. Pichtel, A. Ahmad. |
Stimulation of Regeneration by Magnetic Field in Soybean (Glycine max L. Merrill) Tissue Cultures. Journal of Cell & Molecular |
Role of Proline under Changing Environments: A Review. Plant Signal. Behav., 7 (11): 1456-1466. 2012. |
||
Biology, 2(2). 2003. |
[31] |
R.L. Heath, L. Packer. Photoperoxidation in Isolated Chloroplasts. I. |
|
[10] |
L. Baghel, S. Kataria, K.N. Guruprasad. Static Magnetic Field Treatment of Seeds Improves Carbon and Nitrogen Metabolism under |
Kinetics and Stoihiometry of Fatty Acid Peroxidation. Archives in Biochemistry and Biophysics, 125: 189198. 1968. |
|
Salinity Stress in Soybean. Bioelectromagnetics, 37: 455470. 2016. |
[32] |
S.I.M. Houimli, M. Denden, B.D. Mouhandes. Effects of 24- |
|
[11] |
L. Baghel, S. Kataria, M. Jain. Mitigation of Adverse Effects of Salt Stress on Germination, Growth, Photosynthetic Efficiency and Yield |
Epibrassinolide on Growth, Chlorophyll, Electrolyte Leakage and Proline by Pepper Plants under NaCl-Stress. Eurasia J Biosci., 4: 96 |
|
in Maize (Zea mays L.) Through Magnetopriming. Acta Agrobot., |
104. 2010. |
||
[12] |
72: 1757. 2019. L.S. Bates, R.P. Waklren, 8nd I.D. Te8re. Rapid Determination of |
[33] |
M. Hozayn, A.A. EL-Mahdy, M.T. Zalama. Magneto-Priming for Improving Germination, Seedling Attributes and Field Performance |
Free Proline Water Stress Studies. Plant Soil, 39: 205-207. 1973. |
of Barley (Hordeum Vulgare L.) under Salinity Stress. Middle East |
||
[13] |
N.A. Belyavskaya. Biological Effects Due to Weak Magnetic Field on Plants. Advances in space Research, 34(7): 1566-1574. 2004. |
[34] |
J Agric Res., 7 (3): 10061022. 2018. N. Iqbal, A. Masood, N.A. Khan. Phytohormones in Salinity |
[14] |
K.C. Blits, J.L. Gallagher. Morphological and Physiological Responses to Increased Salinity in Marsh and Dune Ecotypes of |
Tolerance: Ethylene and Gibberellins Cross Talk. In: Khan NA, Nazar R, Iqbal N, Anjum NA (eds) Phytohormones and Abiotic Stress |
|
Sporobolus virginicus (L.) Kunth. Oecologia, 87: 330 335. 1991. |
Tolerance in Plants. Springer, Berlin, pp 7798. 2012. |
||
[15] |
A.A. Boe, D.K. Salunkhe. Effects of Magnetic Fields on Tomato Ripening. Nature, 199: 9192. 1963. |
[35] |
N. Iqbal, S. Umar, N.A. Khan, M.I.R. Khan. A New Perspective of Phytohormones in Salinity Tolerance: Regulation of Proline |
[16] |
J. E. Bojrquez-Quinta, I. EchevarriaMachado, F. Medina-Lara, M. |
Metabolism. Environ Exp Bot, 100: 3442. 2014. |
|
Martfnez- Estevez (2012). Plants Challenges in a Salinized World: The Case of Capsicum. Afr. J. Biotechnol., 11: 13614-13626. doi: |
[36] |
C.A. Jaleel, K. Riadh, R. Gopi, P. Manivannan, J. Inés, H.J. Al-Juburi, C.X. Zhao, H.B. Shao, R. Panneerselvam. Antioxidant Defense |
|
10.5897/AJB12.2145. 2012. |
Responses: Physiological Plasticity in Higher Plants under Abiotic |
||
[17] |
M.M. Bradford. Rapid and Sensitive Method for Quantitation of Microgram Quantities of Protein Utilizing the Principle of Protein |
[37] |
