
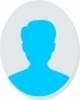
- Open Access
- Authors : Mahesh K , Bassetti Chandrasheker , Lasheer Mohammad
- Paper ID : IJERTV10IS060013
- Volume & Issue : Volume 10, Issue 06 (June 2021)
- Published (First Online): 09-06-2021
- ISSN (Online) : 2278-0181
- Publisher Name : IJERT
- License:
This work is licensed under a Creative Commons Attribution 4.0 International License
Application of Additive Manufacturing Methodology in Legacy Parts
Mahesh K
Cyient Ltd, Hyderabad
Bassetti Chandrasheker
Cyient Ltd, Hyderabad
Lasheer Mohammad
Cyient Ltd, Hyderabad
Abstract The future of successful technology is to adapt to the key mass customization of the product which needs to use the latest manufacturing processes. This reduces the project cycle time, as it takes more time to plan and then execute. The manufacturer needs to be agile. Conventional machining, which can be described as subtractive, material is gradually removed from the stock. Conventional manufacturing processes impose constraints on the design and can hence be described as design- driven by technology. Additive manufacturing (AM), on the opposite hand, is often described as technology-driven intentionally. Manufacturing any complex design without technological constraints is possible in AM. But the limitations in AM technology are the repeatability and the effectiveness to produce critical areas without impact to the desired design profile. Also, the validation of the structural properties concerning design is a limitation in additive manufacturing technology. There are various types of Additive manufacturing methods used for getting the desired properties of the gas turbine engine parts. The cons aspect of additive manufacturing is its adaptability to production thanks to its repeatability. This paper presents design optimization of the parts produced by additive manufacturing using the latest technologies available today such as like Solid Thinking (Inspire, Optistruct, Materialize) software. The paper addresses all these parameters in design for Additive manufacturing using the latest software. A case study is presented by redesign a bracket in gas turbine engines. This paper also has discussed the significance of the identification of cost-driven features and material/powder selection during the preliminary/conceptual design phase of gas turbine components.
KeywordsAdditive manufacturing, Material/Powder selection, Product life cycle
-
INTRODUCTION
The 21st-century industrial revolution depends mostly on the rapid customization of the design. The market trends show companies need rapid design changes to the product to sustain in the market. The rapid customization of design is only possible if we have a manufacturing process that has very little manufacturing time. But to accommodate the change in designs rapidly, the tools used for the manufacturing also require customizations to their features as per the requirements.
The manufacturing lead time for legacy engines and engines under development is the major emphasis for meeting the incorporation date for gas turbine engines. Most of the aero companies are working on technologies which can improve the efficiency of the gas turbine, which can be done either by having altogether new technology like, GTF technology and by possible weight reduction(In the aerospace industry weight is of great importance to the engineering
design) of the legacy engine components and also under development engine components. Complex parts manufacturing requires a lot of skilled labor when we go for a conventional manufacturing process. Meeting the schedules is critical nowadays to meet the change in designs to decrease the product cost. Decision on the design of a product influence the lead time for the component, cost of the manufacturing tools e.g. the method of manufacture of the design will affect manufacturing cost.
Traditionally the design has to pass from various iterations that involve designer and analyst. This design process is the time taken where a designer sends design geometry to the analyst and then the analyst runs his analysis and sends feedback to the designer. This process is repeated until a valid design meets the analysis criteria. The final design is then handed to the manufacturing team, which then may have additional constraints or concerns, and iterations can continue. 3D printing and Additive manufacturing coupled with various optimization technologies (like Topology) allow the design- and-analysis and manufacturing iterations to be reduced significantly.
The below figure depicts the selection of technology based on Complexity and Volume
Fig. 1. Strong cost advantage for complex components
Fig 2. Strong cost advantage for small volume production
-
BACKGROUND
The competitive moves observed in the last two decades were the lead time for manufacturing, evolving from a cost- plus margin paradigm to a market-driven one. This has thrown a real challenge to the strategic sourcing team to create healthy competition among potential suppliers. Making a good choice on the changes after the product is manufactured will be a tough task.
To get reasonable quotes, the strategic sourcing team needs to be familiar with time-driven features, specific manufacturing processes, and materials cost. Sometimes taking control of material, simplifying the complex feature will result in huge cost savings. One solution to these problems is additive manufacturing which reduces the lead time of the product with quick customizations to design. This reduces the cost based on materials, labor, overheads, and increases the profit margins.
There are many additive manufacturing methods like powder- based metal additive manufacturing processes, binder jetting, direct metal laser sintering (DMLS) additive manufacturing processes for complex parts available in the market. This paper concentrates on the powder-based metal additive manufacturing processes and validation methodology for gas turbine parts using Inspire and Optistruct softwares and in addition, it also helps in the understanding of high stress and low-stress areas for optimized design. [6]
To ensure that the part will perform as simulated, a mid-stage validation is conducted on a uniform part before creating the ultimate products.
