
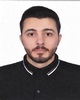
- Open Access
- Authors : Awda Youssef M , Habiballah Ibrahim O
- Paper ID : IJERTV10IS120040
- Volume & Issue : Volume 10, Issue 12 (December 2021)
- Published (First Online): 20-12-2021
- ISSN (Online) : 2278-0181
- Publisher Name : IJERT
- License:
This work is licensed under a Creative Commons Attribution 4.0 International License
The Need for Smart Grid under the vision 2030 for the Kingdom of Saudi Arabia: An Overview
Awda Youssef M
Department of Electrical Engineering
King Fahad University of Petroleum and Minerals Dhahran, Saudi Arabia
Habiballah Ibrahim O
Department of Electrical Engineering
King Fahad University of Petroleum and Minerals Dhahran, Saudi Arabia
AbstractThis paper concisely discusses the revolution of the grid system that is going to take place in the Kingdom of Saudi Arabia (KSA) under vision 2030. In addition, it will represent an overview of the smart grid as well as its advantages over the existing traditional grid, i.e., fossil fuel based. Moreover, this paper will discuss the development and research challenges those are associated with the integration of renewables in the current conventional grid.
KeywordsRenewable Energy, Smart Grid, Vision 2030, SCADA, IBR.
Consequently, in order to achieve the NREPs target, the traditional grid needs to be transformed into a smart grid in which its structure is shown in fig. 3. Shifting to the smart grid is fraught with a lot of research and development challenges. This paper outlines the features of the smart grid and their corresponding challenges.
-
INTRODUCTION
The Kingdom's unique geographical and climatic location makes renewable energy sources commercially viable, bolstering Saudi attempts to diversify the country's energy mix. Therefore, Saudi Arabia has introduced The National Renewable Energy Program (NREP) which seeks to raise the Kingdom's share of renewable energy generation, achieve a balance in the mix of local energy sources, and meet the Kingdom's carbon-reduction commitments. Recently, Saudi Arabia has set a target of 58.7 GW of renewable energy projects by 2030, comprising 40 GW of solar PV, 16 GW of wind, and
2.7 GW of other renewable energy sources, as illustrated in fig.
1. Saudi Arabia issued a tender for 300 MW of solar PV in Sakaka and 400 MW of wind power in Dumat Al-Jandal to meet this goal in the 2018 Renewable Energy Project Development Office (REPDO). Eleven pre-developed solar PV projects with a total capacity of 2.225 GW are in the tendering process for 2019, as shown in fig. 2 [1].
Fig. 1: Adjusted targets of KSA Vision 2030 for renewables.
Fig. 2: New PV projects scheduled to bid in 2019.
Fig. 3: Basic structure of the smart grid.
-
LITERATURE REVIEW
For the past years, the smart grid has intrigued many researchers. For instance, Florian Hertrampf et al. 2018, proposed a work to optimize local power generation by ensuring that the local grid is completely utilized and consumed locally. This recoded the effects of concentrating devices like photovoltaic (PV) solar systems and charging arrangements for electric vehicles and different home appliances, with or without smart grid capabilities. They sought to use a non-hard real-time processing system to maximize the use of local devices and manage the real-time consumption scenario in this project. The architectural design of the significant data processing component WDL is illustrated in this paper. This was created with both functional and non-functional considerations in mind. Electric drives and the specific properties of EVs were integrated, as were different communication technologies. Grid hierarchy was used to classify the research, and additional research was conducted by referring to local smart grids [2].
Roger N. Anderson et al. 2018, have suggested a project titled "Smart Grid: The Future of Electrical Energy System". This study has wonderfully articulated the need for computational intelligence in the smart grid. The complete spectrum of the power system was detailed in this paper, including generation, distribution, consumption, and feedback loops, in order to create the smart grid and its essential features. According to the study, consumer buy-in customers must
witness genuine preservation and competency gains, which quickly described the smart grid's problems and potential successful elements [3].