Constraints. Acta Physiologiae Plantarum, 31: 427436. 2009. A. Jamil, S. Riaz, M. Ashraf, M.R. Foolad. Gene Expression |
dye Binding. Analytical Biochemistry, 72: 248254. 1976. |
Profiling of Plants under Salt Stress. Crit. Rev. Plant Sci., 30: 435- |
||
[18] |
S. Campo, P. Baldrich, J. Messeguer, E. Lalanne, M. Coca, B. San Segundo. Overexpression of a Calcium-dependent Protein Kinase |
[38] |
458. 2011. S. Kagale, U.K. Divi, J.E. Krochko, W.A. Keller, P. Krishna. |
Confers Salt and Drought Tolerance in Rice by Preventing Membrane |
Brassinosteroids Confers Tolerance in Arabidopsis Thaliana and |
||
[19] |
Lipid Peroxidation. Plant physiol., 165(2): 688-704. 2014. Ö. Çelik, Ç. Atak, A. Rzakulieva. Stimulation of Rapid Regeneration |
Brassica napus to a Range of Abiotic Stresses. Planta, 225: 353364. 2007. |
|
by a Magnetic Field in Paulownia Node Cultures. Journal of Central |
[39] |
S. Karimi, S. Hojati, S. Eshghi, R.N. Moghaddam, S. Jandoust. |
|
[20] |
European Agriculture, 9(2): 297-304. 2008. Ö. Çelik, N. Büyükuslu, Ç. Atak, A. Rzakoulieva. Effects of |
Magnetic Exposure Improves Tolerance of Fig Sabz Explants to Drought Stress Induced In Vitro. Scientia horticulturae, 137: 95-99. |
|
Magnetic Field on Activity of Superoxide Dismutase and Catalase |
2012. |
||
in Glycine max (L.) Merr. Roots. Pol. J. Environ. Stud., 18: 175 182. 2009. |
[40] |
H. Karlidag, E. Yildirim, M. Turan. Role of 24-Epibrassinolide in Mitigating the Adverse Effects of Salt Stress on Stomatal |
|
[21] |
Y.P. Chen, R. Li, J.M. He. Magnetic Field Can Alleviate |
Conductance, Membrane Permeability, and Leaf Water Content, Ionic |
|
Toxicological Effect Induced by Cadmium in Mungbean Seedlings. Ecotoxicolog,y 20: 760769. 2011. |
Composition in Salt Stressed Strawberry (Fragaria ananassa). Scientia Horticulturae. 130: 133140. 2011. |
||
[22] |
C. Chen, C. Tao, H. Peng, Y. Ding. Genetic Analysis of Salt Stress |
[41] |
S. Kataria, L. Baghel, K.N. Guruprasad. Alleviation of Adverse |
Responses in Asparagus Bean (Vigna unguiculata L. ssp. Sesquipedalis verdc.). J. Hered. 98 (7): 655665. 2007. |
Effects of Ambient UV Stress on Growth and Some Potential Physiological Attributes in Soybean (Glycine max) by Seed Pre- |
||
[23] |
W. Cheng, Y. Huang, C. Meng, N. Zhang, H. Zeng, J. Ren, Y. Li, Y. |
Treatment with Static Magnetic Field. J. Plant Growth Regul. 36: |
|
Sun. Effect of Exogenous 24-Epibrassinolide on Salt Resistance of Watermelon (Citrullus lanatus L.) under Salinity Stress. Paper |
[42] |
550565. 2017. K.A. Khalid, W. Cai. The Effects of Mannitol and Salinity Stresses |
|
presented at 5th International Conference on Advanced Design and |
on Growth and Biochemical Accumulations in Lemon Balm. Acta |
||
Manufacturing Engineering (ICADME), Shenzen, China, 19-20 September 2015. 2015. |
[43] |
Ecologica Sinica, 31(2): 112-120. 2011. M.I.R. Khan, N. Iqbal, A. Masood, T.S. Per, N.A. Khan. Salicylic |
|
[24] |
R.J.D. Dalio, H.P. Pinheiro, L. Sodek, C.R.B. Haddad. The Effect of |