-
TOPOLOGY OPTIMIZATION
Structural optimization deals with the assembly of material to sustain loads in the best way. It has been used in multiple areas of applications [2]. Problems of structural optimization have been modelled and solved using size, shape or topology optimization. Topology optimization, also known as layout optimization is a mathematical tool which seeks optimal material layout within a given design domain for a given set of boundary and loading conditions. It determines connectivity, geometries of cavities and void locations in the design domain [3].
The base of topology optimization date back to the late 1980s. This is an iterative process that determines the best arrangement of a limited volume of structural material within a given spatial domain to obtain optimal mechanical performance of the design concept. Bendsoe and Kikuchi [4] first introduced this computational technique for the optimal distribution of material in continuum structures. The comparative study on stress and compliance based topology optimization was performed by Shiemels & Endiga [5]. The objective of the problem discussed in this paper is to achieve minimum mean compliance with a constraint on the available mass, or volume and it is intended to explore the role of topology optimization in AM to design a lightweight model.
-
ADDITIVE MANUFACTRING
Manufacturers found that the planning of the design based on the manufacturing process feasibility is very essential for the successful development of the design. A design that has no manufacturability is difficult to be manufactured. So, to overcome all these hindrances we need a quick prototype of te object being selected. 3D printing (or additive manufacturing, AM) manufacturing process is thus selected with more design flexibility. Additive manufacturing now enables both a design and industrial revolution, in various industrial sectors such as aerospace, energy, automotive, medical, tooling, and consumer goods.
The use of Additive Manufacturing (AM) with metal powders may be a new and growing industry sector with many of its leading companies based in Europe. It became an appropriate process to supply complex metal net shape parts, and not only prototypes, as before. [7]
-
Selection of part for Metal additive manufacturing process
With the current development in additive manufacturing the production of all the parts by using this technology is not feasible and not cost-effective. Cost is one of the main parameters in aero engine production. The factors which drive the selection of the components are given in below fig.
-
Complexity of the part (includes size and shape)
-
Customization required
-
Functional integration
Fig 3. Steps involved in Metal additive manufacturing process
Below steps are involved during the selection of aero-engine parts which is suitable for a metal additive manufacturing process,
-
Size of the component:- currently, this technology is explored up to only the part size of 450mm all around and height of 1500mm
-
Enabling a completely new design approach
-
Resolving the constraints of conventional manufacturing
-
Leading to completely new solutions, e.g move from metal to plastic, making a part lightweight, yet functional
-
New design not possible with conventional methods
-
Material to be used:- currently, only a few Nickel based and Ti-based material are qualified for the use
-
FAA (Federal Aviation Administration) certification
-
-
-
The Overview of metal additive manufacturing processes
Metal additive manufacturing technologies offer many key benefits.
-
More design freedom versus conventional machining.
-
Integration of end-customer into design process like complex internal channels
-
Fast Prototyping through flexible and fast design adjustments
-
Mass customization
-
The unique use of lattice design can be useful to reduce the use of material or can be used where it is required without any constraints
-
Net shape process means less staple consumption, up to 25 times less versus machining.
-
Reducing assembly and dis-assembly operation by using net shape capability helps creating complex parts in one step.
-
No tools needed, unlike other conventional metallurgy processes
-
Short production cycle time: complex parts are often produced layer by layer during a few hours in additive machines.
-
More cost saving by reducing the use of raw material in the case of expensive or difficult to machine alloys
Below fig. shows the mapping of metal powder additive manufacturing technology
Fig 4. Mapping of main metal powder additive manufacturing technologies
-
-
Constraints of metal additive manufacturing process in aero gas turbine
-
Availability of the material chemical composition
-
Part size
-
Hugh investment
-
FAA certification:- The certification process for the components produced by this method still has to mature
-
Some of the undercuts in the parts where support is not feasible during AM process
Below fig. shows actual manufactured part using a Metal additive manufacturing process [10]
Fig 5. Bracket designed using Additive Manufacturing Technique
-
-
DESIGN OPTIMIZATION FOR AM TECHNOLOGY
-
Introduction:
To take full advantage of AM design possibilities, it is important to redesign conventional parts.
Design optimization can be done in several directions:
-
Reduce the total number of parts
-
Design for functionality
-
Design parts to be multifunctional
-
Lightweight
-
Topological optimization
-
Design for ease of fabrication
-
-
Case Study:
The example below shows a redesign case study of a bracket at reducing drastically the total weight using various AM software.
Fig 6. Initial geometry of the Model
Fig 7. Geometry of the Model after weight optimized
Inspire-Optistruct software was used to optimize the design and make the bracket suitable for Additive manufacturing technology.
