
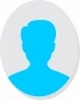
- Open Access
- Authors : Bashir Busahmin
- Paper ID : IJERTV9IS080304
- Volume & Issue : Volume 09, Issue 08 (August 2020)
- Published (First Online): 19-09-2020
- ISSN (Online) : 2278-0181
- Publisher Name : IJERT
- License:
This work is licensed under a Creative Commons Attribution 4.0 International License
Evaluation of Solution- Gas-Drive Performance in Porous Media using Artificial Oil and Crude Oil Utilizing Methane Gas System on Foamy Oil
Bashir Busahmin
Petroleum & Chemical Engineering, Universiti Teknologi Brunei, Brunei Darussalam.
Abstract: Foamy oil flow is a hypothesis used to define the movement and behaviour of gas-in-oil dispersions in porous media. It is an occurrence accompanying the initial recovery of a low viscous oil and is a commonly known reason for more production behaviour detected in different reservoirs with no mobility ratio. It is believed that the high oil viscosity and the presence of asphaltenes and other polar oil components retards the joining of gas bubbles and a great pressure decline practical in reservoir that does not require any heat and allows the molecules of a two phase to migrate without forming a continuous gas phase. However, it is not clear whether the oil viscosity or the interfacial tension might be an essential reason. In this work, an evaluation of artificial and crude oils performance at altered decline rates were investigated. Moreover, drawdown tests were conducted in the laboratory on a 2-meter long sand-pack. Both oil-gas systems were fully characterized by measuring the solution GOR, surface tension, viscosity and density of dead oils and live oils, oil compressibility and the stability of foam generated by the release of the free or dissolved gas. The outcomes demonstrated that the dissolved gas behaviour presented a similarities for both oils used. Both systems revealed a comparable depletion in the production behaviour, as to lower the pressure decline rate. It was concluded that the consistency of fluid property such as viscosity is more vital parameter in foamy oil compared to the existence or lack of n-alkane-insoluble/toluene-soluble fraction of the crude. Although the crude oil system showed marginally superior performance at lower levels of depletion, the final recovery factors were almost the same in both systems.
Keywords:- Foamy oil characteristics, methane gas, bubbles of gas, experiments, artificial oil, and crude oil.
-
INTRODUCTION
As the interest of oil escalates and because of a restricted assets of traditional oil, it is projected that the unusual assets such as bitumen and oil with high viscosity turned out to be progressively conspicuous later for oil business. A chocolate mousse oil type is a unique untraditional oil resource that was produced under gas in solution in spite of very high consistency and has been a point of exchange throughout the previous three decades. The total original volume of non- viscous oil production predicted to reach five to eight trillion barrels and that is around two-third of the global common as oil that can not be easily recovered (Alboudwarej, 2006). Various reservoir-engineering experts researched foamy oil hypothesis over many years; however, it was researched that crude oil was more viscous than refined oil (Abusahmin, B. et al., 2017). Heavy oil begins to containing scattered gas molecules and developing generally a non-stop chocolate mousse as pressure declined under a specific condition. Such chocolate mousse type oil that has more compressibility and pressure dependent properties creates framework exceptionally nonlinear. Moreover, the occurrence of wormholes sorts them hard to recover, (Lei, Xiao et al., 2013) formed a dependent time model to forecast the volumes of dissolved, entrained and developed gas in the chocolate mousse and from this initiated the compressibility of the chocolate mousse. Furthermore, dispersed drive concludes that oil comprises the least available pores, that contains higher surface area per unit volume of accessible porous rocks, and the gas will move towards bigger pores. As a result, saturation pressure is depending on ratio of the effective to absolute permeability for different phases (Bera and Babadagli, 2016; Brito et al., 2015). Largely, the drive of the 2-phase behaviour are measured by the surface interactions between molecules, which confines the dispersed flow at the boundary (Busahmin and Maini, 2019). The engineering features that is tangled in exploration and the growth of oil reservoirs globally relies on the physical features of fluid among of which compressibility density, as well as compressibility for unconventional or traditional oil. The latest research analyzed oil production behaviour for both oils, under gas in solution at various decline rates (Busahmin, B.S et al., 2016). The initial decline, (depletion) considers for a significant volume of present heavy oil recovery, even though huge fraction of hydrocarbon which has low or even very low viscosity deposits exploited by using the advanced enhanced oil recovery techniques. A few heavy oil reservoirs in e.g. Venezuela and Canada had a reasonable good initial recovery factor and that was treated as being an abnormal production trend as per (Smith, 1988). Chocolate mousse is viewed as one of the significant systems answerable for better than anticipated of the important mechanisms (Maini, 2001). Foamy oil, what so called chocolate mousse had investigated over the last couple of decades by many pioneers and few theoretical and practical models had been anticipated to describe the uncharacteristic recovery trend. Table 1 a review of some of experimental rational with the main characteristics for foamy oil behaviour. Experiments were carried out by (Xiang Zhou