Acid Alleviates Adverse Effects of Heat Stress on Photosynthesis |
|
24-Epibrassinolide and Clotrimazole on the Adaptation of Cajanus cajan (L.) Millsp. to Salinity. Acta Physiologiae Plantarum, 33(5): |
Through Changes in Proline Production and Ethylene Formation. doi:10.4161/psb.26374. 2013. |
||
1887-1896. 2011. |
[44] |
F. Khosravinejad, R. Heydari, T. Farboodnia. Effect of Salinity on |
|
[25] |
Y. Dong, W. Wang, G. Hu, W. Chen, Y. Zhuge, Z. Wang, M.R. He. Role of Exogenous 24-Epibrassinolide in Enhancing the Salt |
Organic Solutes Contents in Barley. Pakistan Journal of Biological Sciences, 12(2), 158. 2009. |
|
Tolerance of Wheat Seedlings. Journal of soil science and plant |
[45] |
V. Khripach, V. Zhabinskii, A. Groot. Twenty Years of |
|
[26] |
nutrition, 17(3), 554-569. 2017. I. Egbichi, M. Keyster,A. Jacobs, A. Klein, N. Ludidi. Modulation |
Brassinosteroids: Steroidal Plant Hormones Warrant Better Crops for the XXI Century. Ann. Bot., 86: 441-447. 2000. |
|
of Antioxidant Enzyme Activities and Metabolites Ratios by Nitric |
[46] |
A. Krylov, G.A. Tarakonova. Plant physiology. Fiziol. Rost., 7: 156. |
|
Oxide in Short-Term Salt Stressed Soybean Root Nodules. South African journal of botany, 88: 326-333. 2013. |
[47] |
1960. O.A. Kuznetsov, K.H. Hasenstein. Intracellular Magnetophoresis of |
|
[27] |
Q. Fariduddin, R.R. Khalil, B.A. Mir, M. Yusuf, A. Ahmad. 24- |
Amyloplasts and Induction of Root Curvature. Planta, 198: 8794. |
|
Epibrassinolide Regulates Photosynthesis, Antioxidant Enzyme |
1996. |
-
A. Läuchli, E. Epstein. Plant responses to saline and sodic conditions. Agricultural salinity assessment and management, 71: 113-137. 1990.
-
G.M. López, C.J. Hidalgo, C. Lluch, C.J.A. Herrera. 24epibrassinolide Ameliorates Salt Stress Effects in the Symbiosis Medicago Truncatula-Sinorhizobium Meliloti and Regulates the Nodulation in Cross-Talk with Polyamines. Plant Physiol Biochem., 108: 212-221. 2016.
-
S. Lutts, J.M. Kinet, J. Bouharmont. Changes in Plant Response to NaCl during Development of Rice (Oryza Sativa L.) Varieties Differing in Salinity Resistance. J. Exp. Bot., 46: 1843 1852. 1995.
-
J.J. Magan, M. Gallardo, R.B. Thompson, P. Lorenzo. Effects of Salinity on Fruit Yield and Quality of Tomato Grown in Soil-Less Culture in Greenhouses in Mediterranean Climatic Conditions. Agric. Water Manag., 95: 1041-1055. 2008.
-
B.L. Maheshwari, H.S. Grewal. Magnetic Treatment of Irrigation Water: Its Effects on Vegetable Crop Yield and Water Productivity. Agric. Water Manag., 96(8): 1229-1236. 2009.
-
F. Marco, M. Bitrián, P. Carrasco, M.V. Rajam, R. Alcázar, A.F. Tiburcio. Genetic Engineering Strategies for Abiotic Stress Tolerance in Plants. In Plant biology and biotechnology (pp. 579- 609). Springer, New Delhi. 2015.
-
E. Martinez, M.V. Carbonell, J.M. Amaya. A Static Magnetic Field of 125 Mt Stimulates the Initial Growth Stages of Barley (Hordeum vulgare L.). Electro-and magnetobiology, 19(3): 271-277. 2000.
