
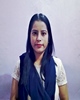
- Open Access
- Authors : Rimpi Borah , Vikas Patel , Surangana Baruah
- Paper ID : IJERTV11IS010196
- Volume & Issue : Volume 11, Issue 01 (January 2022)
- Published (First Online): 07-02-2022
- ISSN (Online) : 2278-0181
- Publisher Name : IJERT
- License:
This work is licensed under a Creative Commons Attribution 4.0 International License
Performance Analysis of Different Diversity Technique for Wireless Communication
Rimpi Borap
M.Tech, Department of Electronics and Communication IIIT Guwahati, India
Surangana Baruap
-
ech, Department of Electronics and Communication Gauhati University, India
Vikas Patel2
M.Tech, Department of Electronics and Communication NIT Sikkim, India
Abstract— Given that the required wireless equipment is small and bandwidth-constrained, there are many significant challenges in wireless networks which include signal loss, mobility, increased data rates, reduced size and cost, user security, which can directly or indirectly effect on overall quality of service (QoS) of the entire communication system. Different techniques are used to solve these intricacies There are several methods which can be used to overcome the mentioned channel vulnerabilities. While equalization is used to counteract ISI results, channel asymmetry is used more to reduce the depth and duration of the fade encountered by the receiver in a flat fading or in time dispersive channel. Again channel coding can also improve the error performance of any system by applying the concept of redundancy. Various procedures also have drawbacks. We can have more satisfying diversification methods by examining and weighing the existence of the system. This is an introductory paper on different diversity techniques which can be used to compensate fading channel impairments by using multiple antennas at the receiver side. The ubiquity of receiver asymmetry is a tricky problem – how do we 'efficiently' exercise all antennas to demodulate the data. There are various steps to accost the intricacy. Three different strategies are considered – selection diversity, equal gain combining and maximum ratio combining technique. In this paper, we will talk about these three techniques and will analyze their performance individually. The simulation outcomes show that by applying this technique the bit error rate diminishes with a rise in energy per bit of noise spectral density (E b/n o) that is if there is a deep fade in one radio path, there may be a stronger signal in the other independent path. With more than one path to choose from, both the instantaneous and average SNR at the receiver can be improved, often from 20 dB to 30 dB.
KeywordsFading (small-scale and large-scale fading), Diversity, Equal gain combining, Maximal gain combining, Eb/No.)
-
INTRODUCTION
Fading is one of the main challenges faced by any wireless communication link. Fading refers to the undesirable changes occurs on the characteristic of the transmitted signal. This changes generally happens due to the inherent characteristic of the channel for example in the flat fading channel which are usually amplitude varying channel causes a deep fade which incurs a heavy power penalty on modulation performance over wireless channels. Again if the transmitted signal bandwidth is greater than the coherence bandwidth of the channel that also causes frequency selective fading which again creates multiple
versions of the transmitted waveform which are attenuated or may be delayed in time domain which indirectly produces a distorted version of the original signal. Overall fading changes overall characteristics of the transmitted signal by changing its amplitude, phase and frequency. There are several techniques to reduce this kind of challenges. Equalization, diversity, channel coding are three different techniques which can be used to improve the signal quality and overall quality of service of the communication link. Equalization is a process which is used to make the wireless channel ISI free. Equalizer is actually an inverse filter of the channel. It reduces the channel vulnerabilities by altering the channel characteristics. For example, if a channel is frequency selective which can create multiple versions of the transmitted signal by attenuating the signal and delaying in time domain the equalizer can make the channel flat and composite by enhancing frequency components. Again channel coding is a technique where we can add some redundant data with the transmitted data such that if any case some fading occurs we can still recover the original transmitted signal. One of the best way among all these three is reducing fading effects is a variety of combinations of signal paths that are independently fading known as diversity which is an another technique to improve the quality of the communication system without altering the channel. Diversity association is facilitated because autonomous signal tracks are less likely to undergo a concurrent deep fade. Therefore, after diversification, it is planned to send the same data along independent vanishing paths. These independent pathways are connected in such a way that the disappearance of the resulting signal is reduced. Here are two types of fading small-scale and large-scale fading. Profound and rapid amplitude fluctuations characterize small-scale fades as the mobile transits over ways of just fewer wavelengths. Multiple reflections from the mobile environment cause these fades. Small-scale fading typically results in a Rayleigh fading distribution of signal strength over small distances. The Large-scale fading is mainly due to shading due to variation in both the terrain profile and the nature of the surroundings. In the case of deep shadows, the signal strength received on the mobile can drop significantly below the free space [10-12].