et al., 2020) on the stability of a heavy oil utilizing carbon dioxide system under different pressure depletion rates and compared changes of such behaviour. Then, a comparison between heavy oilmethane system and heavy oil carbon dioxide system conducted on foamy oil stability, afterwards; a model was used to history match the stability of the heavy oil utilizing carbon dioxide system. More experimental work was investigated by (Xiang Zhou et al., 2016) with regards to foamy oil, and it was found that heavy oilpropane system was less stable than heavy oilmethane system. Moreover, pressure drawdown tests on foamy oil behaviour investigated in 1-meter-length sand-pack to examine further the influence of pressure depletion rates on various heavy oilsolvent systems. Furthermore, an extended Darcy law for two-phase flow have been studied (Maini et al., 2010) by employing the concept of saturation dependent by relative permeability, where the model was remarkably successful as long as the fluid distribution within the porous medium is controlled by the capillary forces. Several techniques were usually utilized to evaluate the dual-phase relative permeability trends, and critical gas saturation increases linearly meanwhile the relative permeability of gas decreases exponentially, as per (Rostami et al., 2010) suggested to apply Corey relations. Furthermore, (Wu et al., 2020) researched the effect of temperature related to foamy oil flow by conducting numerous decline runs, and recommended the negative effect of temperature on foamy oil. Moreover, a series of experiments and simulation work had been conducted (Xinqian Lu et al., 2018) by applying different pressure depletion rates in a one-meter long sand-pack. The numerical simulation models were built to history match the experimental runs, for oil and gas recovery information and pressure history in the sand-pack using methane saturated heavy oil system. In addition, (Olusegun Ojumoola et al., 2020) conducted an experimental work and concluded that C3H8 was a much better solvent to extract and recover the heavy oil than CO2.
The foremost goal of the current manuscript is to conduct a comparison between an artificial oil from Shell-Canada and Agentinian crude oil systems at the same conditions of primary depletion at the same reservoir (sand pack) conditions. The oil production behaviors of both mentioned oil types under solution-gas-drive mechanism at various drawdown rates were compared. The influence of gas-oil ratio and additional related factors on the performance of solution gas drive were examined in both systems. The comparison conducted by (Xiang Zhou et al., 2017) which is somewhat in lined with the current experimental studies related to pressure volume temperature, (PVT) data that presented the differences of the density of oil, oil consistency (µ), oil formation factor (Bo), compressibility, gas-oil ratio (GOR). of 0.46%, 4.5%, 0.84%, 9.05%, and 6.55% respectively. Moreover, the proposed PVT model for foamy oil provided an average error of 0.63%, 10.68%, 0.73, 16.07% and 7.07% respectively for the density for oil, oil consistency (µ), oil compressibility, oil formation factor (Bo), and gas-oil ratio (GOR). Furthermore, these results are in comparison with the lab-scale experimental runs stated by (Zhao et al., 2016).
TABLE 1. A REVIEW OF EXPRIMENTAL RATIONALE WITH THE MAIN CHACTERISTICS FOR FOAMY OIL BEHAVIOUR
Experimental rational
Characteristics
Maini (2001)
J.A. Mitchell et al. (2003)
I. Adil and B. B. Maini (2007)
Patrice A. et al. (2008)
X. Zhang. et al. (2012)
Zhangxing Chen et al. (2015)
Hailong, Z. et al. (2016)
Jian, W. et al. (2018)
Yongchao Xue et al.(2019)
-
Discussed the pros and cons of different mathematical models suggested for numerical simulation of foamy oil flow.
-
Proposed a wallpaper paste solution (carboxyl-methyl cellulose and water) and antacid mixture might be utilized to account for a number of the physical processes and the properties required understanding foamy oil.
-
Proposed in various experiments that maybe be there is a relation concerning the foam-ability of oil and the presence of high asphaltenes content.
-
Described the viscosity model for foamy oil.
-
It was considered the first-order kinetics of appearance and the release of bubbles in oil.
-
The model was history matched with the experimental work using various heavy crude oils.
-
Suggested a technique base on the hydrodynamic equation,
-
Concerning the bubble growth between the bubbles concentration distribution and the pressure distribution.
