
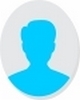
- Open Access
- Authors : Arya Mukesh , Narayanan N I
- Paper ID : IJERTV11IS070143
- Volume & Issue : Volume 11, Issue 07 (July 2022)
- Published (First Online): 27-07-2022
- ISSN (Online) : 2278-0181
- Publisher Name : IJERT
- License:
This work is licensed under a Creative Commons Attribution 4.0 International License
Numerical Study on The Flexural Behaviour of Steel-Concrete-Steel Sandwich Beam Enhanced with Stiffener
Arya Mukesh
Dept. of Civil Engineering Govt. College of Engineering Kannur, India
Prof. Narayanan N I
Dept. of Civil Engineering Govt. College of Engineering Kannur, India
Abstract Steel-Concrete-Steel sandwich beam with stiffener in the bottom face plate, in addition to C shaped shear connectors connecting the top and bottom faceplates, to improve the flexural behaviour of the sandwich beam is studied in this paper. Sandwich beam with Ultra High-Performance Concrete as core, and mild steel face plates and stiffener are considered in this study. The effect of thickness of face plates, spacing of C shaped connectors, thickness and height of stiffener plate are the parameters considered in the study. The effect of these parameters on ultimate strength and load-deflection behaviour of the sandwich panel are studied numerically using finite element software ABAQUS/CAE. Introduction of stiffener plate of thickness 3 mm and height 50 mm to the base face plate improved the ultimate capacity by 28.58 %, compared to beam of same geometric parameters without stiffener plate. Increasing the thickness of faceplates improved the ultimate load of the beams. 54.56% increase in the load capacity was observed as the thickness increased from 3 mm to 6 mm. Increasing the stiffener thickness showed limited influence on the ultimate load, 11.5 % increase in the Ultimate load was observed as the thickness was increased from 3 mm to 6 mm. The elastic part of the Load- Deflection curve showed nearly same variation to increase in the thickness of the stiffener plate. The effect of the spacing of the channel section on the ultimate load was limited. Percentage decrease of 9.76 was observed as the spacing increased from 90 mm to 210 mm. The percentage increase in the load capacity was 4.09 % as stiffener height was increased from 25 mm to 50 mm. All the beams analyzed experienced vertical cracking which was described in terms of strain in concrete. Local buckling of top face plates was also observed in case of beams with channel section spacing of 150 mm and 210 mm.
Keywords Steel-concrete-steel, sandwich beam, C shaped shear connectors, Ultra High- Performance Concrete, UHPC, Stiffener
-
INTRODUCTION
Steel-Concrete-Steel Sandwich Beam consists of two steel face plates and a concrete core in between the face plates. The face plates are relatively thin compared to concrete core. The key aspect in the SCS sandwich structure is to ensure the composite action of steel plates and concrete so that it act as a single structural unit. To ensure the composite behaviour of the sandwich beam, shear connectors which connects the face plates and the concrete core or certain adhesive materials at the interface of steel face plates and concrete core are used. The study by using enhanced Channel connectors, conducted by Yan et. al. showed high tensile resistance to separation of faceplates from concrete core and highly efficient bond at the interface of faceplate and core concrete. This study focuses on SCS sandwich beam with channel section shear connectors. Jia-Bao Yan et al. (2020) [1] [2] [3] conducted experimental
and numerical studies on the performance of the SCS sandwich beams with channel section shear connectors for connecting the face plates at the top and bottom. The influence of steel plate thickness and grade, shear-span ratio, channel spacing, orientation of the channel connectors were the parameters studied in their study. The authors developed a reliable analytical model to predict the stiffness and strength of these composite beams. Jia-Bao Yan et al. (2020) [4] conducted experimental studies to investigate the shear behaviour of the sandwich structures with C shaped shear connectors considering the influence and effect of the dimensions and orientation of the connectors and the strength of the core from the push out tests. Tao Wang et al. (2020) [5] provided the details of the structural performance of structural components. Bending, compression, in-plane and out-of-plane punching shear test, and pushout tests were carried out to study the flexural, compression, cyclic, and shear behaviour of these structural components. Analytical equations to predict these behaviours were also formulated by the authors. Jia-Bao Yan et. al. (2020) [6] investigated the efficiency of headed shear stud connectors in Steel-UHPFRC-Steel beam. The impact of different compressive strength of UHPFRC, thickness of face plate, distance at which studs are placed along the length of the beam, and loading position were considered in the study. Yonghui Wang et. al. (2021) [7] [8] investigated the behaviour SCS sandwich beams and panels with longitudinally aligned stiffener plates along with bolted shear connectors. It was observed in all the tested beams that failure was in flexural mode. It was inferred from the impact test on the beams that the addition of stiffeners on the bottom plate improved the impact resistance of the sandwich beam.