-
THEORETICAL OVERVIEW
We noticed that some fading incurs a heavy power penalty on modulation performance over wireless channels. One of the best ways for reducing fading [1, 16] effects is a variety of
combinations of signal paths that are independently fading. Diversity association is facilitated because autonomous signal tracks are less likely to undergo a concurrent deep fade. Therefore, after diversification, it is planned to send the same data along independent vanishing paths. These independent pathways are connected in such a way that the disappearance of the resulting signal is reduced. Here are two types of fading
small-scale and large-scale fading. Profound and rapid amplitude fluctuations characterize small-scale fades as the mobile transits over ways of just fewer wavelengths. Multiple reflections from the mobile environment cause these fades. Small-scale fading typically results in a Rayleigh fading distribution of signal strength over small distances. The Large- scale fading is mainly due to shading due to variation in both the terrain profile and the nature of the surroundings. In the case of deep shadows, the signal strength received on the mobile can drop significantly below the free space.
-
INTRODUCTION TO DIVERSITY
In wireless systems, there are numerous methods for achieving independent feeding channels. One option is to use self- governing fading systems with receiver space fluctuation without affecting signal strength or bandwidth. In other words, the observable sequence of diversity signals improves the signal-to-noise power ratio at the receiver compared to the SNR received with only one receiving antenna[2]. Weighting the antenna broadcast power relative to the channel gain with the transmitter space fluctuation yields the SNR picks, also known as array gain. The Diversity gain is the change in the slope of the error probability due to the combination of the variances. The highest diversity gain for transmitter or receiver space diversity usually necessitates that the separation between antennas be such that the vanishing amplitudes for each antenna are nearly indepndent, based on the space diversity. Getting two broadcast antennas or two reception antennas with different polarizations is another technique to get variety. Polarization variety is the term for this. There are two drawbacks to polarization variety. First, we can have two types of polarization corresponding utmost pair diversity branches (vertically and horizontally).The second is that when transmitting or receiving power is divided between two differentially polarized antennas; polarization diversity effectively dissipates the moiety of the power (3 dB).By attaining frequency diversity, where the channel's coherence departs the bandwidth carrier[4], we can transmit the same narrowband signal at different carrier frequencies. Multiple frequency bands deliver the signal overhead, which needs considerable communication power. When time variation is more important than channel coherence time, time diversity is done by forwarding the same signal at different times. Increased temporal diversity does not necessitate more power, but it does limit data rate because data is reproduced in the variation time slot rather than new data being sent to that time slot. We focus on the space variety, while the principles can be extended to any variety. Because their system models and assembling processes are fundamentally different, receiver diversity exists.
The independent fading paths associated with numerous receive antennas are merged in receiver diversity to produce a signal, which is then passed via a typical demodulator.
Combining can be done in various ways, each with its level of complexity and overall performance. As demonstrated in Figure 1 for N-branch diversity, the output of most combining procedures is simply a weighted total of the various fading routes or branches. When all of the complex except one are zero, just one path is sent to the combiner output. If more than one of the is nonzero, the combiner will combine numerous pathways, each of which will be weighted differently. When more than one branch signal is combined, co-phasing is required, in which the phase of the ith branch is removed by
multiplying by = for some real-valued .This phase removal necessitates the observation of each branch coherently to ascertain its phase . The branching signals would not add up coherently in the connector without co- phasing, leading to significant fading due to the constructive and destructive addition of the signals in all the branches. With no change in performance, multiplication by can be done before detection (pre-detection) or after detection (post- detection)[3].
After detection, combining is usually done because the branch signal power and phase are necessary to obtain the proper value. Multiple branch post-detection combining necessitates a dedicated receiver for each branch to determine the branch phase, which adds hardware complexity and power consumption, especially when there are many branches. The primary goal of diversity is to integrate the independent fading paths to mitigate fading impacts. The original transmitted signal multiplied by a random complex amplitude term
equals the signal output from the combiner. The distribution is a function of the number of diversity paths, the
fading distribution on each path, and the combining
mechanism, resulting in a random SNR at the combiner output. The diversity order of this statement is which corresponds to a single receive antenna. A system with N antennas can have a complete diversity order of N, and when the diversity order equals N, the system is said to have achieved complete diversity order. In the next quarter, we resolve to go via the various combining approaches and how they work in more depth. These systems are capable of a variety of efficiency and complexity trade-off[5-7].
