
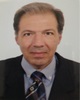
- Open Access
- Authors : Fatsis Antonios
- Paper ID : IJERTV9IS120234
- Volume & Issue : Volume 09, Issue 12 (December 2020)
- Published (First Online): 04-01-2021
- ISSN (Online) : 2278-0181
- Publisher Name : IJERT
- License:
This work is licensed under a Creative Commons Attribution 4.0 International License
Topping of Marine Gas Turbines using Four-Port Wave Rotors
Fatsis Antonios
General Department
National and Kapodistrian University of Athens Evripus Complex, 34400 Psachna, Greece
AbstractPropulsion power plants of sea going ships have to produce power at low fuel consumption, but they are subsequent to emission legislative constrains. Marine two-shaft aero- derivative gas turbines are used either as exclusive power plants, or, in combined cycles with steam turbines to provide either mechanical propulsion or to generate electricity, which is subsequently used by electric drives to propel the ship. However, thermal efficiencies of gas turbines are lower than those of Diesel engines of similar power. In the context of improving the performance of existing two-shaft marine gas turbines, it is proposed to enhance engines performance by integrating a pressure wave supercharger (or wave rotor), while keeping the components of the baseline engine unchanged. Cycle analysis is performed at design point for the baseline engines, as well as for the topped with four-port wave rotor engines. Important benefits are obtained for four-port wave rotor-topped engines including recuperator in comparison to the self-standing baseline engines for the whole range of engines operation. It is found that the lower the compressor pressure ratio and the higher the turbine inlet temperature is, the more the benefit gain of the wave rotor topped engine is attained in terms of thermal efficiency and specific power.
KeywordsMarine gas turbine, wave rotor, thermal efficiency, specific power, recuparator
-
INTRODUCTION
Greenhouse gas emissions (GHG) are considered primary source for an incontestable trend in global warming. Thus, pollution control is strongly recommended and lately established by law. NOx and SOx emissions produced by seagoing ships are of great concern in recent years. The International Maritime Organization (IMO) has imposed strict emission limitations by putting in force MARPOL regulations [1]. In a study of three alternative propulsion systems done in [2], it was concluded that gas turbine engines (GTEs) satisfy the IMO requirements and reduce significantly the machine room space and weight required with respect to the rest of the configurations examined, but additional efficiency improvement methods have to be incorporated in order to increase the relatively low efficiency of GTEs. Additional to the advantages of GTEs, the choice of LNG as fuel contributes substantially to the reduction of CO2 emissions. Moreover, the GTE combustion technology, with steady-state and controlled flame temperature allows significant reduction in NOx emissions, easily matching nitrogen regulations for current marine engines, [3].
Development in gas turbine technology during the last years, made possible for a simple gas turbine cycle to attain levels of thermal efficiency of the order of 37%. Marine gas turbines have been almost exclusively developed by aero engines. High fuel prices dictate the
need for efficiency improvement methods resulting in major savings on fuel consumption and life cycle costs. One of the methods suggested to increase the thermal efficiency is to integrate to the baseline engine intercooling and regeneration (ICR). The predicted efficiency improvement is of the order of 20%, [4].
Another method is to use combined cycles for ships: A first category is combines gas and steam turbine (COGAS) where the shafts of the turbines drive directly the propeller, and a second one is the combined gas turbine and steam turbine integrated electric generator (COGES). At full load the COGAS configuration offers higher efficiency as the transmission efficiency is higher. With a COGES configuration, it is easier to achieve rational part-load performance, because one or several power units can be shut off as the power is reduced while the remaining power units are operated at power conditions where they are efficient, [5].
A genuine method proposed in this article for efficiency improvement with simultaneous power increase is the integration of a four-port wave rotor into a marine gas turbine.
A wave rotor, Fig. 1, is composed of a purely cylindrical rotor inside a casing. Circumferentially equidistant axial straight blades are formed between these cylinders. Two stationary endwall plates with perforated circumferential openings are mounted at the rotor extremities, allowing only partial inflow and outflow through the rotor blade channels, as described in [6] and [7].