-
R. Munns, M. Tester. Mechanism of Salinity Tolerance. Annu. Rev. Plant Biol., 59: 651681. 2008.
-
C. Mussig, G.H. Shin, T. Altmann. Brassinosteroids Promote Root Growth in Arabidopsis. Plant Physiol., 133: 1261-1271. 2003.
-
S. Oueslati, N. Karray-Bouraoui, H. Attia, M. Rabhi, R. Ksouri, M. Lachaal. Physiological and Antioxidant Responses of Mentha pulegium (Pennyroyal) to Salt Stress. Acta Physiologiae Plantarum, 32(2): 289-296. 2010.
-
A.K. Parida, A.B. Das. Salt Tolerance and Salinity Effects on Plants: A Review. Ecotoxicol. Environ. Saf., 60(3): 324349. 2005.
-
Z. Peleg, E. Blumwald. Hormone Balance and Abiotic Stress Tolerance in Crop Plants. Curr. Opin. Plant Biol., 14: 290295. 2011
-
M.M. Qaryouti, W. Qawasmi, H. Hamdan, M. Edwan. Inuence of NaCl Salinity Stress on Yield, Plant Water Uptake and Drainage Water of Tomato Grown in Soilless Culture. Acta Horticulturae 747: 539-544. 2007.
-
R. Radhakrishnan, B.D.R. Kumari. Protective Role of Pulsed Magnetic Field against Salt Stress Effects in Soybean Organ Culture. Plant Biosys., 147, 135140. 2013.
-
M.M. Rady. Effect of 24-Epibrassinolide on Growth, Yield, Antioxidant System and Cadmium Content of Bean (Phaseolus vulgaris L.) Plants under Salinity and Cadmium Stress. Sci. Hortic., 129: 232237. 2011. doi: 10.1016/j.scienta.2011.03.035
-
G.R. Rathod, A. Anand. Effect of Seed Magneto-Priming on Growth, Yield and Na/K Ratio in Wheat (Triticum aestivum L.) under Salt Stress. Indian J. Plant Physiol., 21: 1522. 2016.
-
I.B. Rebey, S. Bourgou, F.Z. Rahali, K. Msaada, R. Ksouri, B. Marzouk. Relation between Salt Tolerance and Biochemical Changes in Cumin (Cuminum cyminum L.) Seeds. Journal of Food and Drug Analysis, 25(2): 391-402. 2017.
-
J.M. Ruiz-Lozano, R. Porcel, C. Azcón, R. Aroca. Regulation by Arbuscular Mycorrhizae of the Integrated Physiological Response to Salinity in Plants, New Challenges in Physiological and Molecular Studies. J. Exp. Bot., 63: 40334044. 2012.
-
H. Sahebjamei, P. Abdolmaleki, F. Ghanati. Effects of Magnetic Field on the Antioxidant Enzyme Activities of SuspensionCultured Tobacco Cells. Bioelectromagnetics: Journal of the Bioelectromagnetics Society, The Society for Physical Regulation in Biology and Medicine, The European Bioelectromagnetics Association, 28(1): 42-47. 2007.
-
M.M. Santoro, Y. Liu, S.M. Khan, L.X. Hou, D.W. Bolen. Increased Thermal Stability of Proteins in the Presence of Naturally Occurring Osmolytes. Biochemistry, 31(23): 5278-5283. 1992.
-
A.F.H. Selim, M.F. El-Nady. Physio-Anatomical Responses of Drought Stressed Tomato Plants to Magnetic Field. Acta Astr. 69: 387396. 2011.
-
M. Shahbaz, M. Ashraf, H.U.R. Athar. Does Exogenous Application of 24-Epibrassinolide Ameliorate Salt Induced Growth Inhibition in Wheat (Triticum aestivum L.)? Plant Growth Regul., 55: 5164. 2008.
-
M.B. Shine, K.N. Guruprasad, A. Anjali. Enhancement of Germination, Growth, and Photosynthesis in Soybean by Pre- Treatment of Seeds with Magnetic Field. Bioelectromagnetics, 32: 474-484. 2011.