Girish R. et al. 2019, proposed an improved switched capacitor based on multilevel inverters (MLIs) as an alternative to the lossy, bulky, and expensive classical inverters used to link DC power source to AC grid. MLIs offer a higher level of control, decreased total harmonic distortion, and less inductor design. On the other hand, MLIs are beneficial in reducing the filtering of the distributed sources of the smart grid at common coupling points. In general, the proposed topology eliminated the for-semiconductor devices such as auxiliary circuits and voltage sensors. However, a reduction of 5.8% in total harmonic distortion is achieved. The design is modulated and validated utilizing the PODPWM technique and the simulation [4].
Uddin R. et al. 2019, worked on a proposal for "Fault Detection and Service Restore System in a Smart Grid." The goal of the project was to develop a DMS (Distribution Management System) using SCADA and the FDIR (Fault Detection, Isolation, and Service Restoration) technology, which is widely used in smart grids. The Markov model was used to examine FDIR behavior in a smart grid distribution network. When FDIR is used in a wired communication system, the failure probability of different distribution components is predicted. The analysis and prediction in this work were made so successfully that the isolation probability at which the load switches of the distribution network separate themselves when a malfunction in the wireless communication network occurs was increased. In addition, the chance of recovering the system through non-active switches in wired and wireless communication networks was predicted as anticipated. They also looked at the failure, isolation, and restoration probability of smart grid components [5].
Yousef E.M. et al. 2020, suggested work on Automated Home Management for the smart grid system, which produced better outcomes than previous theories. This paper discusses how to control a smart grid of electric loads in real-time using an ICT idea (Information and Communication Technology). The automated home management system aids in the regulation of electric load scheduling. For managing and controlling the power, a mix of hardware and software is used efficiently. Microcontroller, electric current and voltage sensors, and Ethernet module are some of the hardware components. The Smart Electrical Task Scheduling (SETS) algorithm is used to schedule electrical demands in the second stage. The fundamental goal of this project was to create an energy management system that could intelligently regulate electric demands at home, with the smart grid playing an essential part [6].
solution is to utilize satellites telemetry terminals as a contingency or main communication channel by integrating monitoring middleware and Distribution Operation Center (DOCs) in the cloud with metering and protective devices. This technology has been in use for 5 years in 1500 point located around Latin America and the main electrical companies in Brazil. The data indicated an improvement in SAIFI and SAIDI which enable the electrical companies to make a real time strategical decision and better managing of the installed devices in the field. Furthermore, this technology eliminated the need for specialized teams at the site for equipment installation and mating time. In brief, the distribution companies' need has been fully met by implementing this solution as no loss of communication between measurement and field-based protection equipment and the DOC [7].
Joeph Kamto et al. 2011, shared their thoughts on the topic of 'Light-Weight Key Distribution and Management for Advanced Metering Infrastructure.' AMI (Advanced Metering System) is a critical component of the smart grid for analysis and assessment. It improves the smart grid security system by utilizing crypto algorithms while also protecting the user's privacy and authenticity. The AMI was employed in this work to focus on a lightweight distribution and management system. By managing HAN (Home Area Network) to BAN (Building Area Network), as well as NAN (neighborhood Area Network) and WAN, the integrated form of ICT (Information and Communication Technology) is employed. For our Future grids, i.e., Smart grids, these cryptographic processes produce the needed outcomes for light weight distribution [8].
-
SMART GRID
Burian R. et al. 2019, presented a new technology based on satellite telemetry connectivity to control and monitor installed protection equipment, measurement systems, and reclosers on the electrical networks. This technology aimed to overcome the problem of achieving highly reliable and available communication channels connecting the field-installed devices to SCADA installed on utilities DOC in remote regions where telecommunication coverage is unstable or unavailable. Large power cooperatives and concessionaires faced a techno- financial market differential in such areas. The idea behind this
Fig. 4. The main differences between conventional and smart grids [9].
The traditional electrical networks were built a century ago due to the lack of high-level automation and communication inputs, with relatively simple power generation, transmission, and distribution conceptions. Then came the conventional grid (the current grid system in Saudi Arabia), which has a one-way power flow (From energy generation to energy consumption). Creating a grid from a traditional network that meets all the
average variance in power consumption requirements in a real- time period is challenging. It is required to upgrade the existing system by gathering diverse components such as voltage and current sensors, fault detectors, and two-way communication networks, among others. As a result, future grids will be able to provide a notion of bi-directional energy flow and
communicational data and control power networks. Table 1 and Fig. 4 depict a comparison between a traditional grid and a smart grid to provide a good understanding of smart grid ideas [10].