-
NUMERICAL MODELLING
The finite element modelling of the SCS sandwich beam was carried out in the finite element software ABAQUS CAE. ABAQUS/ Explicit solver was used for Finite element analysis. The flexural behaviour of the beam was established by simulating four- point bending test in the software. The finite element beam models developed in this study were validated against the models in the experimental study conducted by Jia Bao Yan et. al. (2020) [2].
-
Description of the Experiment
In the experimental study conducted by Jia-Bao Yan et al. (2020) [1] [2], SCS sandwich beams with UHPC core and channel shaped shear connectors were introduced. The fabrication of the beams for the study was done in a sequence of steps. First, Channel connectors and nuts were welded to the top and bottom face plates at the required location. Then
the two face plates with welded connectors were assembled with bolts. After the steel skeletal framework of the beam was completed, concrete was poured unto the framework. Alternate welding and bolting on the top and bottom face plates was done to connect the channels to the plates. Flexural behaviour of the beam was obtained by four-point bending test. The load applied to the beam was displacement- controlled manner through the load cell and spreading beam.
-
Geometrical Modelling
The dimensions of the beam and material properties of the components of the beam adopted in the experimental study conducted by Jia Bao Yan et. al. (2020) [2] are used for the validation of the FE model. The beam model of span 1900 mm and width 200 mm; the channel section with flange thickness 9 mm, web thickness 5.5 mm, height of the channel section h mm, width 50 mm and length 53 mm; bolt of shank diameter 14 mm for connection, and mild steel plates of thickness 2.7 mm and 4.7 mm are dimensions of the components of the model used for the validation purpose. The steel skeletal framework of the model is given in Fig.1.The dimensional details of the beam used for the validation of the FE model are given in Table 1.
Fig. 1 Dimensions of SCS validation beam model in XY plane
The different components of the model are separately modelled in the part module. The channel section with top bolt hole, channel section with bottom bolt hole, bolt, concrete core with C shaped holes to accommodate the channel connector and holes, top and bottom steel plates, loading platen and supports are the components of the SCS beam. The concrete core was modelled with channel shaped holes. The concrete core moel was thoroughly partitioned so that the part can be given structured mesh. The assembled SCS beam model is shown in Fig.2.
Model Name
a (mm)
Thickness of UHPC (tc) (mm)
Thickness of steel plate (tp) (mm)
Spacing of Channel connectors
(S) (mm)
Shear Span (L1)
(mm)
B1
30
119.3
2.7
90
600
B4
30
119.2
4.7
90
600
TABLE I. DETAILS OF VALIDATION MODELS [2]
Fig. 2 SCS Sandwich beam modelled in ABAQUS
-
Material Modelling
The constitutive model of the steel components was developed by Jia-Bao Yan et. al. (2020) [1][2] based on the tensile coupon test conducted by them. The constitutive material model for the UHPC given in the work done by J B Yan et al. was taken for the modelling. The authors Jia Bao Yan et. al. (2020) adopted the stress-strain constitutive law of UHPC in compression and tension from Yang et. al. [9] and Zhang et al. [10] respectively. These material models were adopted for the parametric studies as well. For steel, elastic plastic, non-linear kinematic hardening model was adopted in the study. The stress- strain characteristics of the steel material were input using the trilinear stress strain curve which includes the strain hardening of the steel material. The modulus of elasticity Es = 202 GPa and Poissons ratio was taken as 0.3. The material model of UHPC adopts Concrete Damage Plasticity Model (CDPM) which can conveniently implement nonlinear behaviour of the material. In CDP model, density of concrete, compression behaviour, tension behaviour, plasticity parameters, elastic properties including Youngs Modulus and Poissons ratio. The concrete in the study had ultimate tensile stress of 6.65 MPa and ultimate compressive stress of 125.4 MPa.