Fig.1. Four-port wave rotor schematic configuration
Depending of the number of openings (or ports), wave rotors can be classified as three-port, four-port or five-port configurations. Figure 1 illustrates a four-port wave rotor configuration, which is the one best suited as a gas turbine topping device, [8]. The rotor is connected via a ducting system to the compressor, turbine and combustion chamber of the baseline engine. The principle of operation of wave rotors is based on propagation and interaction of unsteady pressure waves inside the channels formed by two consecutive purely radial blades of the rotor. These moving pressure waves are formed inside the wave
rotor channels when a high enthalpy gas stream (e.g. hot combustion gas) is coming in contact for a short time so that mixing is avoided- with low enthalpy gas (e.g. compressed air) being inside the rotor. According to the basic theory of gas dynamics in [6], the propagation of a compression wave inside each of the rotor channels results to the
and Tg are the average temperatures during the compression and expansion processes in the compressor and turbine respectively.
For air at low temperature range of 200 to 800 K:
C 1018.9 0.13784T 1.9843104 T 2 4.2399 107 T 3
formation of an expansion wave and its propagation at the opposite direction. The contact discontinuity between the high and the low enthalpy gas streams guarantees that no mixing between the two
pa a
a
a
3.76321010 T 4
a a
(1)
streams will occur. When a wave rotor is integrated in a gas turbine, extra compression in the air flow is achieved by means of compression waves formed inside the wave rotor channels when hot
For air at high temperature range of 800 to 2200 K:
C 798.65 0.5339T 2.2882104 T 2 3.7421108T 3
exhaust gases coming out of the combustion chamber come in contact with air from the compressor. Simultaneously expansion is achieved when expansion waves are directed at the outflow port towards the turbine. An efficient design of wave rotors is attained when the pressure waves inside the rotor result in uniform and steady flows at the outflow ports, so that the air flow towards the combustion
pa a a
For specific heats of products of combustion:
C C f BT
pg pa 1 f
a
(2)
(3)
chamber and the gas flow towards the turbine are uniform. For the case of the four-port through flow configuration, the rotor blades are self-cooled because hot gas and compressed air are traversing the rotor, cooling the blade material. Feasibility studies of integrating a wave rotor to aircraft gas turbines, [9], predicted reduction of the specific fuel consumption and increase of the specific thrust delivered by the engine. Recently, the possibility of integrating a four-port wave rotor also to industrial gas turbines was also explored [10].
This article is an original study on wave rotor technology applied to marine gas turbines used either as the unique propulsion system, or in combined cycles for propulsio. Performance assessment is
where f is the fuel-to-air ratio, BT at low temperature range of 200 to 800K is given by:
g g g
g g g
BT 359.494 4.5164T 2.8116 103T 2 2.1709 105 T 3
g g
g g
2.8689108 T 4 1.2261011T 5
(4)
and BT at high temperature range of 800 to 2200 K is given by:
BT 1088.8 0.1416T 1.916103T 2 1.2401106 T 3
performed for two-shaft gas turbines with and without recuperator at design point conditions by means of the thermodynamic model developed. It is concluded that integration of wave a rotor to a marine gas turbine improves the thermal efficiency of the engine and at the
g g g
g g
g g
3.06691010 T 4 2061171014 T 5
(5)
same time increases its specific power. The improvement is more remarkable for engines operating with low compressor pressure ratios
Constant values are assumed for the air and for the exhaust gases as:
1.4 and 1.333. Table 1 summarizes typical values of input
(rc) and high Turbine Inlet Temperatures (TIT), for the range of c h
compressor pressure ratios examined.
-
TWO-SHAFT GAS TURBINE CALCULATIONS
-
Input data for two-shaft gas turbines
The procedure of the thermodynamic calculations of two-shaft gas turbine cycles with the integration of a four-port wave rotor is described in detail in [11]. It is based on standard thermodynamic analysis of gas turbines, as presented in [12], [13], adding the compression and expansion processes inside the wave rotor. Figure 2 illustrates the configurations for the wave rotor-topped two-shaft gas turbines used in this article.
Fig. 2 Two-shaft gas turbine configurations, C: compressor, T: turbine, CC: combustion chamber, WR: wave rotor, CT: compressor turbine, PT: power turbine
Thermodynamic properties of combustion gases and air at various stages throughout the gas turbine cycle are calculated by considering variation of temperature according [14]. In the equations below Ta
data used, Turbine Inlet Temperature, (TIT) and the compressor pressure ratio rc .