Fig 8. Optimized Geometry from Inspire Software
-
Material Properties
Material
Titanium
Yield strength (at room temp)
128 ksi
Ultimate tensile strength (at room temp)
138 ksi
Youngs modulus
16500 ksi
Poisons ratio
0.342
Density
0.16
Table 1. Material Properties of case study bracket
Note: Used the same material properties [8] for initial geometry and for weight optimized geometry.
Optimization algorithm
Method of moving asymptotes
Objective function
(Default value)
Constraints
Kinematic coupling between bolt holes
Optimization algorithm
Method of moving asymptotes
Objective function
(Default value)
Constraints
Kinematic coupling between bolt holes
-
Boundary Conditions:
All DOF for bolt holes
Loads applied
8000 lb load in X, Y, Z direction
Table 2. Boundary Conditions applied for the case study
Fig 9. Kinematic couplings are used between bolt holes with reference point in order to simulate bolts and at the Clevis location for applying load
Fig 10. Bolt holes constrained in all DOF
Fig 11. Load of 8000 lbf is applied in Global X, Y and Z direction
Note: Boundary conditions and loading is the same for both the models and above are the pics corresponding to the initial geometry. [9]
-
Smax Principal
Structural finite element analysis is performed on the original bracket to observe the stress distribution in the part. Fig (12) shows the von Mises stress distribution for all the load cases. The areas shown in the blue color of the part replicates the inefficient use of material. This represents the negligible effect on the performance and still having the scope to optimize the geometry.
Note: The final design satisfies yielding condition with safety factor from 1.1 to 2.2
-
-
CONCLUSIONS
This paper has discussed the significance of using the Inspire-Optistruct software for design optimization and suitability for additive manufacturing process with a proper case study. The stress margin was within the yield strength and the weight reduction percentage was 70% (6.8lbs to 2.0lbs). Further research may be required on other gas turbine parts
ACKNOWLEDGMENT
The case study developed as part of an initiative taken at Cyient Ltd on Additive Manufacturing workshop with IIT Hyderabad and EOS Germany. Images are prepared by Cyient for illustration purposes only. Does not contain any customer information.
REFERENCES
Fig 12. Stress plots at different locations of bracket
Note: The maximum stress developed is 40.31 ksi which is well below the yield stress of 128 ksi
The redesined part has to sustain the same loads while fulfilling the same design requirements. The von Mises stress developed should be lower than the yield strength. Structural analysis has been carried out by using ANSYS software. As shown in Fig (13), the redesigned part satisfying the strength requirement for all load cases.
Fig 13. Stress plots at different locations of Redesigned bracket
-
Brooke, Rose. Additive Manufacturing Can Lower Aircraft Building and Operating Costs. TCT Magazine, 29 Oct. 2013, www.tctmagazine.com/additive-manufacturing-3d-printing- news/additive-manufacturing-can-lower-aircraft-building-and-oper.
-
Zhu JH,Gu XJ,Zhang WH and Beckers P, Structural design of aircraft skin stretch-forming die using topology optimization. Journal of Comput and App Math, 2013. 246: p. 278-88.
-
Deaton JD and Grandhi RV, A survey of structural and multidisciplinary continuum topology optimization: post 2000. Struct and Multidisci Optim, 2014. 49(1): p. 1-38.
-
Bendsøe, M. P., and Kikuchi, N., Generating Optimal Topologies in Optimal Design Using a Homogenization Method, Computer Methods in Applied Mechanics and Engineering, Vol. 71, No. 2, Nov. 1988, pp. 197224.
-
Shimels, G. H., Engida, W. D., & Mohd, H. F. (2017, October). A comparative study on stress and compliance based structural topology optimization. In IOP Conference Series: Materials Science and Engineering (Vol. 241, No. 1, p. 012003). IOP Publishing.
-
Adamski, Wodzimierz, and Janusz Michalcewicz. "Development of methods for designing and manufacturing aircraft components with additive technologies." Mechanik 93.3 (2020): 8-14.
-
"Additive Manufacturing Solutions & Industrial 3D Printer By EOS". Eos.Info, 2021, https://www.eos.info/en.
-
"Aerospace Metal Distributor." ASM Aerospace Specification Metals, Inc.| Florida Aerospace Metal Distributor, www.aerospacemetals.com/index.html.
-
""Autodesk Community." Autodesk Community, forums.autodesk.com/autodesk/.
-
Brooke, Rose. "Additive Manufacturing 'can Lower Aircraft Building and Operating Costs'." TCT Magazine, 29 Oct. 2013, www.tctmagazine.com/3d-printing-news/additive-manufacturing-can- lower-aircraft-building-and-oper/.