
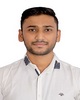
- Open Access
- Authors : Gaurav D. Patil , Atul M. Elgandelwar , Mandar M. Lele
- Paper ID : IJERTV10IS060162
- Volume & Issue : Volume 10, Issue 06 (June 2021)
- Published (First Online): 18-06-2021
- ISSN (Online) : 2278-0181
- Publisher Name : IJERT
- License:
This work is licensed under a Creative Commons Attribution 4.0 International License
Investigation on Lithium-Bromide and Water (Li-Br-H2O) Vapour Absorption Refrigeration System with Additive
Gaurav D. Patila, Atul M. Elgandelwarb, Mandar M. Lelec
aM.Tech Scholar, School of Mechanical Engineering,
b Associate Professor, School of Mechanical Engineering,
c Professor, School of Mechanical Engineering
Dr. Vishwanath Karad MIT-World Peace University Pune, Kothrud Pune (M.S), INDIA-411038.
Abstract:- This paper evaluates the mixture of Li-Br and potassium formate (CHO2K) as an alternative absorbents of vapour absorption system. The objective of this study is to investigate the performance improvement parameters of Li-Br vapour absorption refrigeration system by use of additive. To compare the performance of both the systems, thermal analysis of both the systems has been studied and influence of changing operating conditions has been analysed numerically and graphs has been plotted. Analysis of results has shown the efficiency of the system is increased by 16%. Hence, by use of potassium formate the heat requirement in the absorber and generator is dramatically decreased and therefore, an improvement in the efficiency of the system has been achieved.
Keywords: Refrigeration system, Lithium bromide, Potassium formate, Absorption system
-
INTRODUCTION
At the early stages, vapour absorption refrigeration system was widely used for refrigeration but because of its low co- efficient of performance, vapour compression refrigeration cycle took its place. Nowadays, due to increasing global warming the environmental activist are focusing on developing an alternative of VCRS system with almost similar or more co-efficient of performance. A popular alternative is an absorption system. It can operate at low grade heat or the heat produces from turbine exhausts, solar plant, industrial process etc. It can be useful where there is no supply of electricity or electricity is very costly.
The absorption system mainly uses two mixture combination:
-
Ammonia water: Ammonia is used as a refrigerant in the system while water acts as an absorber. This system finds its application in commercial plants. This system is not useful in domestic air conditioning because of its toxicity.
-
Li-Br and water: Water is used as a refrigerant and lithium bromide as an absorber. This system is widely used for air conditioning applications. The Li-Br system requirements are less than ammonia- water system which makes its system maintenance easy.
Potassium formate possess low vapour pressure which is helpful in working at lower temperature [1]. The crystallization temperature, density and viscosity are various properties of potassium formate which makes its a prominent additive for selection [2]. Potassium formate easily gets absorbed at low temperature than lithium bromide [3]. A new absorbent mixture of Li-Br + CHO2K is proposed [5]. The values of vapour pressure, density and viscosity are obtained experimentally [6]. The major drawbacks of using lithium bromide alone is that it crystallises at high concentrations and lower absorber temperature. Therefore, an anti-crystallization additive should be used to overcome this crystallization problem. Potassium formate as an additive is used in this paper because of its physical properties to overcome crystallization problem which eventually helps in increasing systems performance.
-
-
WORKING OF VARS CYCLE The Li-Br refrigeration cycle is shown in fig.1
As we can see the liquid refrigerant coming from evaporator has low temperature as well as low pressure reaches absorber. Simultaneously, the strong solution from generator reaches absorber. In absorber mixing of liquid refrigerant and strong solution takes place and this is again pumped back to generator. Pump delivers high pressure weak solution to generator where the refrigerant drives off to condenser after receiving heat from the source. The Condenser condenses high pressure refrigerant into liquid and then it is allowed to enter into throttling valve. After getting throttle, the refrigerant enters evaporator where refrigerant changes its phase from liquid to vapour after absorbing heat load, thus producing refrigeration effect. The vapour refrigerant again enters absorber and cycle gets repeated.
Fig. 1: Diagram of Lithium-Bromide Absorption System.
-
ANALYSIS
Following are the design considerations for the system under operation:
-
Enthalpy of sat. Liquid leaving condenser, H8 = (TE 25) x 4.184 (3)
-
Enthalpy of sat. Water vapour,
Sr. No.
