
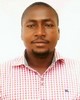
- Open Access
- Authors : Ibrahim A. Sukamari , Abdulaziz Mohammed , Bitrus Kwaji
- Paper ID : IJERTV9IS120274
- Volume & Issue : Volume 09, Issue 12 (December 2020)
- Published (First Online): 16-01-2021
- ISSN (Online) : 2278-0181
- Publisher Name : IJERT
- License:
This work is licensed under a Creative Commons Attribution 4.0 International License
Assessment of The Suitability of Some Groundwater Sources in Mubi for Domestic Application
Ibrahim A. Sukamari1*, Abdulaziz Mohammed2, Bitrus Kwaji2
1Depatment of Civil Engineering Technology, Federal Polytechnic Mubi, Nigeria
2Department of Geology and Mining Technology, Federal Polytechnic Mubi, Nigeria
Abstract:- This study investigated the quality of groundwater from shallow hand dug wells in Mubi. Physicochemical analysis of some important water quality parameters was done and the results obtained compared to the World Health Organisation (WHO) and Nigerian Standard for Drinking Water Quality (NSDWQ). Results obtained show that Iron failed by both standards adopted for checking the compliance while manganese failed to meet the NSDWQ standard for well 2 and well 6 (DW2 and DW6). Furthermore, results of Total Hardness for well 4 (DW4) well 6 (DW6), well 7 (DW7), well 10 (DW10) and well 11 (DW11) failed. The water quality of all locations sampled could be considered safe for domestic use with regard to their physicochemical character as determined by this study.
Key words: Hand dug well, physicochemical, compliance, water quality, groundwater
INTRODUCTION
In order to guarantee public health, access to adequate water of good quality must be given to the people (Sukamari et al., 2020). However, safe water supply for domestic use in many urbanizing communities of the world is increasingly becoming an issue of great concern due to the impact of human population growth and its consequent expansion of anthropogenic influences. The human industrial, commercial and agricultural complexes, all expanding to support the rapidly increasing human population, have resulted in significant pollution burden on the available water resources. Although to a greater extent the surface water sources are more susceptible to pollution and are therefore more rapidly polluted, the rate at which the groundwater resources are polluted and contaminated by natural and anthropogenic activities in recent times is alarming (Egbueri, 2020).
Notwithstanding, groundwater is the most preferred source of water in various user sectors on account of its near universal availability, dependability and low capital cost (Sukumaran et al., 2015). It is considered the healthiest source of drinking water since it is subjected to numerous physiochemical phenomenon and reactions that have bearing on its final quality as it percolated through the soil structure. Consequently, the quality of a groundwater can be said to be a function of all the processes and reactions it went through, from the moment it condensed in the atmosphere to the time it is discharged by a well or a spring.
One principal way groundwater contamination or pollution can occur is naturally, through geological condition such as
rock-water interactions (Dugga et al., 2020; Ishaku & Abdulhakeem, 2010). Other natural factors include precipitation, the nature of groundwater flow and its direction; its time of residence, and the intra/inter-aquifer interactions. Moreover, through anthropogenic influences, leaching of pollutants originating from various activities such as industrial and domestic wastes and wastewater generation and discharge, commerce and agriculture also have capacity to degrade groundwater quality (Shukla & Saxena, 2020).
Groundwater is the most important source of water supply for domestic, commercial and agricultural activities in Mubi. For this reason, the assessment of the groundwater quality of Mubi is very important to the sustainability of its scarce water resources as well as the maintenance of the health of the environment. The quality of human life is significantly dependent on the quality of water man has access to and the health of the environment he inhabits. Poor drinking water affects the health of both man and the environment.
This study therefore set out to assess the suitability of some groundwater sources in Mubi for domestic application.
MATERIALS AND METHODS
Location of the Study Area
The study area is geographically situated on latitude 10°2930 to 10°630 and longitude 13°1400 to 13°3200, and covers a total land mass of 506.4 2 (Figure 1). The Principal economic activities in Mubi is commerce and agriculture. River Yedzaram and River Mudzira are the two significant rivers found in Mubi. River Yedzeram flows northward from its source at the Hudu Hills located south-east of Mubi, and receiving flow from river Mudzira at Digil, it drains into the Lake Chad after about 330 kilometres (Sukamari et al., 2020; Wante & Anoliefo, 2014 cited Adebayo,2004). Mubi has a climate classified as Equatorial Savannah with dry winter or tropical rainy according to Koppens code (Kottek et al., 2006).