TABLE I. ADVANTAGES OF SMART GRID OVER THE CONVENTIONAL GRID
S.No.
Characteristics
Conventional Grid
Smart Grid
1.
Technology
Electromechanical:
Previously, the energy
infrastructure was
electromechanical. In other words, mechanical machines are electrically controlled. This technology lacks communication capabilities and is governed by ineffective internal regulations.
Digital:
The smart grid is made up of digital technology that allows for self-regulation and can be remotely controlled.
2.
Power Flow
Unidirectional:
Conventionally, power was distributed in just one direction, from the primary generation plants to the distribution network.
Bidirectional:
Here, the smart grid-enabled technology improves power distribution through the main power plant, and it also becomes bilateral with the aid of an auxiliary supplier, allowing power to be returned to the main plant. The electrical energy can be returned to the grid by integrating PV-based solar panels or other secondary energy sources. This method promotes the use of renewable energy.
3.
Generation
Centralized:
The electrical power in a typical system was generated centrally. This maintains the drawback of not being able to connect other energy sources to the supply system as and when needed.
Spread:
Power distribution is enabled in this system using numerous plants and several substations, which aid in load balancing, reduce peak time transients, and significantly reduce power shortages.
4.
Detection
Limited capability:
Few sensors cannot be connected to the power lines in this system. Therefore, identifying the specific point of failure becomes more difficult. With lengthy downtimes, it is possible to get the desired result.
High-level technology:
The smart grid system's power lines are equipped with various sensors that aid in detecting the exact location of a defect so that electricity may be diverted to the appropriate location. As a result, the system may be secured without causing too much downtime in the healthy parts.
5.
Tracking
Manually:
Due to several restrictions in the prior grid system, energy distribution monitoring was traditionally done.
Autonomous:
We have the capability of digitally controlled self-monitoring of energy distribution in the smart grid. This aids in load balancing, outage troubleshooting, and general distribution system management without the need of any external sources.
6.
Restoration
Hand-operated:
Repairing things in the traditional way. This approach entails technicians physically moving to the source of the breakdown and repairing it. As a result, the procedure takes a long time.
Self-Healing:
Sensors can diagnose and repair issues without the need for human interaction. In the event that the framework is damaged, this system features a mechanism that immediately alerts the specialists in the control room, allowing them to take rapid action to fix the damage.
7.
Equipment
Failures & Outages:
Traditional grids have been increasingly vulnerable to breakdowns over time. Blackouts are a serious condition that occurs when there is no power remaining at the consumption point due to a malfunction in the system.
Adaptive & Islanding:
The power flow in a smart grid system can be controlled under any fault location. This helps to avoid the blackout of that specific location.
8.
Controllability
Bounded:
Since this conventional technique is manual, controlling the full energy level becomes challenging. The distribution of power cannot be regulated by multiple agencies once it has been sent.
Prevalent:
With modern sensors and other actively controlled devices, the Smart Grid system has precise control over the flow of electricity from the first stage of transmission to the final stage of distribution to the consumer end.
9.
Consumer Choices
Scant:
The previous power grid infrastructure was unable to provide consumers with a variety of energy delivery options. It was restricted and unsuccessfully satisfied the customers needs.
Plenty:
Sharing is now possible because of smart grid technologies. It features a partnership of many firms, energy resources, and innovative control systems to deliver more alternative possibilities to energy recipients till they are satisfied.
Fig. 4 shows that in a traditional grid, electricity is supplied utilizing the conventional method following the same generation-transmission-distribution pattern with centralized electricity market. Then this distributed power is delivered to various consumption points such as factories, villages, and homes using multiple methods (including overhead lines and underground cables). On the other hand, the smart grid uses information technology and automation systems, as well as smart meters and controllers, to create an integraed power system that increases efficiency, lowers costs, and improves customer satisfaction and confidence. The whole power system is controlled from a specific location, i.e., the control center.