-
Selection Diversity:
Figure 1: Block diagram for selection diversity
The signal on the branch with the highest SNR is outputted by the combiner in selection combining (SC). If all branches have the same noise power = N, this equals picking the branch with the greatest . Since only one branch is used simultaneously, SC frequently only requires one receiver to be switched into the active antenna branch. For systems that broadcast continuously, however, a specialized receiver on each antenna branch may be required to monitor SNR on all branches simultaneously. The combiner's path output has an SNR equal to the maximum SNR of all the branches when using SC.
Furthermore, because only one branch output is needed, multiple branch co-phasing is unnecessary; hence this approach may be employed with either coherent or differential modulation. The best signal from all the signals from the diversity channels at the receiver is selected using the selection combining procedure. The receiver uses Switch logic to monitor the SNR of the incoming signals in this approach. This procedure chooses the strongest signal from all of the channels' signals. The predicted Diversity gain is represented as a power ratio when N signals are independent, and Rayleigh distributed. As a result, any extra advantage quickly reduces as the number of channels grows.
The gain of each branch is modified from all Diversity branches to obtain the average SNR of each branch; no comparison is necessary. That means average value of signal to noise ratio[9].
Equation 1
For Rayleigh fading channels, a has a Rayleigh distribution, hence and X have a two-degree-of-freedom chi-square allocation.
That if every arm has an immediate SNR =, then the pdf of is calculated from
Equation 2
A represents the average SNR of each branch. The chance of a single branch with SNR just under a
threshold is
Equation 3
Now, the possibility of all M independent diversity branches receiving signals that are less than some given SNR threshold is
Equation 4
The possibility of all nodes failing to reach SNR = is given by in Equation 5. If one branch obtains SNR , the odds that one or more branches achieve SNR > is given by
Equation 5
When selection diversity is utilized, Equation 6 provides an expression for the likelihood of surpassing a threshold. When using diversity, it is required to first find the pdf of the fading signal[14] in order to estimate the average SNR of the received signal. To get the average SNR for selection diversity, first compute the derivative of . Continuing in this direction of some way of finding PDF
Equation 6
Equation 7 is evaluated to yield the average SNE improvement offered by selection diversity is
Equation 7
The mean SNR in the line picked via selecting variance naturally grows, as it is always assured to be over the set threshold, as shown by Equation 8. As a result, selection diversity improves the connection margin on average without requiring more transmitted and processed digitally or specialized receiver electronics. The average bit error rate for various modulations can be directly connected to the diversity improvement. However, because it does not employ all of the
viable branches at the same time, it is not an ideal diversity strategy. Maximal ratio combining employs each of the M branches in a co-phased and weighted manner to ensure that the receiver always has the greatest possible SNR[11,13].
-
Maximal Ratio Combining:
The data from all M sections are weighted according to their respective signal voltage to noise power ratios and then totaled. A block diagram of the technique is shown in Figure
2. Individual signals must be co-phased before being added together (unlike selection diversity), which usually necessitates a separate receiver and phasing circuit for each antenna element. As mentioned in calculation, maximal ratio combining gives an output SNR equal to the total of the individual SNRs. As a result, it has the benefit of delivering an acceptable SNR output even when none of the component signals are acceptable. This approach provides the best statistical reduction of fading of any known linear diversity combiner[8]. This ideal variety is now possible because of modern DSP algorithms and digital receivers.
The voltage signals from each M diversity branch are co- phased to give coherent voltage addition and separately weighted to offer the best SNR in maximal ratio combining. If each branch has gained, the signal envelope applied to the detector will be as follows:
Equation 8
The total noise power supplied to the sensor is just the weighted sum of the noise in each branch, given that each of the branch has same mean noise power N. Thus,
Equation 9
As a result, an SNR is applied to the detector, with equal to,
Equation 10
The probability that is less than some SNR threshold is,
Equation 11
The probability distribution for maximal ratio combining is given by Equation 12. The average SNR is just the sum of the individual from each branch, according to Equation 11. To put it another way,
Equation 12
The gain and phase control techniques used in maximum ratio combining receivers are similar to those used in equalizers and RAKE receivers. Maximal ratio combining structures are shown in Figures 2 . Calculate of maximal ratio combining, which may be used to nearly every diversity application, but at a significantly higher cost and complexity than other diversity strategies.
Figure 2: Maximal Ratio Combiner
MRC is the popular term for this, and usually, the diagram x is sent through a channel with a coefficient of h, and received through one antenna with a particular weight factor. A particular weight factor weights the second antenna, up to , weight , after which the receiver does some processing, and the output y is obtained. It might also be written as M for simplicity, is the noise caused by that branch. As a result, anything it receives gets doubled by this weight factor[11].