Figure 1: Map of the study area
Geology and Hydrogeology of the Study Area
Mubi is of the Precambrian Basement Complex of the Hawal Massif in the northeastern sector of Nigerias eastern Basement Complex. It is exactly located in the parts that extend into the northern parts of Adamawa State (Figure 2) (Obiefuna, 1997). The rocks within the Hawal Basement are characterized by high grade metamorphic rocks, pervasive migmatization and extensive granite plutonism (Ferre et al, 1996). The rocks are gneiss, granite gneiss, granites and some minor rocks such as dolerite, aplite and pegmatite (Obiefuna, 1997; Adekeye, and Ntekim, 2004; Patrick, 2005; Bassey, and Valdon, 2011). Some parts of the Basement Complex in Mubi is overlain by elluvial deposits which may have been derived from the weathering of the basement complex uphill or insitu (Obiefuna, 1997). Moreover, some areas are characterised by reddish to brownish laterite overburden with characteristics thickness ranging from 5m to 25m overlying the bedrock along river Yedzaram. The predominant geological structures of the area include Older granite with dolerite and pegmatite dykes, as well as quartz feldspar veins, folds, sheer zones, etc. (Adebayo, 2004; Obiefuna, 1997).
Mubi is characterised by two aquifer systems which are both highly localised as is characteristic to groundwater occurrence in the crystalline basement area. These aquifer types are namely: the fractured basement (mainly migmatite granite) aquifer, and the unconsolidated weathered overburden aquifer. In a study by Obiefuna (1997), he found that all hand dug wells studied tap water from the unconsolidated weathered overburden. The weathered overburden are luvisols characteristically made up of low organic matter, low cation exchange capacity and low water
Figure 2: Geological setting of the study area (Modified after Maluskiet al., 1995)
Sampling and Analytical Methodology
Groundwater samples were collected from twelve (12) randomly selected hand dug wells having a range of depth between 9m to12 m in the study area. The preselected wells were sampled twice in the months of August and September. The wells were purged prior to sampling using a decontaminated bailer so that the samples collected were representatives of groundwaters at the sampling locations. Sampling followed procedure described in Triplett et al (2006).
All water samples were collected into 1-liter capacity acid- cleaned High Density Polyethylene (HDPE) bottles with strict adherence to the sampling protocols described by Standard methods (APHA et al., 2017) and were analysed independently.
Samples collected were analysed for temperature, pH, turbidity, total dissolved solids (TDS), total hardness (TH), chloride, nitrate, phosphate, sulphate, sodium, magnesium, calcium, manganese, iron.
For quality assurance purpose, allequipment were adequately calibrated according to standards before measurement, and all reagents used were of analytical grade. Samples for metal analysis were treated in the field with 3 ml concentrated HNO3 per litre in order to bring the pH to less than 2 for its preservation (Begum et al., 2014; David
4
4
4
4
K. et al., 2011). Samples for Cl- , SO 2-, NO3 -and PO 3- analyses were stored in temperature controlled environment at 4 OC for transport and analyses in the laboratory within 24 hours.
retention ability making it very unpredictable in terms of
The concentrations of major anions Cl- , SO 2-, NO
-and
water yielding and retention capacity (Adebayo, 2004). 3- 4 3
PO4 ; TDS and TH in water samples were determined
according to standard methods (APHA et al 2017). The
elemental concentrations for Na2+, Mg2+, Ca2+, Fe and Mn in water samples were determined by an Atomic Absorption Spectrophotometer AAS (Buck Scientific, VPG 210) following procedure as reported by David K. et al., (2011).