TABLE 1. Baseline Engine typical Input Data
Quantity
Symbol, Unit
Value
Ambient pressure
Pa , kPa
101.3
Ambient temperature
Ta , K
288
Intake pressure losses
Pin %
1
Compressor pressure ratio
rc
5 30
Combustion chamber pressure losses
Pcc %
5
Fuel Low Calorific Value
FCV, MJ/kg
42.8
Turbine Inlet Temperature
TIT, K
1000 1600
Isentropic compressor efficiency
isc
0.85
Combustion chamber efficiency
cc
0.99
Isentropic turbine efficiency
ist
0.90
-
Input data for the wave rotor
Typical input data for wave rotor thermodynamic calculations are shown in Table 2.
TABLE 2. Wave Rotor Typical Input Data
-
Performance
Symbol, Unit
Quantity
Value
PR
Wave rotor pressure ratio
1.4 2.2
Pduct %
Ducting and leakage losses
8 16
nC
Efficiency of compression processes inside the wave rotor
0.75 0.92
nE
Efficiency of expansion processes inside the wave rotor
0.75 0.92
Symbol, Unit
Quantity
Value
PR
Wave rotor pressure ratio
1.4 2.2
Pduct %
Ducting and leakage losses
8 16
nC
Efficiency of compression processes inside the wave rotor
0.75 0.92
nE
Efficiency of expansion processes inside the wave rotor
0.75 0.92
The Power consumed by the compressor, WC
WC mair Cpc (T03 T02 )
(3)
Figure 3 illustrates the model developed to calculate the thermodynamic properties of air and hot gases when a four-port wave rotor is integrated to a two-shaft gas turbine. The four-port
Power produced by the gas turbine compressor
WT WC
Heat added by the fuel Qin
WT is used to drive the
(4)
configuration has been proposed by many researchers, [7], [9], [11] because it can be easily integrated to an existing gas turbine and it offers important performance enhancement of the baseline engine.
Qin mf FCV
Power produced by the power turbine, WPT
WPT mair mf Cph (T07 T08 )
Net power delivered by the engine, WN
WN WPT
(5)
(6)
(7)
Fig. 3. Symbols used for the four-port wave rotor thermodynamic calculations
In the four-port configuration, schematically shown in Fig. 3, when the hot exhaust gases from the combustion chamber exit enter the wave rotor from the hot port 4.2, they come in contact with the compressed air from the compressor which is already inside the rotor
Specific power ws
Thermal efficiencyth
ws WN
mair
WN
(8)
(9)
through the cold port 4.0 and has filled the space between the rotor blades. These two streams (i.e. the cold air and the hot exhaust gases) are brought into contact inside the rotor, [6]. As a result, a compression wave is initiated which compresses further the air stream; the compression wave is propagating along the rotor,
th
Specific fuel consumption, sfc
QIN
reflected on solid walls directed via the port 4.1 towards the combustion chamber. Simultaneously, an expansion wave is formed due to the contact of the streams of cold air and the hot gases. It is propagating to the opposite direction (with respect to the
sfc
th
1
-
FCV
(10)
compression wave), reflected on solid walls directed towards the turbine through the port 4.3. The location of the inlet and outlet ports of the rotor depends on the unsteady wave interaction inside the rotor (called wave diagram) and its rotational speed, which is about one third the rotational speed of the high pressure turbine shaft.
The wave rotor pressure ratio is a very important parameter that characterizes the performance of the wave rotor and accordingly the performance of the whole gas turbine. It is defined as:
The allowable temperature of metal used to manufacture the blades is
approximately 800 – 900oC, but the allowable surface temperature of blades has been increased up to 1000oC due to the recent application of thermal barrier coatings on blade surfaces, [15]. Thus, in the simulations, the surface temperature of the blades is set to be constant at 1000oC. The present thermodynamic model accounts for cooling
the turbine in case TIT 1000oC by subtracting air flow from the high
pressure outflow port of the wave rotor. The coolant flow rate is determined by considering various operating parameters such as the
PR P4.1
P4.0
(1)
operating temperature of the blades and the temperatures and specific heats of the main gas and coolant. The coolant flow rate at the design
point is calculated using an energy balance equation based on the inlet air flow rate, power output, efficiency, gas turbine outlet
The pressure at te wave rotor outflow towards the turbine is given
according to [8] by the following relation known as the wave rotor characteristic.
temperature, and TIT according to the method proposed in [15], [16]. Turbine blades are made of nickel or rhenium alloys capable of withstanding high heat without distortion. The High Pressure Turbine
C 1 n T
c 1
h
h 1
airfoils as well as the Power Turbine airfoils are cooled. They are
1
pa E
04 PR c 1
made of INCO 738 coated with a silicon aluminate coating, [18].