-
Described a pseudo-bubble-point model that might be utilized in determining foamy-oil fluid properties.
-
The techniques developed were utilized to apply foamy oil in the Orinoco belt, Venezuela
-
Mathematical model was history matched with the experimental work.
-
Studded the concept for foamy oil reservoir and its development.
-
Modified the properties for conventional oil, as well as the modification for foamy oil flow and considered the pseudo-bubble -point pressure model.
-
Conducted a comparison between normal oil and foamy oil related motilities, by using core flooding experiments.
-
The Improvement of the oil phase as a result of forming the foam structure was analyzed.
-
A new IPR model was suggested for foreseeing the initial oil recovery primary of foamy oil.
-
Afterwards, the five-component model was applied to mimic the progress for foamy oil.
-
-
MATERIALS AND METHODS
-
Exploratory Layout
The schematic of the exploratory arrangement appeared in Figure 1. The core has a length of two-meters along of which seven mounted gauges and liquid dissemination tops at the inlet as well as the outlet, a blender to set up the desired oil
type of interest; a safety pressure system for adjusting the pressure; hydrocarbon gatherers; an exchange container driven along a positive displacement Isco- little drive and a computer. In addition, tools utilized for information obtaining were a thermocouple, pressure controllers and a balance. The pressure gauges were placed uniformly lengthwise of 2-meters long core, utilized for screening the pressure at different position during the drawdown tests. The balance was utilized for checking the amount of oil recovery along with a designated software for tracing the pressure behavior. Moreover, thermocouple served to check the test temperature, nevertheless all the experiments were run at a room temperature. Core holder comprised of a two-meter length rounded with hollow space, which was finished with a metal, and with an inside diameter of two and a half inches, along a flexible lead-type tube that has an outside, inside diameter of one-quarter and one inches respectively, where stretching out the inner tube, shaped an annular space for pressing the core. The core structure demonstrated in Figure 1.2. The flexible lead type tube was pressed for packing the sand alongside the internal surface of the tube shaped space. 500 psi as an overburden that was kept constant for all the experimental. The recovery end of the core was associated with oil and gas devices over a safety pressure controller. The gas cylinder type was utilized to keep up the basis pressure and give a flat drawdown in the recovery side. All drawdown interval at a fixed backpressure regulator was continuously- reduced by allowing the nitrogen-gas at a steady rate utilizing a mass flow controller devise to reach the atmospheric pressure.
Figure 1: Exploratory Layout
-
Source of gas,
-
A mixer,
-
Conveyor container,
-
A Pump,
-
The core,
-
N2- Tube,
-
Pressure gauges,
-
Mass regulator,
-
Safety pressure device,
-
Container for oil production,
-
Digital Balance,
-
A cylinder for gas production,
-
A software, and
-
A Computer.
-
-
Steps for Declining Experiments
All the experimental work conducted with one core throughout the decline experiments. The drainage process took place by infusing a 2 ½ volume of oil into the core and the back-pressure-regulator was adjusted accordingly to be greater than the desired system. The goal is to displace the solution gas which was remained in the core since the former experiment. Later, almost 2 ½-pore volume of dead oil that was saturated with methane gas was filled inside the core to check whether the core is totally soaked with the desired volume of oil. The injection valve was stopped for almost one-day to permit the pressure to settle. Once the gauges become stable, the decline run begins. At the time of the decline run, the recovery end the pressure diminished at consistent value heavily influenced by the back-pressure regulator. At the time of the run, a significant factors, for example, the stabilization of pressure gauges, core temperature as well as the amount of
hydrocarbon recovered had been checked and noted by the use of a computer. Oil recovered was collected in a container and the gas recovery was gathered in a gas chamber. The recovered gas pressure was assessed by estimating the expansion in the gas vessel. The decline test then was finished when the back-pressure regulator arrived at the barometric pressure.
-
-
RESULTS AND DISCUSSIONS:
-
Artificial Oil Utilized CH4 Gas
Three decline runs were completed with artificial oil immersed CH4-gas at 550-psi and includes the back up pressure utilizing pressure decline values were 0.406 psi/min, 0.274 psi/min, and 0.021 psi/min. Entire test runs studied at a room temperature of 23 °Centigrade. The significant experimental observations were done from the quickest and the slowest decline tests as introduced below.