RESULTS AND DISCUSSION
The results of the physicochemical parameters for the different dug wells sampled as analysed and statistically treated for means of concentrations and deviations from the means as well as mean of means of concentrations are presented in Tables 1-3. The World Health Organisation (WHO) guidelines (WHO, 2017) and the Nigerian Standards for Drinking Water Quality (NSDWQ) guidelines (SON, 2007) were recognized and used as the standards for comparison and checking of compliance. The compliance of the results to the standards adopted is diagrammatically summarised in Figures 2 and 3.
DISCUSSION OF RESULTS
The mean temperature values recorded for all the wells sampled fall below the standard set for maximum permissible water temperature by WHO based on palatability and aesthetic objectives (WHO, 2017). Temperature has been reported to affect the physical, chemical and biological nature of water (Kale, 2016). Significant impact on the quality of groundwater have been observed as a consequence of little increases in its temperature. Commonly affected parameters by temperature increase include magnesium, potassium, sodium, pH, dissolved organic carbon (DOC), As, B, and F (Bonte et al., 2013; Saito et al., 2017).
The temperature values obtained in this study are consistent with temperatures prevailing and obtainable in the tropical belt (Oyem et al., 2014).
From Table 1, it can be seen that the mean of all pH values reported are within limits of acceptability by both quality standards (WHO and NSDWQ). The pH of water is a measure of the concentration of hydrogen ions in the water and an indicator of its alkalinity or its acidity. The pH of water has significant impact on health and the environment as it helps to inhibit or drive many chemical interactions. For example, a pH of less than 6.5 inhibits the human body from the intake of vitamins and minerals and when it exceeds 8.5 the water becomes caustic and irritating (Gupta et al., 2017). The turbidity of water relates to the ability of water to allow or impede the penetration of light due to the scattering effect of suspended particulate matter in the water (Sukamari et al., 2020). Simply put, turbidity in water is the degree of its cloudiness or clarity.
WHO advises that should the turbidity of groundwater supplies exceed 1NTU, then it becomes imperative to determine whether there is a potential risk for such water to cause health problems due to bacteria or nitrate pollution (World Health Organization, 2017). Consequently, groundwaters with a turbidity greater than 1 NTU should be sampled for bacteria and nitrate. The WHO standard as well as the NSDWQ standard for turbidity concerning potability of water is a value of not more than 5 NTU or equal to
5NTU. By these standards, all sampled well meet the criteria of acceptance. The highest recorded value for turbidity was
-
NTU (Table 1) while the least value was 0.52 NTU and the mean of the mean of all wells was computed to be 2.00 NTU.
One of the very important indicator of organic and inorganic pollution in water is the amount of total solids dissolved in it. The higher the concentrations of solids in a given water the higher the degree of contamination irrespective of its source. Aside being a very important pollution indicator, TDS also plays a very important role in aesthetics of water for drinking. Water containing high concentrations of TDS is inferior with regard to palatability and may also produce unfavourable physiological reaction in the transient consumer (David K. et al., 2011; Kumar et al., 2016). With reference to Table 1, all TDS results fall quite below the recommended values of both WHO and NSDWQ standards. Similarly, the mean of the mean of all TDS value indicate that waters around Mubi may be generally fit for consumption as regards the TDS parameter.
With reference to the results presented in Tables 1, the TH values observed for the groundwater sources all fall within the acceptable limits for WHO considering the range of acceptability stipulated therein (100 mg/L as CaCO3 to 500 mg/L as CaCO3).and NSWDQ standards of less or equal to 500 mg/L for domestic supply. Conversely however, with regard to NSWDQ, all results but for DW4, DW6, DW7, DW10 and DW11 failed to meet standard. Total hardness in water is primarily due to the excess of Ca, Mg and Fe salts (Bairu et al., 2013). The high values for TH recorded may be as a result of the weathering of silicates which are present in the formation of the geology of the study area. Guillemant et al. (2000) and Sengupta, (2013) indicated that hard water has the tendency to influence mortality, in particular, cardiovascular mortality in addition to reproductive failure, cancer and Alzheimers disease.