P P
-
PR
Cph nEnC
TIT
(2)
4.3 4.0
C T
c 1
1 pa 04 PR c 1
Cph nE TIT
-
-
-
WAVE ROTOR TOPPED TWO-SHAFT GAS TURBINE
Figure 4 presents the performance curves of wave rotor topped two-shaft gas turbines at design point for various values of rc and TIT, illustrated with continuous lines in comparison to the base line (without wave rotor) two-shaft gas turbines illustrated with dotted lines. These results correspond to PR=1.8 and nE=nC=0.83, values widely accepted in the literature, [8-11]. Dotted lines with triangular symbols illustrate the performance of the baseline engines while continuous lines with spherical symbols of the same color illustrate the performance of the wave rotor-topped engines. Each symbol in the diagram corresponds to the design point conditions of a specific two-shaft gas turbine. From this figure, it can be seen that for a given value of turbine inlet temperature, thermal efficiency of wave-rotor topped engines, as well as specific power are increasing with respect
to their values of the base line engines. As it is easily seen, the performance curves of the wave rotor-topped engines are shifted to the upper right part of the diagram. Figure 5 presents the sfc – ws distribution of wave rotor topped two-shaft gas turbines at design point conditions for various values of rc and TIT. One can observe that the performance curves of the wave rotor-topped engines are shifted to the lower right part of the diagram compared to the corresponding baseline gas turbines without wave rotor. Therefore the wave rotor integration, decreases baseline engines sfc and simultaneously increases its ws.
Figures 4 and 5 indicate that low values of the compressor pressure ratio, rc, are more favorable to the integration of a four port wave rotor to a gas turbine in terms of thermal efficiency, specific fuel consumption and specific power, especially at high values of TIT.
Fig.4. Thermal efficiency against specific power of baseline and wave rotor topped two-shaft gas turbines at design point
Fig. 5 Specific fuel consumption against specific power of baseline and wave rotor-topped two-shaft gas turbines at design point
Figure 6 shows qualitatively the effect of integrating a four-port wave rotor on two-shaft engines. In this figure, a typical case with PR=1.8, nC=nE=0.83 for TIT=1500 K is presented. In the same figure, the percentage increase in ws (blue bars) and in nth (grey bars) are illustrated.
A clear benefit in terms of ws and nth increase for all values of rc considered can be seen. More specifically, the prevalent increase in nth reaches 19.3% and in ws reaches 18% for compressor ratio rc=5. This increase decays as rc increases, ending up to a minimum value of 4.8% and 2.6% for nth and ws respectively, for rc=30. This means that for all range of gas turbines there is a clear performance enhancement.
Figure 7 shows qualitatively the effect on specific fuel consumption of integrating a four-port wave rotor on two-shaft engines. A typical case with PR=1.8, nC=nE=0.83 for TIT=1500 K is presented. The major decrease in sfc is 23.9% for rc=5, descending to 5% for rc=30. Conclusively from Figs. 6 and 7, performance enhancement of two- shaft wave rotor-topped engines operating at design point conditions having TIT=1500 K is maximized for low values of rc. Referring to Figs. 4 and 5, it is concluded that the higher the TIT (especially for low values of rc) the more the benefits are for the engines performance.
.
Fig. 6 Increase in specific power (ws) (grey bars) and thermal efficiency (nth) (blue bars) of two-shaft gas turbines topped with four-port wave rotor
with C=E=0.83, PR=1.8 for TIT=1500 K
Fig. 7 Reduction in specific fuel consumption (sfc) of two-shaft gas turbines topped with four-port wave rotor with C=E=0.83, PR=1.8 for TIT=1500 K
-
WAVE ROTOR RECUPERATED GAS TURBINES
In industrial gas turbine industry, fuel economy can be achieved by introducing a recuperator in the baseline engine, [11]. This device recovers waste energy from the gas turbine exhaust, preheating the air entering the combustion chamber, improving cycle efficiency and
CphT06 CpcT05,hyp
cc
cc
mf m n FCV
(11)
reducing fuel consumption, [17]. The development of the Rolls Royce WR-21 engine for marine applications is based on this
The hot exit temperature of the recuperator (T10) is calculated as:
concept, [18].