-
Twenty four hours Decline Run
The pressure at the recovery port declined in this type of test beginning of 550 psi and the aim was approached to the barometric pressure for the 24-hrs. Figure 2 shows the difference in pressure at the recovery end and the normal pressure in the core depicted versus time alongside the oil production. There is basically no oil recovery at the beginning of 350 minutes of the decline run whereas the pressure decreased to 370 psi. At that point, the oil recovery begins as well as the normal pressure started to deviate from the recovery port. A short period of time appeared while the normal pressure in the core stays steady or insignificantly increments and oil production proceeded to a fast rate, where the normal pressure had begun to decline at about a similar rate at the recovery end. Nevertheless, a significant contrast among normal pressure and the recovery port pressure continues as far as possible of the test. The test carried about 26.1 %.as an improvement factor. Total or cumulative recovery of hydrocarbon were appeared in Figure 3. It was clear that at first the amount of gas recovery was moderate and the amount of oil noticed to be more. At that point, the oil recovery began to back off whereas gas recovery rate raised, then stays steady from 650 minutes as far as possible of the test.
-
Sixteen Days Decline Run
-
In this kind of test, the pressure depletion rate was decreased to make the test last more than sixteen days. The rate for pressure depletion turned out to be to some degree higher following 12-days because of a sudden alteration in adjusting the mass flow regulator which was associated with backpressure controller in the gas cylinder. This does not seem to have brought about any significant change in the decline behviour. The recovery in this run was fundamentally not quite the same as the quick test. As appeared in Figure 4, the normal pressure currently firmly followed the recovery port pressure all through the test. It implies the depletion stayed small over the run. Moreover, lack of balanced out the pressure with proceeded oil recovery as found in the quick decline test. The oil recovery began at a lot greater pressure, nearby 450 psi during this run when to compare it with 370 psi in the quick decline run. More oil recovery appeared at a short period of time before the rate of gas recovery turned out to be high. The amount gas recovery stayed constant at the rest of the test. The real oil and gas recovery were appeared in Figure 5.
3.2 Crude Utilized CH4 Gas
Four primary decline runs were studied using crude oil with decline rates of 0.35 psi/min, 0.23 psi/min, 0.048 psi/min, and
0.023 psi/min. Altogether tests began with the drainage process, with live oil at initial water saturation and the experimental layout and steps showed similar behaviour with the artificial oil system. Moreover, laboratory tests obtainable by Bennion et al. (Bennion et al., 2003) satisfies with the current results, as stated that in somewhat slow decline tests, the fluid properties such as surface tension plays a significant role in the gas bubbles to be created and attached to each other as a function of time. However, for fast drawdown tests, where there was a lack of time for the trapped gas to be attached.
-
Fast Decline Test
In the fast depletion run, the pressure at the recovery end of the core decreased regularly from 525 psi to the barometric condition over a period of approximately 24 hours. Figure 9 presents the pressure and oil recovery trend for this test. The sand-pack pressure values recorded at various positions along the core length, where pressure transducer number one denotes the pressure at the inlet and pressure transducer number seven is the pressure at the outlet. Throughout the primary segment of the decline test, the pressures in various positions decrease exponentially along the pressure at the recovery port. The pressure gradient stays minor to measure, where less oil recovered at that stage. The performance was steady along the fluid phase increase under the pressure reduction. When the pressure was lowered, the volume of solution gas tends to further increase and ultimately drives to more bubble coalesces. The pressure in the production end lingers to decline linearly. In fact, a bounce in pressure occurred in the middle of the core, it was best noticeable at the inlet. The bounce occurred because of the rapid discharge of gas in solution and guided to the increase of a substantial change in pressure in the sand-pack. Further than, the movement of two phase flow out of the sand-pack was controlled by the pressure change and that worked to reduce the pressure gradient while the continued reduction of pressure at the recovery end, where acted to increase it. A short time later, during the period when the pressure at the production port stays steady close to the air pressure, the pressure slope decreases gradually for artificial and crude oils .
The oil production trend is likewise depicted in this figure. Oil recovery has begun shortly once the gas bubbles appeared and the rate of oil stayed significant during the reminder of the depletion time. However, the total oil curve showed a decreasing slope and the rate becomes very slow after the production port has reached near atmospheric pressure. The ultimate oil production rate of the test appeared to be 388 milliliters and that symbolises 26% as the ratio of the oil production versus initial oil in place.
The trend for gas production appeared in Figure 7 and cumulative oil recovery. Gas recovery rate initiated at a low value, Afterwards it escalated throughout the depletion run and later depleted fast when the production port has gotten the ending pressure. Generally, gas production data is somewhat noisy due to the disturbance created when the collection vessel had to be emptied.
