
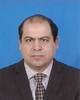
- Open Access
- Authors : M B Bughazem , Imhamed M. Saleh , Khalifa Khalifa , N. Algharbi
- Paper ID : IJERTV9IS061009
- Volume & Issue : Volume 09, Issue 06 (June 2020)
- Published (First Online): 08-07-2020
- ISSN (Online) : 2278-0181
- Publisher Name : IJERT
- License:
This work is licensed under a Creative Commons Attribution 4.0 International License
Modelling of Combined Cycle Gas Turbine Power Plant and Reduction of CO2 Emissions by using Absorption Chiller Technology
1M B Bughazem, 2Imhamed M. Saleh, 3Khalifa Khalifa, 4N. Algharbi
1234Mechanical Engineering Department, Faculty of Engineering, Sirte University Sirte, Libya
AbstractThe main purpose of this study is to propose a technical solution for all these issues (increasing demand for electric power, urgent need to reduce air pollution and global warming and the need to improve the combined cycle gas turbine power plant efficiency and prevent the effects of severe ambient conditions). The combined cycle gas turbine power plant is enhanced by utilizing the rejected heat obtained from the condenser to drive an absorption chiller to cool the gas turbine compressor inlet air to a temperature less than the ISO temperature.
The waste heat from the gas turbine exhaust gases may be utilized to produce hot water via double-pipe heat exchanger. The hot water produced could then be used to drive a single- effect lithium-bromide absorption chiller machine which in turn could be used for air conditioning and food storage requirements with cooling capacity of 1375 refrigeration tonne. This study has shown that the combined cycle gas turbine power plant integrated with absorption chiller attains output power by 10% when operated at ambient temperature of 47 °C with pre- cooling temperature of 10 °C applied to the compressor inlet air. The amount of carbon dioxide emissions reduced by the absorption chiller was 7%.
The simulation program IPSEpro has been used to do the modelling and simulation of the combined cycle in this study.
KeywordsLi-Br absorption chiller; Combined cycle power plant; IPSEpro software; Global warming.
-
INTRODUCTION
The growing demand for electric power and the rising cost of energy as well as the environmental restrictions on thermal pollution and the depletion of the ozone layer by the global warming and chlorofluorocarbon (CFC) refrigerants, together with the need for energy conservation have created an increasing interest in heat recovery technologies.
Recently, the international society has been increasingly concerned about environmental issues such as global warming and ozone layer depletion in the earth's atmosphere. Various attempts have been made, based on further utilization of waste heat rejected from conventional power cycles, to conserve the available energy sources and limit atmospheric pollution. The further use of the rejected waste heat reduces the specific fuel consumption, as the released amount of energy from a fixed amount of burnt fuel is utilized to a further significant extent, which results in saving energy and limits the amount of combustion products emitted to the atmosphere. According to the pre-mentioned concept, recently, the most successful and attractive method for the efficient use of energy is the linking of more than one
conventional power cycle. Cogeneration or CHP (combined heat and power) is the simultaneous production of electricity and heat using a single fuel source such as natural gas.
The use of CHP can reduce the fossil fuel consumption and the emissions to the atmosphere. It can result in reductions of up to 50% in CO2 emissions compared with conventional sources of heat and power as well as reduced emissions of sulphur dioxide (Cred Carbon Reduction [1]).
Maidment & Tozer [2] have studied the theoretical analysis of an application of an integrated combined cooling heat and power cycle in the supermarket. To increase the utilization time, it has been proposed that heat generated by the CHP could be used to power an absorption refrigeration system to provide cooling effect. They have initially described the cooling, heating, and power requirements of a typical supermarket and reviewed a number of combined cooling, heating, and power options with different cooling and engine technologies. Their investigation compared the energy saving and capital costs of the different options against typical conventional supermarket technology. The results indicate that this application could offer significant primary energy and CO2 saving in the short to medium term. In the longer term CCHP system will have to complete against more efficient grid generated electricity.
The most important consequence of global warming is the rise of the sea level due to glaciers and the South Pole melting. It has been predicted that there will be more extreme weather such as storms, hurricanes, floods and heat waves. The frequency and the power of this weather are going to increase and the consequence could be dramatic. The combustion of coal, oil and natural gas currently contributes about 5.5 to 6 billion metric tons of carbon per year to the atmosphere in the form of carbon dioxide (United Nations [3]).