Under this perspective, a four-port wave rotor can be introduced to
T10 T09 nHE T09 T5.1
(12)
the basic gas turbine recuperator cycle, as illustrated in Fig. 8.
where nHE is the efficiency of the recuperator (its value lies between 0.84 and 0.92).
The updated value of the cold exit of the recuperator is calculated as:
T05,real T5.2 T5.1 nHE T09 T5.1
(13)
If the quantity
T05,real T05,hyp T05,real
is less than a prescribed error (e.g.
Fig. 8 Two-shaft recuperated Gas Turbine configuration, C: gas turbine compressor, T: turbine, CC: combustion chamber, WR: wave rotor, CT: compressor turbine, PT: power turbine, HE: heat exchanger (recuperator)
The thermodynamic calculation of the recuperator is based on an iterative procedure. Initially the temperature of the cold exit 5.2 of the heat exchanger is assumed (T05,hyp). Then fuel mass flow through the combustion chamber is:
0.001), then the calculation is converged, otherwise a new value
T05,hyp T05,real is assumed and a new iteration begins.
The pressure loss in the recuperator is expressed by means of the recuperator pressure loss PHE,loss that takes values between 1% and 4%. The performance map of two-shaft wave rotor recuperated engines with respect to the corresponding two-shaft baseline
recuperated engines at design point is shown in Fig. 9.
Fig. 9 Thermal efficiency percentage against specific power for baseline and wave rotor-topped two-shaft recuperated gas turbines
As for the case of two-shaft wave rotor-topped engines without recuperator (shown in Fig. 4), it can be observed that for low values of rc, the integration of the wave rotor reduces significantly the engines specific fuel consumption especially at high values of TIT. At higher TIT values, the performance curves of the topped engines recover their expected fish-hook shape. Figure 10 shows qualitatively the effect of integrating a four-port wave rotor on two-shaft recuperated engines. A typical case with PR=1.8,
nC=nE=0.83 for TIT=1500 K is illustrated. In the same figure, the percentage increase in nth is shown (the specific power, ws is not affected by the presence of the recuperator). From this figure, it can be seen that there is a benefit in terms of nth increase for all values of rc considered. More specifically, the prevalent increase in nth reaches 22.6% for compressor ratio rc=5, whereas the minimum increase is never less than 19%. This increase is kept almost constant as rc increases, even for engines with rc=25 or 30.
Fig. 10 Increase in thermal efficiency (nth) for two-shaft rcuperated gas turbines topped with four-port wave rotor with C=E=0.83, PR=1.8 for TIT=1500 K
Figure 11 shows qualitatively the effect on specific fuel consumption when integrating a four-port wave rotor on two-shaft recuperated engines. A typical case with PR=1.8, nC=nE=0.83 for TIT=1500 K is presented. The decrease in sfc is as much as 29.2% for rc=5, and for rc=15 is 23.7%. From Figs. 10 and 11, performance enhancement of two-shaft wave rotor-topped recuperated engines
operating at design point conditions having TIT=1500 K is maximized for low values of rc, but there is a net benefit which is kept almost constant independent of the value of compressor pressure ratio. For the typical value of TIT examined, the net benefit in terms of thermal efficiency is more than 19% and the reduction in sfc is close to 24% for all compressor pressure ratio values examined.
Fig. 11 Reduction in specific fuel consumption (sfc) for two-shaft recuperated gas turbines topped with four-port wave rotor with C=E=0.83, PR=1.8 for
TIT=1500 K.
-
INTEGRATION CHALENGES
The Rolls-Royce Alison 250 turboprop two-shaft gas turbine integrating a four-port wave rotor was built as a demonstrator [19]. The original engine was modified to integrate the wave rotor and the associated ducting, keeping compressor, turbine and combustor the same. The rotor diameter and length were approximately equal
to the tip diameter of the High Pressure Turbine. The wave rotor was mounted on a separate shaft between the turbine and combustor. The rotor spins coaxially with the gas turbine shaft at approximately one-third the speed of the gas generator spool through its operating range. As far as it concerns power plant gas turbines, typical wave rotor diameter and length are similar to those of the baseline engine. It was found to produce 11.4% more
shaft power (+20% specific power) with a 22% decrease in engines sfc at design point conditions, values comparable with the present simulations. The greatest challenges are related to the design of the ducts connecting the combustion chamber to the turbine due to high temperatures, [20].