Considering Table 2, it can be observed that all the major anions analysed were below their respective maximum allowable limits as per WHO and NSDWQ standards. Such low concentrations notwithstanding, nitrate concentrations of 10 mg/L and above poses a health threat to infants (Sharma & Kaur, 2017) due to its ability to cause infant Methaemoglobin anaemia or the blue baby syndrome (WHO, 2017). Some health risks are associated with ingesting waters with high nitrate concentration even in adults. Ward et al. (2018) reported the risk of specific cancers (colorectal cancer, bladder and breast cancers) and birth defects when nitrate is ingested under conditions that increase formation of N-nitroso compounds.
High concentrations of sulphate has also been linked to some minor health concerns such as catharsis, dehydration and gastrointestinal irritation (Gulumbe et al., 2016). Hyperthyroidism and renal failure are major health concerns tied to high sulphate concentration in drinking water (Sharma & Kaur, 2017).
Anthropogenic and natural sources of the major anions analysed are presented in Table 4.
Table 1: Statistical summary of groundwater characteristics for temperature, pH, turbidity, total dissolved solids and total hardness
Parameters ( ± )
Sample ID
Temp ()
pH
Turb. (NTU)
TDS(mg/L)
TH(mg/L)
DW1
27.50±0.56
6.98±0.48
1.58±0.68
371.75±1.26
199.38±1.49
DW2
27.50±0.58
7.07±0.17
1.10±0.35
366.75±0.96
173.75±0.50
DW3
27.50±0.56
7.33±0.09
0.91±0.20
425.25±0.96
198.75±2.22
DW4
27.25±0.50
6.98±0.10
3.03±0.49
324.75±0.50
122.00±0.82
DW5
27.25±0.50
6.98±0.25
0.52±0.68
305.00±0.82
181.50±1.29
DW6
26.25±0.50
7.00±0.18
3.19±0.08
393.00±0.82
118.38±4.39
DW7
28.00±0.82
7.20±0.08
2.53±0.56
253.75±0.96
120.25±0.96
DW8
27.00±0.81
7.43±0.10
1.74±0.05
365.47±0.70
150.99±0.56
DW9
26.75±0.65
7.20±0.08
5.45±0.76
364.17±5.29
198.82±0.23
DW10
27.75±0.96
6.65±0.13
0.92±0.51
364.10±0.12
104.41±0.83
DW11
27.25±0.50
6.90±0.08
1.39±0.12
362.25±0.52
135.48±0.59
DW12
27.50±0.58
7.28±0.22
1.58±0.68
349.87±0.86
190.74±0.94
Mean of mean
27.29
7.08
2.00
353.84
157.87
WHO
27-29
6.5-8.5
<5
500
100-500
NSDWQ
Ambient
6.5-8.5
5
500
150
=mean of wells, =standard deviation, TH (mg/L as 3)
Table 2: Statistical summary of groundwater characteristics for chloride, sulphate, nitrate and phosphate
Parameters ( ± )
Sample ID
Chloride(mg/L)
Sulphate(mg/L)
Nitrate(mg/L)
Phosphate(mg/L)
DW1
13.95±0.93
41.56±0.98
12.24±0.98
2.39±0.48
DW2
15.53±0.78
39.13±0.94
13.25±0.54
2.03±0.08
DW3
16.06±0.49
32.66±0.96
9.51±0.28
1.15±0.06
DW4
10.86±0.25
43.33±0.62
13.34±0.29
1.65±0.04
DW5
19.56±0.50
48.16±0.98
16.25±0.44
0.68±0.02
DW6
20.91±0.25
53.57±0.81
14.92±0.09
0.66±0.06
DW7
24.79±0.65
48.04±0.06
12.87±0.13
0.90±0.02
DW8
18.85±0.71
61.40±1.00
20.95±0.17
0.99±0.14
DW9
35.88±0.77
28.24±0.63
12.43±0.67
1.53±0.10
DW10
10.99±0.05
22.18±0.35
6.39±0.94
0.40±0.09
DW11
20.02±0.68
45.43±0.50
9.32±0.35
1.29±0.43
DW12
27.13±0.23
28.92±0.89
9.09±0.17
1.45±0.14
Mean of mean
19.54
41.05
12.55
1.26
WHO
250
250
50
5
NSDWQ
250
100
50
–
=mean of wells, =standard deviation
From the results presented in Table 3, it is observed that all the light and heavy metals analysed recorded values below both the WHO and NSDWQ standards except for iron and manganese. Iron exceeded the maximum allowable concentration in all well water samples and while manganese failed in two wells (DW2 and DW6) by NSDWQ standard. The highest iron concentration exceeded both
standards by 483.33%. The presence and the relative high concentrations of the light and heavy metals is as a result of reductive dissolutions of the soil minerals (Egbueri, 2020; Khozyem et al., 2019).containing high TDS is of inferior palatability and may produce unfavorable physiological reaction in the transient consumer (David K. et al., 2011; Kumar et al., 2016).