In another work by Mohanty & Paloso [4], the option of cooling the intake air to the compressor of the gas turbine engine using absorption chiller in order to increase the gas turbine capacity was investigated. Double effect absorption chiller was powered by steam produced from a recovery boiler. An analysis carried out by taking the weather data of Bangkok indicated that reducing the temperature from ambient condition to 15°C could increase the power output up to 13%. Consequently, the plant's energy output could increase by 11%.
Ameri & Hejazi [5] have presented an overview of an intake air-cooling system that uses steam absorption chiller and an
air cooler in the Chabahar power plant. The results show the output power can be increased by 11.3%.
G. Mohan et al [6] developed and analyzed an integrated energy system based on the utilization of exhaust waste heat from an existing natural gas fired turbine power plant for the simultaneous production of electricity from a steam Rankine cycle and cooling from an absorption refrigeration cycle. The results showed that for the integrated trigeneration system, energy efficiency was about 85%, normalized CO2 emission per MWh was reduced by 51.5%. The simulation results also showed that the performance of the thermal cycles were affected by the ambient air intake temperature.
The results of the analysis presented by I. H. Njoku et al [7] showed that by utilizing the flue gas waste heat of the combined gas- and steam- turbine cycle power plant to power an absorption refrigeration system to cool the inlet air streams to 15°C in the gas turbine plants, additional 51.1 MW of electricity was generated.
M. Elberry et al [8] developed a thermodynamic model which is an integration of a (Lithium Bromide-Water) absorption inlet air cooling scheme to a cooled gas turbine-based combined cycle. The model shows an increase of 11% in the produced electricity when the inlet air was cooled from 30 °C to 10 °C.
This study and many others (Alhamzy & Najjar [9], Kakaras et al [10], Wang et al [11], Hosseini et al [12], MB Bughazem et al [13], Shazly et al [14]) report the same conclusion; that using an absorption chiller to cool the intake air of any gas turbine is economically viable, and can both enhance the plant performance and reduce its impact on the environment.
-
DESCRIPTION OF THE PROPOSED COMBINED POWER SYSTEM
The proposed model as can be seen in Figure (1), comprises of a single shaft combined cycle gas turbine power plant attached to a double-pipe heat exchanger that utilizes the exhaust waste heat of the power plant to produce hot water. The hot water produced will then be used to drive a single- effect lithium-bromide absorption chiller machine (COP = 0.83) which in turn could b used for air conditioning and food storage requirements. The combined cycle gas turbine power plant consists of a gas turbine with a capacity of 46 MW (at ISO), a single pressure level, vertical flow, and a forced circulation heat recovery steam generator (HRSG). The steam turbine has a capacity of 21 MW, and the combined cycle connected with a deaerator that controls the dissolved oxygen level in the makeup water.
The steam turbine condenser has hot stream of water with a temperature of 73 °C that drives an absorption chiller (Figure 2), which produces 1375 RT of cooling capacity at a temperature of 6 °C. Also, the chilled water stream (at a temperature of 6 °C) was circulated between the absorption chiller evaporator and the compressor air intake double-pipe heat exchanger, where it cools the inlet air of the gas turbine from 47 °C to 10 °C with a mass flow rate of 126 kg/s.
-
SIMULATED MODEL RESULTS AND DISCUSSIONS In this study the proposed power plant was modeled, tested and the results were presented at ISO conditions. Following a parametric study will be performed to evaluate the behavior of the power plant at off-design conditions, including the effect of ambient temperature on power output. Sensitivity analysis will be performed to show the effect of condenser pressure, pinch point temperature and the steam turbine isentropic efficiency on power output.
Reducing carbon dioxide CO2 emissions to the atmosphere from the generation of electricity can be obtained by improving the efficiency of existing power plants. The benefit of utilizing combined cycle and absorption chiller to reduce CO2 will be investigated.