-
Meshing
To select a suitable mesh for the study, FE model with 4 different mesh sizes were analysed. Beam was meshed with size of 5 mm, 10 mm, 15 mm, and 20 mm and load deflection curve was plotted for each beam. B1 model (J B Yan et. al.) were taken for the mesh study. The results from the FE analysis were compared to the test results to select the most accurate mesh size for the study. Fig.3 shows the comparison of load deflection behaviour of the beams analysed. From the figure, change in mesh size had limited influence on the FE Load displacement results. However, the initial elastic behaviour of the beam was accurately traced by the beam meshed with 10 mm size. The ultimate load at the 44 mm displacement was compared in the beams analysed. Beam meshed with 5 mm size showed ultimate load result closer to the test result compared to other beams. Table II shows the comparison of Ultimate load of beams with different mesh size and test beam B1. Considering the accuracy at the initial part of the load deflection curve, limited difference in the ultimate load, and computational time required, mesh size of 10 mm was adopted for the study.
Fig. 3 Load deflection curve of B1 with different mesh sizes
TABLE II. COMPARISON OF ULTIMATE LOAD OF BEAM B1 FOR DIFFERENT MESH SIZES
Global Mesh size (mm)
Ultimate load (FE) (kN)
Ultimate load (Test-B1) (J B
Yan et al.) (kN)
Ultimate Load (Test/FE)
5
92.4095
88.4
0.96
10
93.585
88.4
0.94
15
96.445
88.4
0.92
20
97.533
88.4
0.90
-
Loading, Boundary conditions and Interactions
Boundary condition at the support were provided such that all the movements in the x, y and z direction were arrested. The load is applied as displacement loading in the negative y direction, which is applied along the loading platen. Displacement loading of 50 mm was applied to the loading platen.
The interactions of all the contact pairs were taken as surface to surface contact. The hard contact property along the direction and penalty friction property perpendicular to the direction were used to define the interaction. In this study, friction coefficient of 0.5 were adopted.
Tie constraints were provided to channel faceplate interface at the welded portions of the beam.
-
Validations
For validation of the SCS sandwich beam, Model B1, and Model B4 (Jia-Bao Yan et.al. 2020) [2] were modelled and analysed using ABAQUS software.
Fig.4 shows the comparison of Load Displacement curve from FE analysis and experimental study for B1 model. FE generated Load- Displacement curve showed reasonable conformation to the experimental load-displacement curve. The percentage difference between the Ultimate load results of FE analysis and the test result is 5.86 %. Initial elastic part of the graph showed good conformation to the test curve.
Fig. 4 Load deflection curve of B1
The logarithmic strain contour in ABAQUS was used to describe the cracks in the UHPC core. Fig.5 and Fig.6 shows the comparison of logarithmic strain contour and cracks in the concrete as in the experimental test by Jia-Bao Yan et al. and NFEM developed in this study, respectively. The figures show that developed NFEM simulates reasonably well, the deformations and cracks in the UHPC core, even though crack pattern cannot be predicted with full accuracy. The developed NFEM could capture most of the main cracks in the UHPC.
Fig. 5 Cracks in concrete after experimental test and FEA of B1 (Jia-Bao Yan et al. (2020))
Fig.6 Strain contour of B1 model
Fig.7 shows the comparison of Load Displacement curve from FE analysis and experimental study for B4 model. FE generated Load- Displacement curve showed reasonable conformation to the experimental load-displacement curve. The percentage difference between the Ultimate load results of FE analysis and the test result at displacement of 45 mm is
0.57 %. But the load displacement curve in the strength hardening part of the curve showed a slight variation. In the initial elastic stage, FE graph followed the test curve closely.
Fig.7 Load deflection curve of B4
The comparison of logarithmic strain contour and cracks in the concrete given in Fig.8 and Fig.9 show that developed NFEM simulates the deformations, and cracks in the UHPC core, reasonably well even though crack pattern cannot be predicted with full accuracy. The developed model could predict most of the crack pattern in the UHPC. The regions of crack formation can be easily identified from the strain contour.