Table 3: Statistical summary of groundwater characteristics for sodium, calcium, magnesium and manganese and iron
Parameters ( ± )
Sample ID
Sodium(mg/L)
Calcium(mg/L)
Magnesium(mg/L)
Manganese(mg/L)
Iron(mg/L)
DW1
10.28±0.64
38.27±0.44
9.06±0.68
0.18±0.22
1.09±0.14
DW2
11.59±0.99
43.52±0.68
11.81±1.03
0.23±0.26
0.93±0.08
DW3
15.28±0.33
37.98±0.21
11.99±0.11
0.15±0.23
0.84±0.06
DW4
25.44±0.85
27.69±0.39
7.89±0.12
0.05±0.58
1.08±0.07
DW5
19.54±0.13
48.16±0.98
10.72±0.30
0.03±0.50
0.61±0.11
DW6
16.03±0.04
28.89±0.10
10.85±0.20
0.23±0.22
1.08±0.10
DW7
11.08±0.15
48.25±0.54
21.93±0.62
0.10±0.20
1.15±0.12
DW8
9.31±0.60
41.88±0.74
13.14±0.18
0.08±0.10
1.45±0.11
DW9
8.16±0.69
26.31±0.52
21.63±1.06
0.13±0.10
0.47±0.09
DW10
7.00±0.27
23.58±0.42
19.60±0.94
0.18±0.10
0.83±0103
DW11
7.99±0.07
27.56±0.40
35.45±1.00
0.13±0.10
0.85±0.04
DW12
7.83±0.13
42.14±0.20
45.52±0.59
0.18±0.22
0.45±0.16
Mean of mean
12.46
36.19
18.30
0.14
0.90
WHO
200
75
150
0.4
0.3
NSDWQ
200
–
20
0.2
0.3
=mean of wells, =standard deviation
Table 4: Some anthropogenic
and natural sources
of anion pollution in grou
ndwater
Anion
Possible anthropogenic sources
Source
Source
Chloride
Batabyal & Chakraborty (2015)
Kelly et al. (2012)
Sulphate
Gulumbe et al. (2016)
Elahcene et al. (2019) Sharma & Kaur, (2017) Egbueri, (2020)
Nitrate
DAlessandro et al., (2012).
DAlessandro et al., (2012).
Phosphate
<>Fadiran et al. (2008) Nemati Varnosfaderany et al.( 2009) decomposition of rocks and minerals,
Fadiran et al. (2008) Nemati Varnosfaderany et al.( 2009)
-
saline water intrusion
-
application of de-icers,
-
agricultural activities,
-
domestic wastewater,
-
industrial chemical,
-
landfill leachates
-
weathering of salt- bearing geological formations,
-
Chloride from marine aerosols or volcanic gases in precipitation and dry deposition
-
wastewater and septic system effluent,
-
discharge of industrial wastes
-
washing of acidic lateritic soils,
-
acid precipitation,
-
fulvic/humic soils,
-
oxic conditions that could convert sulfide (H2S) in soil to sulphate
-
leaching of gypsum, barite, epsom and
-
the dissolution of salts of sulfuric acid
-
usage of nitrogen based fertilizers,
-
disposal of wastes in proximity to the sampling locations
-
human-animal bodily wastes
-
leaching/washing of soils with animal waste material
-
fertilizers,
-
wastewater and septic system effluent,
-
animal wastes,
-
detergents,
-
industrial discharge,
-
phosphate mining,
-
drinking water treatment,
-
forest fires
-
atmospheric deposition,
-
natural
-
weathering of soluble inorganic materials,
-
decaying biomass,
-
runoff
-
sedimentation
Test Conc. WHO NSDWQ
500
400
300
200
100
0
Figure 2: Compliance of tested groundwater with drinking water standards for high-value and moderate-value parameters.