Components
Operating Range
1
Evaporator
5ºC
2
Condenser
20 to 50ºC
3
Absorber
5 to 50ºC
4
Generator
60 to 120ºC
5
Refrigeration Capacity
5.25 kW
6
Effectiveness of Heat Exchanger
0.65
7
Refrigeration Solution
-
Li-Br
-
Li-Br + CHO2K
Sr. No.
Components
Operating Range
1
Evaporator
5ºC
2
Condenser
20 to 50ºC
3
Absorber
5 to 50ºC
4
Generator
60 to 120ºC
5
Refrigeration Capacity
5.25 kW
6
Effectiveness of Heat Exchanger
0.65
7
Refrigeration Solution
-
Li-Br
-
Li-Br + CHO2K
Table 1: Operating conditions of Li-Br system
H10
= (572.8 + 0.417 TE) x 4.184 (4)
Thermal analysis of both the systems is carried through following procedure:
(1) Concentration of strong solution,
-
Refrigerant mass flow rate,
MR =
108
-
Strong solution mass flow rate,
M s = 4
(41)
-
Weak solution mass flow rate,
Mw = 1
(41)
(5)
(6)
(7)
XSS OR X1 = 49.05+1.125
134.65+0.47
(2) Concentration of weak solution, XWS OR X4 = 49.04+1.125
134.65+0.47
(1)
(2)
-
Temperature at state 5,
T5 = TG ESHX (TG TA) (8)
-
Specific heat of strong solution,
CX1 = [1.01 – 1.23 X1 + 0.48 (X1)2] x 4.184 (9)
-
Specific heat of weak solution,
CX4 = [1.01 – 1.23 X4 + 0.48 (X4)2] x 4.184 (10)
-
Temperature at state 3,
T3 = T3 + [ESHX x 1 x 4 x (TG – TA)] (11)
4 4
-
Enthalpy at state 1,
QC = MR (H7 HG) (15)
-
Heat capacity of generator,
QG = (MWH5 + MRH7 MGH1) (16)
-
Heat capacity of absorber,
QA = (MWH5 + MRH10 M8H1) (17)
H1 = [42.81 425.92 X1 + 404.67 (X1)2] + [1.01 – 1.23 X2
+ 0.48 (X2)2] x (TA) (12)
-
Enthalpy at state 5,
H5 = [42.81 425.92 X4 + 404.67 (X4)2] + [1.01 – 1.23 X4
+ 0.48 (X4)2] x (T5) (13)
-
Enthalpy at state 7,
H7 = (572.8 + 0.417 TG – 0.043 TE) * 4.184 (14)
-
eat capacity of condenser,
The pressure of evaporator and condenser is calculated by
-
Co-efficient of performance,
COP =
-
Maximum COP,
(COP) MAX = ( +273.15) ( )
+273.15) ( )
-
Relative performance ratio,
=
-
Evaporator pressure, PEVA=antilog
(18)
(19)
(20)
the equation given by Lasing [7].
4
4
-
Condenser pressure, PCOND = antilog
( 273.15)
11.2414 10 ]
4
4
(+273.15)2
[7.855 1555( 273.15)
11.2414 10 (+273.15)2
components on the efficiency of both the system has been shown below:
-
-
RESULTS AND DISCUSSION
The new absorbent mixture of Li-Br + CHO2K absorption system is compared with lithium bromide absorption system under identical conditions. Co-efficient of performance of both the systems is compared by generator temperature, absorber temperature, condenser temperature at varied operating conditions were carried out and an increase of 16% in the efficiency of the system has been achieved with the new absorbent mixture.
The addition of potassium formate in the mixture dramatically decreased the heat requirement in the generator section and provides lower crystallization temperature. The impact of varying operating conditions of different
-
Co-efficient of Performance (Cop) v/s Generator Temperature
Fig 2. Shows the variation of performance of Li-Br system with respect to generator temperature. The maximum COP
= 0.789 is obtained when the Generator temperature is kept 85 OC (Keeping all other system parameters as constant). Further increasing the generator temperature COP tends to decrease because of increasing weak solution concentration, which initiates crystallization.
The variation of performance of the Li-Br + CHO2K system with respect to generator temperature is shown in fig.2 The maximum COP = 0.915 is obtained when the Generator temperature is kept at 65OC without crystallization problem.