-
IMPACT OF SMART GRID ON POWER SYSTEM STABILITY Ensuring the stability of the electrical grid is not a new
challenge. However, due to high penetration of inverter-based recourses (IBRs) in smart grid, the stability phenomena, both new and existing, are revealing themselves. This section covers the challenge of frequency stability in the smart grid.
In a typical power system combined with synchronous generators, the initial rate of change of frequency (RoCoF) is calculated based on the inertial response after contingency. However, Before the protective system (e.g., over frequency generation reduction and under frequency load shedding) reacts, the response of the generator governor helps in holding the frequency of the system. Meanwhile, frequency restoration and stabilization are accomplished using an additional reserve. On the other hand, a higher RoCoF after a contingency may result from displacing the inertial response of the displacing synchronous machines such as IBRs. This could result in frequency limits exceedance. The latter, provides no time to countermeasures of the protective machines. Hence, they may shut down the load or generation. In addition, Protective devices actuated by a high RoCoF may provoke these effects. Therefore, state-of-the-art IBRs is used to provide frequency control as they have rapid response time compared to the synchronous generators governor. Overall, aggressive controls, on the other hand, may create frequency oscillations and other sorts of instability in low-inertia systems. System operators need to continuously revise the reserve amount and performance of the frequency response as the characteristics of the power system are changing [11].
There are other crucial factors for frequency stability, namely, load contingency and the largest generation. While IBRs sizes are smaller than synchronous generators, a new failure mode may appear as a high resources share is done. For instance, voltage-induced frequency dips may appear in a geographically compact system resulting from wider-area low- voltage propagation during faults. An appropriate mitigation measure needs to be determined for such events. System split or a high RoCoF after a contingency may restrict IBR maximum instantaneous penetration unless a sufficient degree of inertia is maintained. One option is to maintain several must- run synchronous generators. However, synchronous generators (particularly gas turbines) can be sensitive to large RoCoF and may not alleviate the risk. Synchronous condensers (SynCons) and IBRs can also supply the required inertia. While SynCons have low inertia, flywheels can be added to boost their contribution. The available energy buffer and inverter overcurrent capabilities influence the inertial response of an IBR [11].
A proposed solution is to use energy storage to aid the frequency response of the power system which is functional to regulate the frequency in high penetration of IBRs. The discharging length and profile of energy-constrained with high- power-density energy storage could be adjusted for best control performance [12].
-
IMPACT OF SMART GRID ON POWER SYSTEM PROTECTION
One of the most common challenge that smart grid faces while considering the protection and control system is limited fault current. In this section, the inverter fault current limitation is discussed among with the proposed solution.
The total fault current produced by a synchronous generator against a continuous inverter with the same rating can be illustrated in Fig. 5. The fault current generated by generators varies dynamically with fault duration. Extremely high currents define the initial few cycles of a fault, followed by relatively lower currents. By assuming artificial impedances behind an ideal voltage source. Protection engineers may simplify that as transient reactance () and subtransient reactance (). In a typical synchronous generation-driven power system, the transient reactance is the parameter used to coordinate most protective relays.
Inverter currents during faulted-circuit situations are very different from synchronous generator currents, as shown in Fig.
5. Inverter-generated fault currents can be divided into two categories: 1) silicon's short-term constraints, and 2) the heat sinks and thermal management control's longer-term thermal restrictions. Following the silicon time, inverters drop their current from the silicon to the thermal limit. After prolonged operation at the long-term thermal limit, certain inverters stop commutating. Others lower voltage to keep current within limits and trip only if the resultant voltage falls below permissible levels [13].
Fig. 5: The fault current of synchronous generator Vs. Inverter [13].
When silicon current restrictions are exceeded, power electronic devices are irrevocably ruined. As a result, the inverters' firmware strongly controls this current, which is why the silicon limit is also known as a hardware or surge limit. Therefore, real-time readings of aluminum heat sinks and cooling water jackets are widely used to modify thermal limitations in inverter firmware. Because heat-removal
hardware is costly, the thermal limit is frequently the determining factor in overall inverter building costs [13].