Fig.8 Cracks in concrete after experimental test and FEA for B4 (Jia-Bao Yan et al. (2020))
-
-
PARAMETRIC STUDY
The validated finite element model is used to carry out study on the Steel-Concrete-Steel sandwich beam with the addition of stiffener plate on the bottom steel plate, considering different parameters. The study is conducted to predict the load- displacement behaviour and failure characteristics of SCS sandwich beams with stiffener plate.
Beam of span 1600 mm and depth 120 mm was analyzed in the study. The beam was loaded at a distance 500 mm from the supports. The supports were placed at 50 mm from the free end. The channel section of height 120 mm, thickness of flange 9 mm, thickness of web 5.5 mm, length of flange 53 mm, and width of flange 50 mm is taken as shear connector for the beam. The arrangement of Channels and stiffener plate is shown in Fig.10.
Fig.10 Arrangement of Channels and stiffener plate in parametric model
The parameters considered in this study are, thickness of the stiffener plate, height of the stiffener plate, thickness ofthe face plate, and Spacing of the channel section connectors.
The details of the parameters considered in the study are listed in Table III.
Fig.9 Strain contour of B4 model
TABLE III. DETAILS OF MODEL FOR PARAMETRIC STUDY
Model Name
Thickness of steel plate (mm)-(F)
Thickness of stiffener plate (mm)-(S)
Spacing of Channel connectors (mm)-(P)
Height of the stiffener (mm)- (H)
S1-P1-F1
3
3
90
50
S1-P1-F2
4.5
3
90
50
S1-P1-F3
6
3
90
50
S2-P1-F1
3
4.5
90
50
S3-P1-F1
3
6
90
50
S1-P2-F1
3
3
150
50
S1-P3-F1
3
3
210
50
S0-P1-F1
3
–
90
50
S1-P1-F1-H1
3
3
90
25
S1-P1-F1-H3
3
3
90
75
-
Comparison of SCS sandwich beam with stiffener and without stiffener
Fig.11 shows load-displacement behaviour of beam without and with stiffener plate welded to bottom face plate in the beam of same dimensional parameters. The load capacity at 50 mm displacement was 129.77 kN and 166.86 kN respectively for beam without stiffener and with stiffener of dimension 3 mm. Addition of stiffener plate increased the load carrying capacity of the beam by 28.58%.
Fig.11 Load- Displacement curve of beams with and without stiffener
The initial elastic part of the curve shows same behaviour for the two cases. The two beams exhibit different behaviours in the nonlinear part of the curve. The nonlinear behaviour occurred in the beam after the yielding of the bottom face plate. Here, in beam S1P1F1, stiffener plate was present in the bottom faceplate, so the stiffener plate along with bottom face plate underwent yielding together which enhanced the load at which yielding occurred, the stiffness and the ultimate load. The Channel section connectors also showed yielding and the stress distribution in the channel section was also enhanced with the addition of stiffener. The increase in the load carrying capacity can be attributed to the yielding of the stiffener plate at the bottom side of the beam.
Fig.12 and Fig.13 shows stress contour of beam with stiffener and without stiffener respectively. The stiffener plate in the bottom steel plate is yielded which implies that the addition of stiffener plate in the bottom face plate contributed to the enhancement of the flexural resistance of the beam. Presence of stiffener plate in the bottom plate also caused the distribution of stresses to the channel section which increased the load capacity of the beam with stiffener.
Fig.12 Stress contour beam with stiffener- S1-P1-F1
Fig.13 Stress contour beam with stiffener- S0-P1-F1
The strain contour for S0-P1-F1 and strain contour of S1-P1-F1 are shown in Fig.14 and Fig.15 respectively. It can be said that the presence of stiffener plate enhances the overall structural performance of the beam.