Figure 6 demonstrated a typical pressure of the core along the total production. Initially the cumulative oil increases rapidly with nearly constant average pressure. During that period, pressure is maintained by rapidly evolving gas and for some time the average pressure actually increases while the oil is being produced. Subsequently, the cumulative oil curve assumes its expected shape of declining slope with declining average pressure. The gas production data for this test are also shown; after a brief period of gas production at stabilized pressure, the gas production became almost proportional to decline in the pressure.
-
Slow Decline Test
In this test-run, the pressure at the recovery end of the core had decreased from the beginning of 550 psia to near barometric against time interval of 16 days. Figure 10 presented the behaviour and recorded the history of the test. Only the recovery port pressure as well as the shut-in end pressure are shown for clarity. The pressure values at all locations were almost the same in this test as is evident from the two pressure profiles shown in the figure. No oil recovery for the initial period of 4-days when the core pressure declined to almost 420 psig. At that period, the gas bubbles appeared and oil recovery started. There is just a hint of pressure difference between the inlet and the out let of the core instantly when the bubbles of gas appeared. Gas-oil-ratio basically affected by the creation of the bubble of gas, and the nucleation approach was of the abrupt nature (Tang and Firozabad, 2005). This quickly fades and during the rest of depletion, there is very little pressure difference between the two ends. A small pressure difference re-appears near the end and it again results in increased rate of oil production. The total oil production seemed to be 240 milliliters, and that represented a production rate of 16%.
Figure 11, presented the usual pressure against the total recovery. There was a short-term period of rapid oil recovery by lowering the pressure which lasted nearly 390-psia, and then oil rate started to become low. In addition, the trend for gas recovery remained linear with the declining pressure.
-
Comparison of Crude oil and Artificial Oil Decline Tests
It is interesting to compare the performance of solution gas drive in the crude oil system with that observed in the mineral oil tests. A comparison of oil production depicted vs. the regular core pressure as in Figure 12 for fast depletions. Recovery factor is used here to account for the difference in original oil in place in the two systems. Crude oil system presented marginally superior performance at lower levels of depletion but the final recovery factors are almost the same in both systems. Therefore, it can be concluded that the presence of asphaltenes and other polar components in the crude oil does not affect the performance in high rate depletions.
The performance in slow depletion tests were compared in Figure 13. Here also the differences do not appear to be significant. The final recovery factors are very similar. Therefore, it can be concluded that the polar crude oil components did not provide any significant benefit in solution gas drive recovery, at least in these two systems. The crude oil systems shows marginally superior performance at lower levels of depletion but the final recovery factors are almost the same in both systems. Therefore, it can be concluded that the presence of asphaltenes and other polar components in the crude oil does not affect the performance in high rate depletions. In addition as was reported by Kumar et al. (Kumar et al., 2002) that heavy oil gas drive mechanism is described by the critical gas saturation as the fact until which gas does not move as a separate phase and where discontinuous or free gas has begun to flow.
TABLE 2. PROPERTY OF THE CORE
Factor
Quantity
Original hydrocarbon in place (m3)
1490.59
Cross-Sectional Area (m2)
2.25 x 10-03
Porosity (%)
36
Overburden pressure (kPa)
6894.76
Size of the grain
30 – 50
Connate water saturation (%)
8
Length (m)
2
Pore volume (m3)
1620
Permeability of the rock in meters square
2.37 x 10-11
Fluid permeability in meters square
2.27 x 10-11
TABLE 3. PROPERTIES FOR ARTIFICIAL/REFINED AND CRUDE OILS
Factor
Refined oil
Crude oil
Refined oil
+
CH4-gas
Crude oil
+
CH4-gas
Solution GOR(cc/cc)
–
–
9.1
10
Surface Tension (dyne/cm)
31
29
28
20
Density (kg/m3) @ 23°C
896
936
891
928
Liquid Phase Compressibility (psi-1 )
6.80 x 10-6
6.90 x 10-6
4.52 x 10-6
4.37 x 10-6
Viscosity @ 23°C (mPa.s)
1583
2608
1080
1300
Saturation Pressure (psi)
N/A
N/A
500
500
-
-
CONCLUSION
The conclusions extracted from the initial depletion tests were shown that the level of critical super-saturation which was created at the time of the drawdown test depended on the depletion rate, and the relationship was a direct proportional because it increased when the decline rate increased. Under comparable experimental runs, the total hydrocarbon recovery factors investigated with artificial and crude oils were practically equivalent. Moreover both refined and unrefined oil systems demonstrated similar reduction in the oil production performance as to lower the quantity of rate of decline pressure. .It was inferred that the oil viscosity is a progressively significant factor in foamy or chocolate mousse contrasted to the appearance or absence of asphaltenes. Furthermore, it was discovered that crude oil is more viscous than artificial oil and the production behaviour in both oil systems, relied intensely on the rate of decline, which acted as the dynamic energy for the oil recovery which was progressed in the system due to the fluid withdrawal at the recovery outlet.