As for the case of marine gas turbines, the integration of a four- port wave rotor to an existing aero-derivative gas turbine is expected to increase the overall length of the engine as much as the turbine diameter, without any increase in the maximum diameter of the engine.
A comparison between the wave rotor-topped two-shaft recuperated gas turbines and the wave rotor-topped two-shaft gas turbines is illustrated in Fig. 12.
One can observe that the thermal efficiency of wave rotor-topped two-shaft recuperated gas turbines is comparable to combined cycle efficiencies, surpassing 50% for values of TIT1400 K, whereas the specific power is almost the same between the wave rotor-topped recuperated two-shaft and the wave rotor-topped engines. From this figure, it can be also seen that the integration of a wave rotor to a two-shaft recuperated engine, favors engines with low compressor pressure ratios, rc. For two-shaft topped engines the highest thermal efficiencies are attained to intermediate values of rc.
The expected merits of wave rotors for marine propulsion systems include:
-
Implementation to naval and commercial ships.
-
Implementation to existing aero-derivative gas turbines without major changes to the basic components.
-
Increase in thermal efficiency of the baseline engine.
-
Increase in specific power of the baseline engine.
-
Reduction in specific fuel consumption of the baseline engine.
-
Increment only in engines length without significant changes in other dimensions and weight.
-
Possibility of in-rotor constant volume combustion, [21], replacing the conventional combustion chamber of gas turbines.
Possible demerits are related to the fact that the wave rotor technology being novel and under development, needs investments to be conducted in:
-
Design and manufacturing of necessary ducting to connect the wave rotor to existing components of the gas turbine.
-
Cooling requirements of the ducts connecting combustion chamber exit to wave rotor hot inlet port.
-
Manufacturing of prototypes for experimental performance validation and design optimization of wave rotor-topped engines.
Fig. 12. Comparison of performances between wave rotor-topped two-shaft recuperated (continuous lines) and wave rotor-topped two-shaft gas turbines (dashed lines)
V. CONCLUSIONS
In this article, performance assessment of two-shaft gas turbine engines topped with a four-port wave rotor as a prime mover or combined with steam turbines for seagoing ships was performed. Integration of wave rotor technology in marine gas turbines can moderate the fuel consumption and increase the specific power of the engine. In marine gas turbines, the extra weight of the wave rotor-
topped engine is negligible with respect to the weight of the ship and the extra dimensioning due to the wave rotor and the associated ducting do not impose major changes in the machine room. In the thermodynamic model developed, the compressor, gas turbine and compressor turbine of the baseline engine are kept unchanged to keep the wave rotors integration cost low. Ambient pressure and temperature, thermodynamic constants for the air and hot gases, thermal efficiencies for compressor, compressor turbine and power turbine as well as compression and expansion efficiencies for the
processes inside the wave rotor, are the input data required. Performance maps at design point illustrate the benefits of wave rotor-topped engines with respect to the corresponding baseline engines. Depending on the design requirements concerning specific power and specific fuel consumption, the topped engines maps help to select the most favorable engine and its precise operating conditions (compressor pressure ratio, turbine inlet temperature).
For two-shaft engines working at a given compressor pressure ratio, the higher the turbine inlet temperature is, the more the benefit gain of the wave rotor topped engine is attained in terms of thermal efficiency, specific fuel consumption and specific power. Assuming compression and expansion efficiencies inside the wave rotor, as well as ducting and leakage pressure losses specified in the literature [10], [11], it was calculated for typical aero-derivative engines, such as the GE LM2500 series of the RR Olympus that that the increase in thermal efficiency remains higher than 8% and the increase in specific power remains higher than 6% at TIT=1500 K.
For the case of wave rotor-topped recuperated gas turbine engines, the thermal efficiency increases by at least 19% and the specific fuel consumption decreases by at least 24% for all pressure ratios examined for TIT=1500 K with respect to the baseline recuperated engines. Peak thermal efficiency can exceed 50% for TIT1400 K and low values of the compressor pressure ratio.
Four-port wave rotors have the potential to enhance the performance of marine gas turbines, although there are challenges to be successfully exceeded.
REFERENCES
-
International Maritime Organization, Marine Environment Protection Committee, MEPC 58/23/Add.1, Annex 14, RESOLUTION MEPC.177(58). Adopted on 10 October 2008, Amendments to the Technical Code on Control of Emissions of Nitrogen Oxides from Marine Diesel Engines. 2008.