Test Conc WHO NSDWQ
8
7
6
5
4
3
2
1
0
Figure 3: Compliance of tested groundwater with drinking water standards for high-value and moderate-value parameters.
Mean of Mean Conc. Values
Mean of Mean Conc. Values
CONCLUSION
The physicochemical parameters analysed, except for iron, manganese and TH. Iron failed by both standards adopted for checking the compliance of the waters sampled to the standards while manganese failed to meet the NSDWQ standard for DW2 and DW6. Furthermore, with regard to NSWDQ, all results of TH for DW4, DW6, DW7, DW10 and DW11 failed. The water quality of all locations sampled could be considered safe for domestic use with regard to their physicochemical character as determined by this study.
Mean of Mean Conc. Values
Mean of Mean Conc. Values
REFERENCES
-
APHA, AWWA, & WEF. (2017). Standard Methods for the Examination of Water and Wastewater. In R. B. Baird;, Andrew D. Eaton;, & Eugene W. Rice (Eds.), American Public Health Association, American Water Works Association, Water Environment Federation.
-
Adekeye, J.L.D & Ntekim, E.E. (2004). The Geology of Song area in Southern Hawal Massif, N.E. Nigeria. Zuma Journal of Pure and Applied Sciences 6 (2), 145 151.
-
Bairu, A., Tadesse, N., & Amare, S. (2013). Use of geographic information system and water quality index to assess suitability of groundwater quality for drinking purposes in Hewane areas, Tigray, Northern Ethiopia. Ethiopian Journal of Environmental Studies and Management, 6(2), 110123.
https://doi.org/10.4314/ejesm.v6i2.1
-
Bassey, N.E. & Valdon, Y.B. (2011). Structural Features and Rock Crystal Mineralization in Dumne Area, Hawal Basement Complex, N.E. Nigeria. International Journal of Environmental Science, vol. 7, No. 3. pp 1 8.
-
Batabyal, A. K., & Chakraborty, S. (2015). Hydrogeochemistry and Water Quality Index in the Assessment of Groundwater Quality for Drinking Uses. Water Environment Research, 87(7), 607617. https://doi.org/10.2175/106143015×14212658613956
-
Begum, A., Mondal, S., Ferdous, Z., Zafar, M. A., & Ali, M. M. (2014). Impact of water quality parameters on monosex tilapia (. Int. j. Anim. Fish. Sci, 2(1), 1421.
-
Bonte, M., van Breukelen, B. M., & Stuyfzand, P. J. (2013). Temperature-induced impacts on groundwater quality and arsenic mobility in anoxic aquifer sediments used for both drinking water and shallow geothermal energy production. Water Research, 47(14), 50885100. https://doi.org/10.1016/j.watres.2013.05.049
-
DAlessandro, W., Bellomo, S., Parello, F., Bonfanti, P., Brusca, L., Longo, M., & Maugeri, R. (2012). Nitrate, sulphate and chloride contents in public drinking water supplies in Sicily, Italy. In Environmental Monitoring and Assessment (Vol. 184, Issue 5). https://doi.org/10.1007/s10661-011-2155-y
-
David K., E., Senu, J., Fianko, J. R., Nyarko, B. K., Adokoh, C. K., & Boamponsem, L. (2011). Groundwater Quality Assessment: A Physicochemical Properties of Drinking Water in Rural Setting of Developing Countirs. Canadian Journal on Scientific and Industrial Research, 2(4), 171180.