FIGURE 2. CORE DIAGRAM AT THE LONGITUDINAL VIEW (US. PATENT NUMBER: 5,719,327)
Prod End Pressure psi Avg P RF%
Prod End Pressure psi Avg P RF%
600 30
500 25
Pressure (psi)
Pressure (psi)
Recovery Factor (%)
Recovery Factor (%)
400 20
300 15
200 10
100 5
0 0
0 200 400 600 800 1000 1200 1400
Time (min)
FIGURE 3 RECOVERY FACTOR AND CORE PRESSURE FOR 1300 MINUTES DECLINE IN ARTIFICIAL /REFINED OIL USING CH4-GAS
Cum Gas (Std. cc) Oil Produced (mL)
Cum Gas (Std. cc) Oil Produced (mL)
18000 450
16000
Cumulative Gas (Std. cc)
Cumulative Gas (Std. cc)
14000
12000
10000
8000
6000
4000
2000
0
400
Cumulative Oil Production (mL)
Cumulative Oil Production (mL)
350
300
250
200
150
100
50
0
0 200 400 600 800 1000 1200 1400
Time (min)
FIGURE 4. CUMULATIVE OIL AND CUMULATIVE GAS PRODUCTION FOR 1300 MINUTES DECLINE IN ARTIFICIAL /REFINED OIL USING CH4-GAS
Prod End Pressure psi Avg P RF%
Prod End Pressure psi Avg P RF%
600 14
500
Pressure (psi)
Pressure (psi)
400
300
200
100
0
12
Recovery Factor (%)
Recovery Factor (%)
10
8
6
4
2
0
0 5000 10000 15000 20000 25000
Time (min)
FIGURE 5. RECOVERY FACTOR AND PRESSURE FOR 25000 MINUTES DECLINE IN ARTIFICIAL /REFINED OIL USING CH4-GAS
Cum Gas (Std. cc) Oil Produced (mL)
Cum Gas (Std. cc) Oil Produced (mL)
240 16000
Cumulative Oil Production (mL)
Cumulative Oil Production (mL)
14000
180
120
60
12000
Cumulative Gas (Std. cc)
Cumulative Gas (Std. cc)
10000
8000
6000
4000
2000
0
500.0
400.0
300.0
200.0
100.0
0
0.0
Average Sand-Pack Pressure (psi)
FIGURE 6. CUMULATIVE OIL, CUMULATIVE GAS PRODUCTION VERSUS AVERAGE PRESSURE DECLINE IN ARTIFICIAL /REFINED OIL USING CH4-
GAS
10000
Cumulative Oil Cumulative Gas
400
Cumulative Gas Production, scc
Cumulative Gas Production, scc
8750
7500
6250
5000
3750
2500
1250
350
Cumulative Oil Production, mL
Cumulative Oil Production, mL
300
250
200
150
100
50
0
500.0
400.0
300.0
200.0
100.0
0
0.0
Average Sand-Pack Pressure, psi
FIGURE 7. CUMULATIVE OIL AND CUMULATIVE GAS VERSUS AVERAGE PRESSURE IN FAST DEPLETION TEST WITH CRUDE OIL USING CH4-GAS
PT1 PT2 PT3 PT4 PT5 PT6 PT7 Oil Production
600
500
Pressure,psig
Pressure,psig
400
300
200
100
0
0 2 4 6 8 10 12 14 16 18 20 22 24
Time, hours
450
400
Cumulative oil,mL
Cumulative oil,mL
350
300
250
200
150
100
50
0
FIGURE 8. PRESSURE AND PRODUCTION HISTORY OF THE FAST DEPLETION TEST WITH CRUDE OIL USING CH4-GAS
Production end Shut-in end Cumulative Oil Production, mL
600
500
Pressure, psig
Pressure, psig
400
300
200
100
0
0 2 4 6 8 10 12 14 16 18
Time, days
300
Cumulative Oil, mL
Cumulative Oil, mL
250
200
150
100
50
0
FIGURE 9. PRESSURE AND OIL PRODUCTION HISTORY OF THE SLOW DEPLETION TEST WITH CRUDE OIL – CH4-GAS
Cum Gas (Std. cc) Oil Produced (mL)
Cum Gas (Std. cc) Oil Produced (mL)
300 12000
Cumulative Oil Production, mL
Cumulative Oil Production, mL
250
200
150
100
50
10000
Cumulative Gas, Std. cc
Cumulative Gas, Std. cc
8000
6000
4000
2000
0
500
450
400
350
300
250
200
150
0
100 50 0
Average Pressure in Sand-pack, psig