64 65 70 75 80 85 90 95 100 105 110 115 120
Generator Temperature TG (OC)
64 65 70 75 80 85 90 95 100 105 110 115 120
Generator Temperature TG (OC)
Without additives
With additives
Without additives
With additives
1
0.95
1
0.95
0.914
0.914
Co-efficient Of Performance (Cop) Vs Generator Temperature TG
(OC)
QE = 5.25 KW, TE = 5
OC
Co-efficient Of Performance (Cop) Vs Generator Temperature TG
(OC)
QE = 5.25 KW, TE = 5
OC
0.915
0.915
0.906
0.906
0.898
0.898
0.89
0.89
0.9
0.9
0.883
0.883
0.875
0.875
0.868
0.868
0.861
0.861
0.854
0.854
0.847
0.847
0.841
0.841
0.85
0.85
0.835
0.835
0.8
0.75
0.8
0.75
0.777
0.777
0.787
0.787
0.789 0.787 0.783
0.789 0.787 0.783
0.779
0.779
0.773
0.773
0.768
0.768
0.762
0.762
0.7
0.7
0.65
0.6
0.65
0.6
0.738
0.695
0.654
0.738
0.695
0.654
0.757
0.757
0.55
0.5
0.55
0.5
Co-efficientOf Performance, (Cop)
Co-efficientOf Performance, (Cop)
Fig. 2: Graph of Co-efficient of Performance (Cop) V/s Generator Temperature TG (OC) of Li-Br System.
-
Co-efficient of Performance (Cop) v/s Absorber Temperature
Fig 3. Shows the impact on performance of Li-Br system with respect to absorber temperature. The maximum COP = 0.808 is obtained at 19.95OC absorber temperature. We can see from the graph that higher absorber temperature is not suitable for the system.
The impact on performance of the Li-Br + CHO2K system with respect to absorber temperature is shown in fig 3. The maximum COP = 0.854 is obtained at 15OC absorber temperature. Increasing the absorber temperature COP tends to decrease because the concentration of strong solution increases.
Co-efficientof Performance, (Cop)
Co-efficientof Performance, (Cop)
Co-efficientof Performance (Cop) Vs Absorber Temperature, TA (OC)
QE = 5.25 KW, TE = 5 OC
1
0.95
0.9
0.84 0.847 0.854 0.845 0.837 0.828 0.819
TC = 35 OC , TG = 90 OC
0.85
0.8
0.75
0.7
0.835 0.826 0.817
0.808 0.798 0.787 0.773
0.811 0.803 0.794
0.754
0.65
0.6
0.55
0.5
0.721
0.622
5 10 15 20 25 30 35 40 45 50
Absorber Temperature, TA (OC)
Without additives With additives
Fig. 3: Graph of Co-efficient of Performance (Cop) V/s Absorber Temperature TA (OC) of Li-Br System
-
Co-efficient of Performance (Cop) v/s Condenser Temperature
Fig 4. Shows the impact of condenser temperature on performance of Li-Br system. The maximum COP = 0.805 is obtained when the condenser temperature is kept 20 OC. Increasing the condenser temperature, concentration of
weak solution increases which results in the decline of the system performance.
The impact of condenser temperature on performance of the
Li-Br + CHO2K system is shown in fig 4. The maximum COP
= 0.885 is obtained when the condenser temperature is kept 20 OC.
Co-efficientOf Performance, (Cop)
Co-efficientOf Performance, (Cop)
Co-efficient of Performance (Cop) Vs Condenser Temperature, TC (OC)
0.97
0.93
0.89
0.85
0.81
0.885 0.882 0.879
QE = 5.25 KW, TE = 5 OC TA = 30 OC , TG = 90 OC
0.875 0.871 0.867 0.862
0.77
0.73
0.69
0.65
0.805 0.801 0.795 0.787
0.774
0.752
0.705
20 25 30 35 40 45 50
Condenser Temperature, Tc ( OC )
Without additives With additives
Fig. 4: Graph of Co-efficient of Performance (Cop) V/s Condenser Temperature TC (OC) Of Li-Br System
-
-
CONCLUSION
A new working absorbent pair of Li-Br and CHO2K has been presented in this paper and its performance has been compared with the Li-Br system under identical conditions. The analysis of results shows that, the efficiency of the system is increased by 16%.