Fig.14 Strain contour beam with stiffener- S0-P1-F1
Fig.15 Strain contour beam with stiffener- S1-P1-F1
-
Effect of steel plate thickness
Fig.16 shows Load-Displacement behaviour of beams with varying thickness of the top and bottom face plate. The load capacity for steel plate of thickness 3 mm, 4.5 mm and 6 mm are 159.24 kN, 197.78 kN, and 246.13 kN respectively. As the thickness of the stiffener plate was increased, increase in the load carrying capacity can be observed. As the plate thickness was increased from 3 mm to 4.5 mm, load carrying capacity increased by 24.2 % and increase in plate thickness from 4.5 mm to 6 mm showed 24.44% load carrying capacity. The stiffness of the load- displacement curve is seen to be increased as the plate thickness increased from 3 mm to 6 mm. The reason for
this increase was that with thickness increase, Moment of inertia of the section and percentage of steel in cross section of beam increase, which in turn improved the flexural capacity of the beam. In three beams, vertical cracks were observed, which indicate flexural mode of failure.
Fig.16 Load- Displacement curve of beams with varying steel plate
thickness
-
Effect of thickness of stiffener
Fig.17 shows Load-Displacement behaviour of beams with varying thickness of the stiffener plate. The figure shows that the initial elastic stage of the beam is nearly unaffected by the change in the thickness of the stiffener plate for 3 mm and 4.5 mm stiffener thickness, but for beam with stiffener of thickness 6 mm, initial elastic part of the curve was slightly stiffer than the other two beams. At the plastic region, increase in the load capacity was found with increase in the thickness value. The load capacity at 50 mm displacement for beams with stiffener thickness of 3 mm, 4.5 mm, and 6 mm are 159.24 kN, 175.68 kN, and 177.56 kN respectively. The increase in the percentage load was
11.5 % as thickness increased from 3 mm to 6 mm. The percentage difference between load at 4.5 mm and 6 mm was only 1.07%. Non-linear range begins after yielding of bottom plate. Change in the behaviour in nonlinear part and increase of the load can be attributed to the variation stiffener thickness which increased the steel in cross section to resist tension.
Fig.17 Load- Displacement curve of beams with varying stiffener
thickness
-
Effect of spacing of channel sections
Fig.18 shows Load-Displacement behaviour of beams with varying spacing of channel section shear connectors. The load capacity as spacing was increased from 90 mm to 150 mm to 210 mm are 159.24 kN,
151.06 kN, and 143.69 kN respectively Stiffness of beam decreased as the spacing of the Channel section shear connectors increased as it can be seen from the graph and the variation of ultimate load with increase in spacing is less. 9.76 % decrease in ultimate load occurred as spacing increased from 90 mm to 210 mm.
Fig.18 Load- Displacement curve of beams with varying spacing of channel section
Fig.19 Stress contour of beam S1P2F1
For beams with spacing 150 mm and 210 mm, the top face plate showed local buckling. The local buckling in the top plate reduced the ultimate strength, but the composite action of UHPC, steel plates and channel connectors along with stiffener contributed to the ductility of the beam.
-
Effect of height of stiffener plate
Fig.20 shows Load-Displacement behaviour of beams with varying height of stiffener plate. The load capacity, as stiffener height increased from 25 mm to 50 mm, and 75 mm are 152.98 kN, 159.24 kN, and 163.41 kN respectively. Initial elastic phase of the curve was same till the strain hardening part of the curve is reached. The percentage increase in the load capacity was 6.82 %. The increase in the stress distribution to the channel connectors due to increase in the height of stiffeners improved the load carrying capacity.
Fig.20 Load- Displacement curve of beams with varying height of
stiffener plate
-
-
CONCLUSIONS
The parameters considered are thickness of the face plate, thickness of the stiffener plate, Spacing of the Channel connectors and height of the stiffener plate. From the parametric study conducted on the SCS sandwich beam with stiffener plate in the bottom tension face plate, following conclusions are obtained:
-
All the beams analyzed showed vertical crack formation, which implied that the mode of failure is flexural mode. In case of beams with channel section connectors at spacing 150 mm and 210 mm, local buckling of top flange was observed.
-
The ultimate capacity was found to be increased by 28.58
% when a stiffener plate of thickness 3 mm and height 50 mm is introduced, compared to beam of same geometric parameters without stiffener plate.