FIGURE 10. OIL AND GAS PRODUCTION VERSUS AVERAGE PRESSURE IN SLOW DEPLETION TEST WITH CRUDE OIL METHANE SYSTEM
Crude Oil @0.434 psi/min Mineral Oil @0.406 psi/min "Crude Oil @0.226 psi/min"
Crude Oil @0.434 psi/min Mineral Oil @0.406 psi/min "Crude Oil @0.226 psi/min"
30 30
Recovery Factor, %
Recovery Factor, %
Recovery Factor, %
Recovery Factor, %
25 25
20 20
15 15
10 10
5 5
0
500
400
300
200
0
100 0
Average Pressure in Sand-Pack, psi
FIGURE 11. COMPARISON OF SOLUTION GAS DRIVE PERFORMANCE IN CRUDE OIL AND MINERAL OIL SYSTEMS IN FAST DEPLETION TESTS
Crude Oil @0.023 psi/min Mineral Oil @0.021 psi/min
Crude Oil @0.023 psi/min Mineral Oil @0.021 psi/min
14 14
12 12
Recovery Factor, %
Recovery Factor, %
Recovery Factor, %
Recovery Factor, %
10 10
8 8
6 6
4 4
2 2
0
500
400
300
200
0
100 0
Average Pressure in Sand-Pack, psi
FIGURE 12. COMPARISON OF SOLUTION GAS DRIVE PERFORMANCE IN CRUDE OIL AND MINERAL OIL SYSTEMS IN SLOW DEPLET ION TESTS
FIGURE 13. REFINED MINERAL OIL AND CRUDE OIL VISCOSITIES
FIGURE 14: CARBON DIOXIDE EXPERIMENTS
ACKNOWLEDGMENT
In this research paper, funding was not required; however, we would like to thank Shell Canada for providing the oil samples to conduct the experimental work as well as to Argintinian Company (Grembik oil) for providing some of their crude oil.
REFERENCES
-
Abusahmin, B, B.B, Maini and R.Karri., 2017. Influence of Fluids and Operating Paramters on the Recovery Factors and the gas oil ratio in high viscous Reservoirs under Foamy solution gas. Fuel 197 497-517.
-
Alboudwarej, H ., 2006. Highlighting Heavy Oil," Oilfield Review 18, No.2.
-
Adriana, Brito, Ramon, C, Nolides, G, Luis, M,Jose, M, and Jorge, T., 2015., Hydrodynamic study of multiphase flow transport of highly viscous foamy fluids. Journal of Petroleum Science and Engineering, 135: 367-374.
-
Bera, A. and Babadagli, T., 2016. Relative Permeability of Foamy Oil for Different Types of Dissolved Gases. SPE Reservoir Eval. Eng., 19(04): 604-619.
-
Bennion, D., Mastmann, M. and Moustakis, M., 2003. A case study of foamy oil recovery in the Patos-Marinza reservoir, Driza Sand, Albania. Journal of Canadian Petroleum Technology, 42(03).
-
Brij M. Maini ., 2001, Foamy-Oil Flow, Journal of Petroleum Technology 53(10):54-64, DOI: 10.2118/68885-MS.
-
Busahmin, B.S., Maini, B.B., Sabet, M., and R.Karri .,2016. Studies on the stability of the foamy oil in developing heavy oil reservoirs. conference proceedings in 12th international conference on diffusion in solids and liquids, Split, Croatia.
-
Busahmin, B. and Maini, B.B., 2019. Measurements of for Mineral and Crude Oil Systems. Defect Diffusion Forum, 391: 106-113.
-
Hailong, Zhao, Yuwen Chang, and Xiaofei Guo., 2016. Foamy oil properties and reservoir performance, Petroleum Science and Technology 34(16):1512-1519, DOI: 10.1080/10916466.2016.1206570.
-
J.A. Mitchel, l, Winston A., Mellowes, and Richard A. Dawe., 2003. A Water-Based Analogue Model for Foamy Oil Experiments, Journal of Canadian Petroleum Technology 42(3), DOI: 10.2118/03-03-TN2.