-
The power plant behavior at ISO conditions
The first set of tests has been performed at ISO conditions (ambient temperature 15°C, relative humidity 60%, and atmospheric pressure 1.01325 bar), with seawater temperature at 25°C. The power plant at this condition was able to produce 67 MW power output with 1375 RT cooling effect for compressor inlet air and another 1375 RT cooling effect for air conditioning and food storage requirements.
Also, the previously defined performance parameters were used to evaluate the power plant performance, such as the overall thermal efficiency and the absorption chiller COP. Table (1) shows the most important readings and performance parameter results achieved after the first test at ISO conditions for all the systems within the proposed plant. These results indicate that the absorption chiller have not negatively affected after being attached to the power plant, neither has the power plant itself.
The dominant parameters for single effect absorption chillers were chosen to satisfy the gas turbine cooling and air conditioning and food storage requirements; Table (2) states the most important parameters used in this model.
128.6 47.024
128.6
116.59
Stack
1.013 45
Absorption Chiller Heat Exchanger
42 104.98
1.088 111.21
3.868
2792
Deaerator
42 318.02
1.51 25
3.868 456.75
1.5 75.944
Desorber
54.87
0.5
2.379 162.38
3.279 108.88
42 179.05
0.0619 60
128.6 186.81
1.3 42.743
2.111 2616.2
0.0619 62.219
21.41 1045.6
1.096 177.38
21.41 462.63
42.3 109.6
21.41 456.47
1.379
108.85
21.41 456.47
1.379
108.85
52.76 0.52
224.7 152.58
41.4 241.68
0.0619 63.99
1.9 36.399
128.6 283.83
HRSG
21.41 34.502
1.379 8.1877
54.87 0.5
2.111
153.88
1.103 267.41
0.0619 25.6
0.0619 36.748
171.2
1313.2
52.76 0.52 41.4 252.41
0.0619 27.43
2.111 153.88
192.1 50.602
171.2 1094.1
171.2 1093.9
21.41 2800.4
54.87 0.5
0.0619 25.6
0.008109 3.9535
2 12
Evaporator
21.41 3711.7
128.6 575.56
1.111 527.28
42.3 251.71
40.9 251.66
21.41 3711.7
40.9 251.68
Feed pump
52.76 0.52
40 616.03
40 616.03
0.008109 27.43
224.7 104.93
1 25
224.7 105.07
2 25.035
2.111 2447.1
0.008109 3.9535
224.7 129.46
1.9 30.868
Steam Turbine
21.41 2687.6
Absorber
Exhaust gases
21.41
306.99
0.37 101.8
Condenser
54.87 0.5
192.1
25.411
0.36 73.335
0.008109 25.6
1.9 6
128.6 727.22
21.41
34.327
Desorber
54.87
0.5
Natural gas fuel
2.837 31.778
1.186 636.03
0.16 8.1747
0.0619 60
30 15
2.111
2616.2
0.0619 62.219
52.76 0.52
224.7
152.58
125.8 392.66
128.6
1482.6
0.0619 63.99
1.9 36.399
14 369.61
13.9 1220
2.111
153.88
54.87 0.5
0.0619 36.748
192.1 50.602
0.0619 25.6
52.76
0.52
1.8 12.023
0.0619 27.43
2.111 153.88
192.1 50.602
54.87 0.5
0.008109 3.9535
2 12
125.8 10.385
1.003
10
125.8 10.385
1.003
10
Ambient Conditions
0.0619 25.6
52.76
0.52
125.8 48.864
Compressor
Gas Turbine
0.008109 27.43
1.013 47
224.7 104.93
224.7 105.07
2.111 2447.1
224.7 129.46
1 25
2 25.035
0.008109 3.9535
1.9 30.868
mass[kg/s] h[kJ/kg]
192.1
25.411
Chilled Water
54.87
0.5
p[bar] t[°C]
1.9 6
0.008109 25.6
Figure (1) Single-shaft combined cycle power plant connected with single effect absorption chillers modelled by IPSEpro
-
The power plant behavior at off-design conditions Combined cycle gas turbine power plants often run under off- design situations due to change of the load or ambient conditions or both. Particular attention therefore should be given to analyze the behavior of the proposed power plant used in this study.
This topic is set to investigate the proposed power plant performance under the fluctuating weather conditions i.e. ambient temperature only. The maximum and minimum values will be considered from the weather data in Libya, to ensure the power plant stability at all extreme weather conditions that may take place.