-
Dugga, P., Pervez, S., Tripathi, M., & Siddiqui, M. N. (2020). Spatiotemporal variability and source apportionment of the ionic components of groundwater of a mineral-rich tribal belt in Bastar, India. Groundwater for Sustainable Development, 10(February), 100356. https://doi.org/10.1016/j.gsd.2020.100356
-
Egbueri, J. C. (2020). Groundwater quality assessment using pollution index of groundwater (PIG), ecological risk index (ERI) and hierarchical cluster analysis (HCA): A case study. Groundwater for Sustainable Development, 10(October 2019), 100292. https://doi.org/10.1016/j.gsd.2019.100292
-
Elahcene, O., El-Azim, H. A., & Aidoud, A. (2019). Physico- chemical and bacteriological analysis of water quality in different types of water from the Ain Zada Dam of Bordj Bou Arreridj (Algeria). Egyptian Journal of Aquatic Biology and Fisheries, 23(3), 423439. https://doi.org/10.21608/ejabf.2019.49062
-
Fadiran, A. O., Dlamini, S. C., & Mavuso, A. (2008). A comparative study of the phosphate levels in some surface and ground water bodies of Swaziland. Bulletin of the Chemical Society of Ethiopia, 22(2), 197206.
https://doi.org/10.4314/bcse.v22i2.61286
-
Ferre, E.C., Bouchez, J.L., Kozminski, G., and Omitgun, A.A. (1996). SLAR Interpretation for Structural Mapping in the Basement of Northern Nigeria. Paper presented at the 11thThermatic Conference and Workshops on Applied Geologic Remote Sensing Las Vegas, Nevada, 27 29, Feb. 1996, pp. 573 582.
-
Guillemant, J., Le, H. T., Accarie, C., Du Montcel, S. T., Delabroise, A. M., Arnaud, M. J., & Guillemant, S. (2000). Mineral water as a source of dietary calcium: Acute effects on parathyroid function and bone resorption in young men. American Journal of Clinical Nutrition, 71(4), 9991002.
https://doi.org/10.1093/ajcn/71.4.999
-
Gulumbe, B. H., Aliyu, B., & Manga, S. S. (2016). Bacteriological and Physicochemical Analyses of Aliero Dam Water. International Journal of Innovative Studies in Sciences and Engineering Technology, 4863(April), 3034.
-
Gupta, N., Pandey, P., & Hussain, J. (2017). Effect of physicochemical and biological parameters on the quality of river water of Narmada, Madhya Pradesh, India. Water Science, 31(1), 1123. https://doi.org/10.1016/j.wsj.2017.03.002
-
Ishaku, J. ., & Abdulhakeem, A. (2010). Hydrogeochemical assessment of groundwater in Kaltungo and Environs, northeastern Nigeria. Global Journal of Geological Sciences, 8(2), 187206. https://doi.org/10.4314/gjgs.v8i2.62773
-
Kale, V. S. (2016). Consequence of Temperature , pH , Turbidity and Dissolved Oxygen Water Qualiy Parameters. International Advanced Research Journal in Science, Engineering and Technology, 3(8), 186190.
https://doi.org/10.17148/IARJSET.2016.3834
-
Kelly, W. R., Panno, S. V, & Hackley, K. (2012). The Sources, Distribution, and Trends of Chloride in the Waters of Illinois. Illinois State Water Survey, Bulletin B(March), 159.
http://hdl.handle.net/2142/90994
-
Khozyem, H., Hamdan, A., Tantawy, A. A., Emam, A., & Elbadry,
E. (2019). Distribution and origin of iron and manganese in groundwater: case study, Balat-Teneida area, El-Dakhla Basin, Egypt. Arabian Journal of Geosciences, 12(16), 116.
https://doi.org/10.1007/s12517-019-4689-1
-
Kottek, M., Grieser, J., Beck, C., Rudolf, B., & Rubel, F. (2006). World map of the Köppen-Geiger climate classification updated. Meteorologische Zeitschrift, 15(3), 259263.
https://doi.org/10.1127/0941-2948/2006/0130
-
Kumar, Y. R., Reddy, M. D., Jayaveera, K. N., & Pradesh, A. (2016). STUDIES ON GROUNDWATER QUALITY IN ANANTAPUR DISTRICT. International Journal of Pllant, Animal and Evironmental Science, 6(2), 219225.
-
Maluski, H., Coulon, c., Popoff, M., Boudin, P. (1995). 40/Ar/39Ar chronology, petrology and geodynamic setting of Mesozoic to early Cenozoic magmatism from the Benue Trough, Nigeria Jour. Geol. Soc. Lond. 152: 322-326.