-
M.B. Shine, S. Kataria, K.N. Guruprasad, A. Anjali. Enhancement of Maize Seeds Germination by Magnetopriming in Perspective with Reactive Oxygen Species. Journal of Agricultural and Crop Research, 5:6676. 2017.
-
P. Shrivastava, R. Kumar. Soil Salinity: A Serious Environmental Issue and Plant Growth Promoting Bacteria as One of the Tools for Its Alleviation. Saudi J. Biol. Sci., 22: 123131. 2015.
-
M.L. Stanton, B.A. Roy, D.A. Thiede. Evolution in Stressful Environments. I. Phenotypic Variability, Phenotypic Selection, and Response to Selection in Five Distinct Environmental Stresses. Evolution, 54, 93 111. 2000.
-
M.B. Taârit, K. Msaada, K. Hosni, B. Marzouk. Physiological Changes, Phenolic Content and Antioxidant Activity of Salvia officinalis L. Grown under Saline Conditions. Journal of the Science of Food and Agriculture, 92(8): 1614-1619. 2012.
-
N.B. Talaat, B.T. Shawky. 24-Epibrassinolide Alleviates Salt- Induced Inhibition of Productivity by Increasing Nutrients and Compatible Solutes Accumulation and Enhancing Antioxidant in Wheat (Triticum aestivum L.). Acta Physiol Plant., 35: 729740. 2013.
-
S. Thomas, A. Anand, V. Chinnusamy, A. Dahuja, S. Basu. Magnetoprimingcircumvents the Effect of Salinity Stress on Germination in Chickpea Seeds. Acta Physiol. Plant., 35: 3401 3411. 2013.
-
A. Vashisth, S. Nagarajan. Exposure of Seeds to Static Magnetic Field Enhances Germination and Early Growth Characteristics in Chickpea (Cicer arietinum L.). Bioelectromagnetics: 29(7): 571 578. 2008.
-
Y. Wang, N. Nil. Changes in Chlorophyll, Ribulose Biphosphate Carboxylase Oxygenase, Glycine Betaine Content, Photosynthesis and Transpiration in Amaranthus tricolor Leaves during Salt Stress. J. Hortic. Sci. Biotechnol., 75, 623627. 2000.
-
X-X. Wu, H-D. Ding, Z-W. Zhu, S-J. Yang, D-S. Zha. Effects of 24- Epibrassinolide on Photosynthesis of Eggplant (Solanum melongena L.) Seedlings under Salt Stress. Afr. J. Biotechnol. 11: 8665-8671. 2012.
-
X.J. Xia, Y.J. Wang, Y.H. Zhou, Y. Tao, W.H. Mao, K. Shi, … J.Q. Yu. Reactive Oxygen Species are involved in Brassinosteroid- Induced Stress Tolerance in Cucumber. Plant Physiology, 150(2): 801-814. 2009.
-
Q. Ye, W. Zhu, L. Li, S. Zhang, Y. Yin, H. Ma., X. Wang.Brassinosteroids Control Male Fertility by Regulating the Expression of Key Genes involved in Arabidopsis Anther and Pollen Development. Proc. Natl. Acad. Sci. USA, 107: 61006105. 2010.
-
X.F. Yu, L. Li, J. Zola, M. Aluru, H.X. Ye, A. Foudree, H.Q. Guo, S. Anderson, S. Aluru, P. Liu, S. Rodermel, Y.H. Yin. A Brassinosteroid Transcriptional Network Revealed by Genome-Wide Identification of BES1 Target Genes in Arabidopsis thaliana. Plant J., 65: 634-646. 2011.
-
Y. Zhou, N. Tang, L. Huang, Y. Zhao, X. Tang, K. Wang. Effects of Salt Stress on Plant Growth, Antioxidant Capacity, Glandular Trichome Density, and Volatile Exudates of Schizonepeta tenuifolia Briq. International journal of molecular sciences, 19(1): 252. 2018.