-
Equal Gain Combining:
We have the variable weighting capability necessary for accurate maximum ratio combining in some circumstances. The branch weights are all set to one, but the signals from each branch are co-phased to offer equal gain combining variety in such instances. This permits the receiver to take advantage of signals received on each branch simultaneously. The ability to make a good signal out of many bad ones is still there, and performance is only slightly worse than maximal ratio combining and somewhat better than selection diversity. It shows that EGC's efficiency is similar to that of MRC, with usually less than one dB of power loss. This is the cost of increasing the dimensionality of employing equal gains. It has a more comprehensive analysis of SC, MRC, and EGC performance[15].
-
-
SIMULATION RESULT
Figure 2: BER of BPSK for different Diversity Branches
Figure 3: Bit error rate for MRC and EGC
-
CONCLUSION
In this paper we have analyzed how performance of any wireless communication system can be enhanced with various techniques. Basically, we have experimented on diversity technique with which we can provide wireless link improvement at a very low cost compared to another techniques. Here we have presented a comparison on three basic diversity techniques selection diversity, maximal ratio combining, equal gain combining. From the simulation results included on this paper,it can be seen that selection diversity provides better improvement in the link margin without wasting of much power and also its circuit is quite easy to design as in each time the receiver will select only one branch with highest signal to noise ratio. However this technique of selecting one branch at a time is not an optimal technique to enhance the channel impairments as the resultant signal to noise ratio is not the contribution of all the diversity branches therefore we have shown how maximal ratio combining
techniques provides an enhancement over selection diversity by including all the branches and also it can provide an acceptable signal to noise ratio even if all the branches have a low signal to noise ratio as the resultant signal to noise ratio will be sum of all the signal to noise ratio for each branch. Again, we have also shown how maximal ratio combining technique is still superior to equal gain combing technique for the signal to noise value in terms of performance.
-
REFERENCE
-
-
M. K. Simon and M.-S. Alouini, Digital Communication over Fading Channels: A Unified Ap proach to Performance Analysis, Wiley, New York, 2000.
-
W. C. Y. Lee, Mobile Communications Engineering, McGraw-Hill, New York, 1982.
-
M. Blanco and K. Zdunek, Performance and optimization of switched diversity systems for the detection of signals with Rayleigh fading, IEEE Trans. Commun., pp. 188795, December 1979.
-
M. Yacoub, Principles of Mobile Radio Engineering, CRC Press, Boca Raton, FL, 1993.
-
S. Alamouti, A simple transmit diversity technique for wireless communications, IEEE J. Sel. Areas Commun., pp. 14518, October 1998.
-
A. Paulraj, R. Nabar, and D. Gore, Introduction to Space-Time Wireless Communications, Cambridge University Press, 2003.
-
E. G. Larsson and P. Stoica, Space-Time Block Coding for Wireless Communications, Cambridge University Press, 2003.
-
M. K. Simon and M.-S. Alouini, A unified approach to the performance analysis of digital communications over generalized fading channels,
Proc. IEEE, pp. 186077, September 199
-
Nasser, Y. Effect of mobility on the performance of Amplify-and-Forward cooperation in SC-FDMA systems Computing, Networking and Communications (ICNC), 2012 International Conference on, Pages. 798 803, Jan. 30 2012-Feb. 2 2012
-
M. K. Simon and M.-S. Alouini, A unified approach for the probability of error for noncoherent and differentially coherent modulations over generalized fading channels, IEEE Trans. Com mun., pp. 162538,
December 199
-
D. B. Da Costa, M. D. Yacoub, and G. Fraidenraich, Second-order statistics of equal-gain and maximal-ratio combining for the – (gener- alized gamma) fading distribution, in Spread Spectrum Techniques and Applications, 2006 IEEE Ninth International Symposium on. IEEE, 2006, pp. 342346.
-
Islam, MD. Jaherul, Performance Analysis of Diversity Techniques for wireless communication systems, 2012.
-
Stefan Person,''Compendium in the course 'Wireless Communication', University of Kalmar, Sweden, 2007''
-
Dr. Kamilo Feher, Wireless Digital Communication-Modulation and Spread spectrum Application. Prentice Hall of India, New Delhi, 2001.
-
A. Goldsmith, Wireless communication, 1st ed. Cambridge University Press 40West 20th Street, NewYork, NY 10011-4211, USA: Cambridge University Press, 2005. [Online]. Available: www.cambridge.org/9780521837163.
-
M. D. Yacoub, C. R. C. M. da Silva, and J. E. V. Bautista, Second- order statistics for diversity-combining techniques in Nakagami-fading channels, IEEE Trans. Veh. Technol., vol. 50, no. 6, pp. 14641470, Nov. 2001.