-
A. Armellini, S. Daniotti and P. Pinamonti, Gas Turbines for power generation on board of cruise ships: a possible solution to meet the new IMO regulations? Energy Procedia, Vol. 81, pp. 540547, 2015.
-
D. Barsi, A. Bono, F. Satta and P. Zunino, Gas turbine prime movers fuelled by LNG as a future alternative for sustainable power in marine propulsion: current emission policy assessment and exhaust quality evaluation, E3S Web of Conferences Volume 113, Article No.02018, 2019.
-
G.A. Thomson, D.J. Pratley and D.A. Owen, Intercooling and Regenerating the Modern Marine Gas Turbine Propulsion System, SAE Transactions, Vol. 96, pp.169-175, 1987.
-
F. Haglind, A review on the use of gas and steam turbine combined cycles as prime movers for large ships. Part I: Background and design, Energy Conversion and Management Vol. 49, pp. 34583467, 2008.
-
H.E. Weber, Shock Wave Engine Design, John Wiley and Sons, New York, 1995.
-
L.A. Povinelli, G.E. Welch, M.A. Bakhle and G.V. Brown, Potential Application of NASA Aerospace Technology to Ground-Based Power Systems. NASA TM 2000-209652, 2000.
-
J. Wilson and D. Paxson, Jet Engine Performance Enhancement through use of a Wave-Rotor Topping Cycle, NASA TM 4486, 1993.
-
S.M. Jones and G.E. Welch, Performance Benefits for Wave Rotor- topped Gas Turbine Engines, International Gas Turbine and Aeroengine Congress & Exhibition, Birmingham, UK. Paper No. 96- GT-75, 1996.
-
A. Fatsis, Design Point Analysis of Two-Shaft Gas Turbine Engines topped by Four-Port Wave Rotors for Power Generation Systems, Propulsion and Power Research, Volume 8, Issue 3,pp.183-193, 2019.
-
A. Fatsis, Performance Enhancement of One and Two-Shaft Industrial Turboshaft Engines Topped with Wave Rotors, Int. Journal of Turbo and Jet Engines, Vol. 34. Issue, 2. pp. 137-147, 2018.
-
J.H. Horlock, Advanced Gas Turbine Cycles, Pergamon, Cambridge, United Kingdom, 2003.
-
A.M.Y. Razak, Industrial Gas Turbines, Performance and Operability, Woodhead Publishing Limited, Cambridge, UK., 2007.
-
M. Ebaid and Q. Al-Hamdan, Thermodynamic Analysis of Different Configurations of Combined Cycle Power Plants, Mechanical Engineering Research. Vol. 5. No. 2, 2015.
-
S.W. Moon, H.W. Kwon, T.S. Kim, D.W. Kang and J.L. Sohn, A novel coolant cooling method for enhancing the performance of the gas turbine combined cycle, Energy. Vol. 160. pp. 625-634, 2018.
-
K.B. Prasad, V.T. Chand, N. Kumar, K. Ravindra and V.N.B. RAO, Thermodynamic Analysis of Air Cooled Gas Turbine in Marine Applications, International Journal of Thermal Technologies. Vol. 6. No. 1. pp. 32-39, 2016.
-
S.B. Shepard, T.L. Bowen and J.M. Chiprich, Design and development of the WR-21 Intercooled Recuperated (ICR) Marine Gas Turbine, ASME Paper 94-GT-79, 1994.
-
R. Colin, The WR-21 Intercooled Recuperated Gas Turbine Engine Integration Into Future Warships, IGTC2003Tokyo OS-203. Proceedings of the International Gas Turbine Congress Tokyo, Japan, 2003.
-
G.E. Welch, D.E. Paxson, J. Wilson and P.H. Snyder, Wave-Rotor- Enhanced Gas Turbine Engine Demonstrator, NASA TM 209459, 1999.
-
P.H. Snyder, Wave Rotor Demonstrator Engine Assessment, NASA CR 198496, 1996.
-
T.M. Elharis, S.D. Wijeyakulasuriya, and M.R. Nalim, Wave Rotor Combustor Aero-thermodynamic Design and Model Validation based on Initial Testing, AIAA Paper 2010-7041, 46th AIAA / ASME / SAE
/ ASEE Joint Propulsion Conference & Exhibit. Nashville, USA, 2010.