-
Yielding of the stiffener plate can be seen from the FE results obtained and the strain contour which was used to describe cracks in concrete showed more crack locations on the beam without stiffener plate compared to beam with stiffener plate.
-
Increasing the thickness of faceplates improved the ultimate load of the beams. 54.56% increase in the load capacity was observed as the thickness increased from 3 mm to 6 mm.
-
Composite action of face plate and UHPC, and mechanical properties of steel and UHPC influence elastic stiffness. When the thickness of the face plate is increased, it increases the cross-sectional steel content and decreases the slenderness ratio, which increase the ultimate strength.
-
Increasing the stiffener thickness also showed limited influence on the ultimate load, 11.5 % increase in the Ultimate load was observed as the thickness was increased from 3 mm to 6 mm.
-
The elastic part of the Load- Deflection curve showed nearly same variation to increase in the thickness of the stiffener plate.
-
The spacing of the Channel section shear connectors has limited effect on the Load-deflection behaviour of the
beams. However, increase in the spacing to 150 mm and 210 mm showed local buckling of the top face plate. The effect of the spacing of the channel section on the ultimate load was less and the percentage decrease of 9.76 was observed as the spacing decreased from 90 mm to 210 mm.
-
The height of the stiffener plate also had only limited influence on the load carrying capacity. Elastic behaviour was same for three heights of stiffener analyzed. The percentage increase in the load capacity was 6.82 % as stiffener height was increased from 25 mm to 75 mm. The increase in the stress distribution to the channel connectors due to increase in the height of stiffeners improved the load carrying capacity.
REFERENCES
[1] Jia-Bao Yan, Huining Guan, and Tao Wang (2020), Steel- UHPC-steel sandwich composite beams with novel enhanced C- channel connectors: Tests and analysis, Journal of Constructional Steel Research, 170, 106077. [2] Jia-Bao Yan, Hui-Ning Guan, and Tao Wang (2020), Numerical studies on steel-UHPC-steel sandwich beams with novel enhanced C-channels, Journal of Constructional Steel Research, 170, 106070. [3] Jia-Bao Yan, Hui-Ning Guan, and Tao Wang (2020), Finite element analysis for flexural behaviours of SCS sandwich beams with novel enhanced C-channel connectors, Journal of Building Engineering, 31, 101439. [4] Jia-Bao Yan, Huitao Hu, and Tao Wang (2020), Shear behaviour of novel enhanced C-channel connectors in steel- concrete-steel sandwich composite structures, Journal of Constructional Steel Research, 166, 105903. [5] Tao Wang and Jia-Bao Yan (2020), Developments of steel- concrete-steel sandwich composite structures with novel EC connectors: Members, Journal of Constructional Steel Research, 175, 106335. [6] Jia-Bao Yan, Anzhen Chen, Huining Guan, and Tao Wang (2020), Experimental and numerical studies on ultimate strength behaviour of SCS sandwich beams with UHPFRC, Construction and Building Materials, 256, 119464. [7] Yonghui Wang, Jingyi Lu, Suntao Liu, Ximei Zhai, Xudong Zhi, and Jia-Bao Yan, Behaviour of a novel stiffener-enhanced steelconcretesteel sandwich beam subjected to impact loading, Thin-Walled Structures, 165, 107989. [8] Yonghui Wang, Tilak Prasad Sah, Suntao Liu, and Ximei Zhai (2022), Experimental and numerical studies on novel stiffener- enhanced steel-concrete-steel sandwich panels subjected to impact loading, Journal of Building Engineering, 45, 103479. [9] J. Yang (2007), Flexural Behavior of Ultra-High Performance Concrete Beams Prestressed with CFRP Tendons, Hunan University, Changsha, China,(in Chinese). [10] Z. Zhang, X. Shao, W. Li, P. Zhu, H. Chen (2015), Axial tensile behavior test of ultra high performance concrete, China J. Highw. Transp. 28 (8) 5058. [11] Aditya P. Sawant and Pramod B. Salgar (2021), A Review on Experimental Performance of Steel-Concrete-Steel (S-C-S) Composite Beam with Varying Stud Connectors, International Research Journal of Engineering and Technology (IRJET), Volume: 08 Issue: 06, 2395-0072.