-
Nemati Varnosfaderany, M., Mirghaffary, N., Ebrahimi, E., & Soffianian, A. (2009). Water quality assessment in an arid region using a water quality index. Water Science and Technology, 60(9), 23192327. https://doi.org/10.2166/wst.2009.669
-
Obiefuna, G. I. (1997). The Geology and Hydrogeology of Mubi NE Nigeria. Water Resources-Journal of NAH, 8(1 & 2), 4151.
-
Oyem, H. H., Oyem, I. M., & Ezeweali, D. (2014). Temperature, pH, Electrical Conductivity, Total Dissolved Solids and Chemical Oxygen Demand of Groundwater in Boji-Boji Agbor/Owa Area and Immediate Suburbs. Research Journal of Environmental Sciences, 8(8), 444450. https://doi.org/10.1111/ijfs.12122
-
Patrick, J.O. (2005). Geological Mapping of Parts of Song Area, Adamawa State, Northeastern Nigeria. B.Tech. Thesis, Department of Geology, Federal University of Technology, Yola. Unpublished. 67p, 2005.
-
Saito, T., Hamamoto, S., Ueki, T., Ohkubo, S., & Moldrup, P. (2017). Temperature change affected groundwater quality in a con fi ned marine aquifer during long-term heating and cooling. Water Research, 94(2016), 120127.
https://doi.org/10.1016/j.watres.2016.01.043
-
Sengupta, P. (2013). Potential health impacts of hard water.
International Journal of Preventive Medicine, 4(8), 866875.
-
Sharma, Y., & Kaur, K. (2017). Determination of Nitrates and Sulphates in Water of Barnala ( Punjab , India ) Region and Their Harmful Effects on Human Lives. International Journal of Advanced Research in Education & Technology, 3(February), 79 82.
-
Shukla, S., & Saxena, A. (2020). Groundwater quality and associated human health risk assessment in parts of Raebareli district, Uttar Pradesh, India. Groundwater for Sustainable Development, 10(January), 100366. https://doi.org/10.1016/j.gsd.2020.100366
-
SON. (2007). Nigeria Standards for Drining Water Quality. In
Nigerian Industrial Standards (Vol. 203).
-
Sukamari, I. A., Kwaji, B., Alheri, J., & Abia, I. E. (2020). Application of Water Quality Index to Assess the Potability of Some Domestic Water Supply Sources in Mubi North , Nigeria. International Journal of Engineering Research & Technology (IJERT), 9(08), 848860.
-
Sukumaran, D., Saha, R., & Saxena, R. C. (2015). Ground Water Quality Index of Patna , the Capital City of Bihar , India. American Journal of Water Resources, 3(1), 1721.
https://doi.org/10.3109/09637486.2012.738649
-
Triplett, Laura D., Burford, Patricia, Sielaff, Brad, Clark, R. C. (2006). Sampling Procedures for Ground Water Monitoring Wells.
September, 15. https://doi.org/10.1017/CBO9781107415324.004
-
Wante, S. P., & Anoliefo, G. O. (2014). Impact of developmental needs of the people of Mubi-North local government area of Adamawa state , Nigeria on environmental sustainability. 6(6), 13031316.
-
Ward, M. H., Jones, R. R., Brender, J. D., de Kok, T. M., Weyer,
P. J., Nolan, B. T., Villanueva, C. M., & van Breda, S. G. (2018). Drinking water nitrate and human health: An updated review. International Journal of Environmental Research and Public Health, 15(7), 131. https://doi.org/10.3390/ijerpp5071557
-
WHO. (2017). Guidelines for Drinkingwater Quality (Fourth (In). World Health Organization.
-
World Health Organization. (2017). WATER QUALITY AND HEALTH – REVIEW OF TURBIDITY: Information for regulators and water suppliers. In WATER QUALITY AND HEALTH – REVIEW OF TURBIDITY: Information for regulators and water suppliers. https://www.who.int/water_sanitation_health/publications/turbidit y-information- 200217.pdf%0Ahttp://www.who.int/water_sanitation_health/publ ications/turbidity-information-200217.pdf
-