The IPSEpro process simulator [15] has been used to perform this study, while the PSExcel module has been used to create the required variation and to exchange the input values and results between the IPSEpro process simulator and MS Excel spreadsheet.
3.2.1 Ambient temperature
During the last decades, gas turbines have been widely used to produce electric power. Despite their numerous applications, one of the most important shortcomings affecting gas turbines behavior is their power output decrease at higher air ambient temperatures.
The ambient temperature fluctuation is greatly affects the gas turbine performance. Hence a parametric study have been done to monitor the gas turbine, which defines the whole power plant performance. The weather data presented in this study states that the maximum temperature expected in Libya during summer season is about 47 °C; therefore a variation from 11 °C to 47 °C will be introduced to the power plant.
The study was performed at the following conditions (relative humidity 60% and atmospheric pressure 1.01325 bar), with seawater (cooling water) temperature at 25°C and the gas turbine set at the full load. The proposed power plant results were compared with those of the power plant without cooling the gas turbine air intake, but counting all the cooling effect generated from the absorption chiller as a product.
As mentioned previously, the absorption chiller succeeded in stabilizing the compressor intake air temperature at 10 °C, which stabilized if not enhanced the performance of the whole power plant. The combined cycle gas turbine power plant with the absorption chiller presents a significant opportunity for improving the power output. The power output was increased by 10% (Figure 3).
28.64 0.51
Hot water
11
0.04 53
21.41
306.99
0.36
73.335
21.41
306.99
0.36
73.335
3
2.083
2607.2
mass[kg/s] h[kJ/kg] p[bar] t[°C]
12
21.41
33.004
Desorber
0.04 57.187
384.4
118.44
1.4
28.224
384.4
118.44
1.4
28.224
7 16
0.16 7.8589
mass (kg/s X (kg/kg)
Condenser
384.4 104.97 15
26.56 0.55 4
1.5 25
p (bar) t (°C)
0.04 60.87
Solution heat exchanger
28.64 0.51
8
2.083
121.4
0.04
28.983
2.083
121.4
0.04
28.983
26.56
0.55
0.04
29.89
2.083
121.4
0.007
1.8809
Solutiont expansio
n valv
e
26.56
0.55
0.04
29.89
2.083
121.4
0.007
1.8809
Solutiont expansio
n valv
e
Refrigerant expansion valve
0.04 25.58 2
5
9
192 50.515 17
1.1 12
28.64 0.51
0.04 25.58
26.56
0.55
6
Evaporator
18
Pump
0.007 29.89
10
192
25.322
1 6
2.083 2443.2
384.4 104.97
0.007 1.8809
13 1.5 25
Desorber inlet temperature
73
°C
Chilled water outlet temperature
6
°C
COP
0.825
—
Evaporator heat transfer
4837
kW
Cooling capacity
1375
RT
Desorber inlet temperature
73
°C
Chilled water outlet temperature
6
°C
COP
0.825
—
Evaporator heat transfer
4837
kW
Cooling capacity
1375
RT
Absorber
14 384.4 119.35
1.4 28.461
1 28.64
0.51
0.007 25.58
Figure (2) Single effect water-lithium bromide absorption chiller modelled by IPSEpro
Table (1) The proposed power plant performance parameters (at ISO conditions)
System
Parameters
Value
Unit
Combined cycle gas turbine power plant
Gas turbine power
46
MW
Steam turbine power
21
MW
Steam turbine condenser pressure
0.37
bar
Gas turbine exhaust waste energy
93.54
MW
Ambient temperature
15
°C
Natural gas heat input
141.17
MW
Cooling water temperature
25
°C
Fuel lower heating value
49767
kJ/kg
Absorption chiller
COP
0.8245
—
Cooling capacity
4837
kW
Chilled water temperature
6
°C
Desorber inlet temperature
73
°C
Desorber heat transfer
5866
kW
Electricity consumption
0.120
kW
Net power output
67
MW
Overall thermal efficiency
47
%
Energy utilization factor
54.15
%
Heat energy emitted to atmosphere
6.05
MW
CO2 emissions rate
448
kg of CO2/MW.h
Fuel mass flow rate
2.84
kg/s
Table (2) Operating conditions for a single effect water-lithium bromide absorption chiller (Figure 2)
i
Enthalpy (i) kJ/kg
Mass flow rate (i) kg/s
Pressure (i) bar
Temperature (i)
°C
Mass fraction (i) (% LiBr)
1
52.276369
28.64
0.007
25.58
51
2
52.279429
28.64
0.04
25.58
51