As a suggested solution, the circuit breaker (CB) functioning can be determined using both voltage and current profiles. The bus voltage will be checked after the current exceeds the specified value. The problem location might be approximated by the relay by selecting the voltage range. The time function is linked to the voltage range. As a result, the CB might play a crucial role as a main or backup protection. A three-dimensional current-voltage-time curve can be used to assess this approach [14].
-
CONCLUSION
This paper presented an overview of KSA vision 2030 concerning the enhancement of the conventional grid in Saudi Arabia by integrating various types of renewable energy. In addition, a review of the main features of the smart grid and a comparison between the conventional grid and smart grid were introduced. Finally, some of the main development and research challenges associated with power system stability and protection were discussed while considering the impact of the smart grid on the current conventional grid.
ACKNOWLEDGMENT
The authors acknowledge the support of King Fahad University of Petroleum and Minerals (KFUPM) for giving all the necessary resources to complete this paper.
REFERENCES
-
national transformation program, Vision 2030, 2019. [Online].
-
Florian Hertrampf, Sebastian Apel and Steffen Späthe Requirements and Architecture Concept for a Data Processing System in Local Area Smart Grid Journal of Electrical Engineering 6 (2018) 1-10.
-
AndersonRoger &Ghafurian, Reza &Gharavi, Hamid. Smart Grid The Future of the Electric Energy System IEEE Explore, June 2018.
-
R. Girish Ganesan, R. Shankar, M. Bhaskar and K. Narayanan, "Switched Capacitor Based Multilevel Boost Inverter for Smart Grid Applications," 2019 IEEE Innovative Smart Grid Technologies – Asia (ISGT Asia), 2019, pp. 3902-3907, doi: 10.1109/ISGT- Asia.2019.8881197.
-
Uddin. R., Alghamdi, A.S., Uddin, M.H. et al. Ethernet-Based Fault Diagnosis and Control in Smart Grid: A Stochastic Analysis via Markovian Model Checking. J. Electr. Eng. Technol. 14, 22892300 (2019).
-
Yousef E. M. Hamouda&Sohib J..Dwedar Optimally Automated Home Management for Smart Grid system using sensors,springer nature singaporepte ltd,13 Aug 2020.
-
R. Burian, M. Gontijo and H. Alvarez, "Robustness and Reliability in Smart Grid Solutions," 2019 IEEE 7th International Conference on Smart Energy Grid Engineerng (SEGE), 2019, pp. 59-62, doi: 10.1109/SEGE.2019.8859947.
-
Joseph Kamto, Lijun Qian, John Fuller, John Attia Light-weight Key Distribution and Management for Advanced Meteringinfrastructure, Dec 2011.
-
https://commons.wikimedia.org/w/index.php?curid=69505750 By Bartz/Stockmar, CC BY-SA 4.0. [online].
-
S. Tripathi, P. K. Verma and G. Goswami, "A Review on SMART GRID Power System Network," 2020 9th International Conference System Modeling and Advancement in Research Trends (SMART), 2020, pp. 55-59, doi: 10.1109/SMART50582.2020.9337067.
-
J. Matevosyan et al., "A Future With Inverter-Based Resources: Finding Strength From Traditional Weakness," in IEEE Power and Energy Magazine, vol. 19, no. 6, pp. 18-28, Nov.-Dec. 2021, doi: 10.1109/MPE.2021.3104075.
-
S. You et al., "Energy Storage for Frequency Control in High Photovoltaic Power Grids," IEEE EUROCON 2019 -18th International Conference on Smart Technologies, 2019, pp. 1-6, doi: 10.1109/EUROCON.2019.8861993.
-
S. Manson and E. McCullough, "Practical Microgrid Protection Solutions: Promises and Challenges," in IEEE Power and Energy Magazine, vol. 19, no. 3, pp. 58-69, May-June 2021, doi: 10.1109/MPE.2021.3057953.
-
V. R. Mahindara, A. Priyadi, M. Pujiantara, M. H. Purnomo, A. Y. Saber and E. Muljadi, "Protection Coordination Challenges for Microgrid Distribution Network with High Penetration Inverter-Based Resources," 2020 IEEE Energy Conversion Congress and Exposition (ECCE), 2020, pp. 1618-1622, doi: 10.1109/ECCE44975.2020.9235817.