-
I. Adil and B. B. Maini., 2007. Role of Asphaltenes in Foamy Oil Flow, Journal of Canadian Petroleum Technology 46(4) DOI: 10.2118/07-04- 01.
-
Jian Wang, Bo Kang, Liehui Zhang, Yulong Zhao, and Danling Wang., 2018. Experimental Investigation of Rheological Properties of Foamy Oil, Chemistry and Technology of Fuels and Oils 54(10):1-9 DOI: 10.1007/s10553-018-0948-1
-
Kumar, R., Pooladi-Darvish, M. and Okazawa, T., 2002. Effect of depletion rate on gas mobility and solution gas drive in heavy oil. SPE Journal, 7(02): 213-220.
-
Lei, Xiao, Gang and Zhao., 2013. Integrated Study of Foamy Oil Flow and Wormhole Structure in CHOPS through Transient Pressure Analysis. Heavy Oil Conference, Calgary, Alberta, Canada SPE 165538-MS.
-
Maini, B.B. and Busahmin, B., 2010. Foamy oil flow and its role in heavy oil production, AIP Conf. Proc. American Institute of Physics, pp. 103-108.
-
Olusegun Ojumoola, Hongze Ma, and Yongan Gu., 2020. Solvent-assisted water pressure cycling (SA-WPC) process in a thin heavy oil reservoir. Fuel Volume 270, 15 June 2020, 117195.
-
Patrice A. , I. Hénaut, Jean-Francois A., and M. Moan., 2008. Viscosity Behavior of Foamy Oil: Experimental Study and Modeling. Petroleum Science and Technology 26(13):1545-1558, DOI: 10.1080/10916460701208850.
-
Rostami, B., Kharrat, R., Ghotbi, C. and Tabatabaie, S., 2010. Gas-oil relative permeability and residual oil saturation as related to displacement instability and dimensionless numbers. Oil Gas Science Technology, 65(2): 299-313.
-
Smith, G. E., Fluid Flow and Sand Production in Heavy-Oil Reservoirs Under Solution-Gas Drive, SPE Production Engineering, Volume 3, No. 2, 169-180, 1988.
-
Tang, G.Q. and Firoozabadi, A., 2005. Effect of GOR, Temperature, and Initial Water Saturation on Solution-Gas Drive in Heavy-Oil Reservoirs. SPE Journal, 10(01): 34-43.
-
Xinqian Lu, Xiang Zhou, Jianxin Luo, and Xiaolong Peng., 2018. Characterization of foamy oil and gas/oil two-phase flow in porous media for a heavy oil/methane system. Journal of Energy Resources Technology 141(3):032801-12, DOI: 10.1115/1.4041662.
-
Xiang Z. et al., 2020. Experimental and mathematical modeling studies on foamy oil stability using a heavy oilCO2 system under reservoir conditions. Fuel 264, 116771.
-
Xiaofie Sun. et al., 2017. Study on equilibrium and non-equilibrium PVT behavior of foamy oil using experiments and PVT correlations. Journal of petroleum science and engineering 156 DOI: 10.1016/j.petrol.2017.06.041.
-
Xiang Z. et al., 2016. Foamy oil flow in heavy oilsolvent systems tested by pressure depletion in a sandpack. Fuel 171, 210-223.
-
Wu, M., Lu, X., Yang, J., Lin, Z. and Zeng, F., 2020. Experimental analysis of optimal viscosity for optimizing foamy oil behavior in the porous media. Fuel, 262: 116602.
-
Zhangxing Chen, Jian Sun, Ruihe Wang, and Xiaodong Wu. 2015. A Pseudobubblepoint Model and Its Simulation for Foamy Oil in Porous Media, SPE Journal 20(02):239-247, DOI: 10.2118/172345-PA.
-
X. Zhang, X. Wu, J. Zhang, and K. Liu., 2012. A New Modeling Approach for Bubble Growth in Foamy Oil, Petroleum Science and Technology 30(14):1498-1507, DOI: 10.1080/10916466.2011.644015.
-
Yongchao Xue, Du Mengge, Xingmin Li, and Meng Cao, 2019. Study on foamy oil development effect in horizontal wells based on a novel inflow performance relationship model and a five-component model, Journal of Renewable and Sustainable Energy 11(1):014102, DOI: 10.1063/1.5047263.
-
Zhou, X., Zeng, F., Zhang, L. and Wang, H., 2016. Foamy oil flow in heavy oilsolvent systems tested by pressure depletion in a sandpack. Fuel, 171: 210-223.