3
111.7
28.64
0.04
53
51
4
136.83
26.56
0.04
60.87
55
5
72.75
26.56
0.04
29.89
55
6
72.75
26.56
0.007
29.89
55
7
2607
2.083
0.04
57.19
0
8
121.40
2.083
0.04
28.98
0
9
121.40
2.083
0.007
1.88
0
10
2443.18
2.083
0.007
1.88
0
COP = 0.8245 QAbsorbe = 5.524 MW
High pressure = 0.04 bar QCondense = 5.178 MW
Low pressure = 0.007 bar QEvaporator = 4.837 MW
QDesorbe = 5.866 MW Electric power input = 0.120 kW
Figure (3) Effect of ambient temperature on power output for single shaft combined cycle gas turbine power plant with and without absorption chiller
Furthermore, the carbon dioxide CO2 emissions rate are directly influenced by the utilization of the absorption chiller
which reduces the emissions rate by 7% (Figure 4).
Figure (4) Effect of ambient temperature on CO2 emissions for single shaft combined cycle gas turbine power plant with and without absorption chiller
3.3 Sensitivity analysis of the proposed energy system
In this section, sensitivity analysis is performed. The purpose of sensitivity analysis is to evaluate whether the model appropriately responds to changes in its inputs. This is one approach to help verify the accuracy of the model (Cullen and
Frey [16]). The sensitivity analysis focuses on the effects of changes in inputs (condenser pressure, pinch point temperature and steam turbine isentropic efficiency) on the proposed energy system power output.
Figure (5) Effect of condenser pressure on power output
The condenser pressure has been varied from 0.37 bar to 0.61 bar at ISO conditions and full gas turbine load, to monitor the net power output of the proposed energy system. The results (Figure 5) indicate that lower condenser pressure boosts the net power output, which contributes to improving the overall plant efficiency as well.
A sensitivity analysis was also carried out to examine the net power output against a variation in the pinch point temperature from 14 ºC to 23 ºC at ISO conditions and the gas turbine was at full load. The results (Figure 6) show an increase in the net power output by 250 kW for each 5 ºC reduced in the pinch point temperature.
Figure (6) Effect of pinch point temperature on power output
Another sensitivity analysis was investigated on the behavior of the proposed energy system by varying the steam turbine isentropic efficiency from 63% to 87% at ISO conditions and the gas turbine was at full load. The results (Figure 7)
indicate that the isentropic efficiency has great effect on the net power output.
Figure (7) Effect of steam turbine isentropic efficiency on power output
-
-
CONCLUSION
In conclusion, global warming and improving the energy efficiency were the main motivation behind this study. An approach of solving the two problems at once was proposed by utilizing power plant exhaust heat in an absorption chiller to produce cooling effect, increase the power plants efficiency and decrease the carbon dioxide emissions to atmosphere.
A new model has been developed and constructed using simulation software called IPSEpro, by which a 67 MW combined cycle gas turbine power plant was successfully configured and attached to a single effect absorption chillers. Running and analyzing the model at off-design conditions showed that the power plant is stable, efficient and profitable producing 9674 kW of cooling effect and improving the power output by 10% and the CO2 emissions were decreased by 7%.
The most important parameter that affects the performance of a gas turbine is the intake air temperature.
The combined cycle gas turbine power plant behavior under fluctuating ambient temperature has been investigated by varying the ambient temperature from 11 °C to 47 °C with pre-cooling temperature of 10 °C applied to the compressor intake air, in order to find out the effect of extreme weather conditions on the power plant. The combined cycle gas turbine power plant after cooling the hot ambient air entering the compressor by the absorption chiller shows no reaction towards the ambient temperature fluctuation.