Lower absorber temperature up-to 5OC is obtained without crystallization problem. The heat requirement in the generator section is decreased drastically and the maximum COP=0.915 is obtained at lower generator temperature of 65
OC.
Potassium formate being less expensive and less corrosive to metals forms the best pair with lithium bromide to be used as an absorbent mixtures.
Li-Br |
Lithium Bromide |
M |
Mass flow rate (kg/s) |
RE |
Refrigeration effect(kW) |
T |
Temperature (ºC) |
X |
Li-Br mass fraction in Li-Br/Water solution (%) |
Effectiveness of solution heat exchanger |
|
P |
Pressure of the system (kPa) |
W |
Work done |
Kinematic viscosity (m2/s) |
|
H |
Enthalpy at state point (kJ/kg) |
Li-Br |
Lithium Bromide |
M |
Mass flow rate (kg/s) |
RE |
Refrigeration effect(kW) |
T |
Temperature (ºC) |
X |
Li-Br mass fration in Li-Br/Water solution (%) |
Effectiveness of solution heat exchanger |
|
P |
Pressure of the system (kPa) |
W |
Work done |
Kinematic viscosity (m2/s) |
|
H |
Enthalpy at state point (kJ/kg) |
Subscripts
Nomenclature
A: |
Absorber |
R: |
Refrigerant |
X1: |
strong solution |
X2: |
weak solution |
G: |
Generator |
In: |
entering |
Out: |
exiting |
110: |
Mentioned Position |
E: |
Evaporator |
C: |
Condenser |
REFERENCES
-
Kardos P, Ka´lma´n T, Kerii J, Kerii E. Coolant composition containing potassium formate and potassium acetate and method of use. 1992 [US Patent no.-+5104562].
-
Riffat S, James S, Wong W. Experimental analysis of the absorption and desorption rates of HCOOK/H2O and LiBr/H2O. International Journal Energy Res 1998; 22: 1099103.
-
Kim JS, Lee H, Yu S. Absorption of water vapour into lithium bromide based solutions with additives using a simple stagnant pool absorber. International journal of refrigeration 1999;22 : 188 93
-
Antonio De Lucas, Marina Donate, and Juan F. Rodrguez, Vapour Pressures, Densities, and Viscosities of the (Water
+Lithium Bromide + Sodium Formate) System and (Water + Lithium Bromide+ Potassium Formate) System, Journal of chemical Engineering. Data 2003, 48, 18-22
-
A. De Lucas, M. Donate, C. Molero, J. Villasenor, J.F. Rodriguez, Performance evaluation and simulation of a new absorbent for an absorption refrigeration system, International journal of refrigeration 27 (2004) 324330.
-
A. De Lucas, M. Donate, J.F. Rodriguez, Vapour pressures, densities, and viscosities of the (water+lithium bromide+sodium formate) system and (water+lithium bromide+ potassium formate) system, Journal of Chemical Engineering Data 48 (2003) 1822.
-
Lansing, FL. (1976). Computer modeling of a single-stage lithium bromide/water absorption refrigeration unit. The Interplanetary Network Progress Report.
-
Khan JR, Zubair SM. Design and performance evaluation of reciprocating refrigeration systems. International journal of refrigeration 1999;22: 23543.
-
Engler M, Grossman G, Hellmann HM. Comparative simulation and investigation of ammoniawater: absorption cycles for heat pump applications. International journal of refrigeration 1997; 20:50416.
-
Refrigerating American Society of Heating and Air-Conditioning Engineers. 2009 ASHRAE handbook: Fundamentals. Atlanta: ASHRAE Engineers; 2009.
-
Donate, M.; Rodriguez, L.; De Lucas, A.; Rodrguez, J. F. Thermodynamic evaluation of new absorbent mixtures of lithium bromide and organic salts for absorption refrigeration machines. International journal of refrigeration. 2006, 29, 30.
-
Atkinson S. Vapour absorbent compositions comprising potassium formate. US Patent n° 5846450, 1998
-
Iyoki, S.; Iwasaki, S.; Kuriyama, Y.; Uemura, T. Densities, Viscosities, and Surface S. Tensions for the Two Ternary Systems H2O + LiBr + LiI and H2O + LiCl + LiNO3. Journal of Chemical Engineering Data 1993, 38, 302-305.