The absorption chiller succeeded in stabilizing the compressor intake air temperature at 10 °C and thus enhancing the performance of the whole power plant and
producing cooling effect for air conditioning and food storage requirements with a capacity of 1375 refrigeration tonne.
Absorption refrigeration cycles are attracting increasing interest because they can be driven by low-temperature heat sources, and may therefore provide a means of converting waste heat into useful refrigeration. Absorption cooling is a technology that allows cooling to be produced from heat, rather than from electricity and does not use harmful refrigerants.
REFERENCES
-
Cred Carbon Reduction, (2004), The Community Carbon Reduction Project at UNC-Chapel Hill, CRed in Cambridge- Industrial Sector.
-
Maidment, G. G., Tozer, R. M., (2002), Combined Cooling Heat and Power in Supermarket, Applied Thermal Engineering, Vol. 22, Issue 6, pp. 653-665.
-
United Nations, (1992), Climate Change and Transnational Corporations: Analysis and Trends, Centre on Transnational Corporations, ISBN 9211043859, 9789211043853
-
Mohanty, B. and Paloso, G., (1995) Enhancing Gas Turbine Performance by Intake Air Cooling Using an Absorption Chiller, Heat Recovery Systems and CHP; 15(1); pp. 41-50.
-
Ameri, M. and Hejazi S. H., (2004), The Study of Capacity Enhancement of the Chabahar Gas Turbine Installation Using an Absorption Chiller, Applied Thermal Engineering; 24, pp. 59-68.
-
Mohan, G., Dahal, S., Kumar, U., Martin, A., & Kayal, H., (2014), Development of Natural Gas Fired Combined Cycle Plant for Tri- generation of Power, Cooling and Clean Water Using Waste Heat Recovery: Techno-economic Analysis, Energies,7, 63586381.
-
I.H. Njoku, C.O.C. Oko, & J.C. Ofodu, (2018), Performance Evaluation of a Combined Cycle Power Plant Integrated with Organic Rankine Cycle and Absorption Refrigeration System, Cogent Engineering, 5:1, 1451426
-
Elberry, M., Elsayed, A., Teamah, M., Abdel-Rahman, A., Elsafty, A., (2018), Performance improvement of power plants using absorption cooling system, Alexandria Engineering Journal, 57 (4), pp. 2679-2686.
-
Alhamzy, M. M. and Najjar, Y. S., (2004), Augmentation of Gas Turbine Performance Using Air Coolers, Applied Thermal Engineering, Vol. 24, No. (2-3), pp. 415-429.
-
Kakaras, E., Doukelis, A., Prelipceanu, A. and Karellas, S., (2006), Inlet Air Cooling Methods for Gas Turbine Based Power Plants, Journal of Engineering for Gas Turbines and Power, Vo. 128, No. 2, pp. 312-317.
-
Wang, F. J., Chiou, J. S. and Wu, P. C., (2007), Economic Feasibility of Waste Heat to Power Conversion, Applied Energy, Vol. 84, No. 4, pp. 442-454.
-
Hosseini, R., Beshkani, A. and Soltani, M., (2007), Performance Improvement of Gas Turbines of Fars (Iran) Combined Cycle Power Plant by Intake Air Cooling Using a Media Evaporative Cooler, Energy Conversion and Management, Vol. 48, No. 4, pp. 1055-1064.
-
MB Bughazem and Agnew B., (2009), Improvements of the Single Shaft Combined Cycle Gas Turbine Power Plant by Intake Air Cooling Using an Absorption Chiller, Heat Powered Cycles Conference 2009, TU Berlin, 7-9 September 2009, HPC 800.
-
El-Shazly, A., Elhelw, M., Sorour, M., El-Maghlany, W., (2016),Gas Turbine Performance Enhancement via Utilizing Different Integrated Turbine Inlet Cooling Techniques, Alexandria Engineering Journal, Vol. 55, No. 3, pp. 1903-1914.
-
Sim Tech, (1991-2003), IPSEpro Process Simulator, Simulation Technology, System Version 4.0, Manual Version 4.0.001.
-
Cullen, A. C., and Frey, H. C., (1990), Probabilistic Techniques in Exposure Assessment: a Handbook for Dealing with Variability and Uncertainty in Models and Inputs, Plenum, New York.