
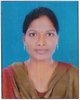
- Open Access
- Authors : M. Vijaya Saritha , S Dastagiri , M. V. Lakshmaiah , V. Ramesh Kumar, K. E. Supriya, G. Pakardin
- Paper ID : IJERTV11IS110069
- Volume & Issue : Volume 11, Issue 11 (November 2022)
- Published (First Online): 17-11-2022
- ISSN (Online) : 2278-0181
- Publisher Name : IJERT
- License:
This work is licensed under a Creative Commons Attribution 4.0 International License
Structural and Optical Properties of ZnTiO3 Nanoparticles Synthesized by Hydrothermal Method
-
Vijaya Saritha1, S Dastagiri1, M. V. Lakshmaiap*, V. Ramesh Kumar2, K.E.Supriya1, and G. Pakardin2
1Department of Physics, Sri Krishnadevaraya University, Anantapuramu, A.P, India.
2Department of Physics, PSC & KVSC Govt. Degree College, Nandyal, A.P, India.
3Department of Physics, Govt. Degree College (A), YSR Kadapa, A.P, India.
Abstract:- ZnTiO3 is a promising wide-energy band gap semiconductor material because of its current and potential uses in catalysts, microwave insulators, luminous materials, nonlinear optics, solar cells, and gas sensors. In the present work, ZnTiO3 nanoparticles were synthesized by hydrothermal method. The structural properties and parameters have been investigated by using X-ray diffraction (XRD). The XRD results raveled that the particle attributed high phase purity, and good crystalline. The structure of ZnTiO3 nanoparticles found cubic spinel phase and using the Scherrer equation the average crystallite size was calculated as 31.74 nm. The X-ray peak broadening analysis was used to evaluate the crystalline sizes and lattice strain by the Williamson-Hall (W-H) analysis. The functional group information conforms to the ZnTiO3 nanoparticles. The FE-SEM images showed the particle shape spherical with partial agglomeration. Furthermore, the optical band gap (Eg) was determined using UVVisible spectra.
Keywords: XRD, FTIR, UV-Vis, Hydrothrmal Method and Optical band gap
-
INTRODUCTION
-
The development of nanomaterials has been strongly pursued due to their unique features such as electronic, chemical, mechanical, magnetic, and optical properties that differ from bulk materials [111]. Size-dependent properties are observed such as surface plasmon resonance in some metal particles, quantum confinement in semiconductor particles, and superparamagnetism in magnetic materials. Among the different nanomaterials, perception of the behavior of ferroelectric materials at the nanoscale is important for the growth of molecular electronics. Indeed, perovskite-phase mixed-metal oxides are significant for their advantageous electrostrictive, pyroelectric, piezoelectric, electro-optic and dielectronic properties with corresponding applications in the electronics industry for high-k- dielectrics, actuators, and transducers [12, 13]. Among perovskite-phase mixed-metal oxides, ZnTiO3 has been reported to have superior electrical properties that are sufficient for applications towards microwave dielectrics [14]. Furthermore, ZnTiO3 is an effective photocatalyst because this compound is categorized in a group of
coupled photocatalysts that can help to enhance the photocatalytic activity of TiO2 through reducing recombination process and change band gap to enhance the optical response in the UV to the visible light range [15, 16]. Pure h- ZnTiO3 represents superior dielectric properties in the microwave range. Many reports have been made to produce pure ZnTiO3 powders and ceramics. But the synthesis of pure ZnTiO3 from a mixture of ZnO and TiO2 with a molar ratio of 1 was not successful. There are various problems with the synthesis of pure ZnTiO3 powders by the solid-state reaction method such as large particle size, a limited degree of chemical homogeneity, high temperature, and long range of diffusion distance. In the past two decades, the sol-gel route has been successfully used for the preparation of ceramics, thin films, fibers, and glasses; this method has various advantages such as low calcination temperature, low cost, chemical homogeneity, and easy component adjustment. Besides, the sol-gel route is one of the methods for the synthesis of nanomaterials [17, 18]. Here, ZnTiO3 nanostructures were synthesized by an unusual sol-gel method. Utilizing new and smaller number chemical materials in addition to a simple procedure is the characteristic benefit of this method in assessment to the other reports [19, 20]. In this paper, ZnTiO3 nanoparticles were successfully synthesized by hydrothermal method. The products were characterized by various analyses such as XRD, SEM, TEM, EDS, FT-IR, and UV-Vis
spectroscopy.
-
EXPERIMENTAL
To synthesize the ZT nanoparticles, the starting materials were chosen as Zn (NO3)2 6H2O and TiO2 (each of 99.9% purity, Sigma-Aldrich). These precursors were mixed after taking their stoichiometric ratio. The whole mixed precursors were transferred to a glass beaker. Furthermore, distilled water was added to the precursors in a ratio of 1:4 (mixed precursors (gm): distilled water (ml)) and the resultant solution was kept on a magnetic stirrer. A stirring rate of 500 rpm was maintained to stir the solution. Later NaOH solution was slowly added, and the pH value reached 11. Furthermore, this solution was transferred to a 500 ml Teflon bowl
inserted in an autoclave. The sealed autoclave was kept in a hot-air oven at an operating temperature of 150°C/8 hours. After completion of the reaction, the autoclave was slowly cooled to room temperature. The final ZnTiO3 nanoparticles were removed from the Teflon-lined autoclave and washed with acetone and distilled water several times until the pH was reduced to 7. Later it was heated at 60°C/2 hour. The final ZT new particles were removed and characterized for various characterized techniques such as the X-ray diffraction method, field emission scanning, and transition electron microscopy. In addition, the Fourier transform infrared spectroscopy (FTIR), and UV-Visible techniques were used to find the presence of metal oxide bands and the optical energy band gap.
-
Results and discussion
structural parameters such as inter-planer spacing (d), FWHM, micro-stain (), miller indices (h k l), and dislocation density () are listed in Table 1 as a function of diffraction angle.
ZT |
|||
(211) (300) (310) (311) (320) (400) (331) (422) (510) (511) (440) |
16.0k
12.0k
Intensity (a.u)
JCPDS: 39- 0190 (Cubic)
8.0k
4.0k
-
XRD analysis
The diffraction pattern of ZT nanoparticles is depicted in Figure 1. It can be seen that the Zinc titanate (ZT)
0.0
20 30 40 50 60
2 degree)
2 (°)
FWHM (°)
d (Ã…)
Da (nm)
hkl
(m2)
25.3579
0.1861
3.509
0.003609
43.7528
211
5.22E+14
31.8093
0.2630
2.810
0.004027
31.4075
300
1.01E+15
34.4708
0.2090
2.599
0.002940
39.7828
310
6.2E+14
36.2927
0.2743
2.473
0.003652
30.4756
311
1.08E+15
37.8497
0.2182
2.375
0.002776
38.4871
320
6.75E+14
47.8762
0.8057
1.898
0.007919
10.7881
331
8.59E+15
53.9284
0.2442
1.698
0.002095
36.4878
422
7.51E+14
55.0872
0.2501
1.665
0.002092
35.8263
510
7.79E+14
56.6124
0.3383
1.624
0.002741
26.6667
511
1.41E+15
62.8195
0.3924
1.478
0.002803
23.7221
440
1.78E+15
nanoparticles exhibit the cubic perovskite structure about the reflection planes as indicated in Figure 1. These reflection planes are in good agreement with the Joint Committee on Powder Diffraction Standards (JCPDS) data of file number: 390190. Amongst these planes, the 211 planes at 25.3579° revealed the maximum intensity. No secondary peaks are observed in the ZT structure. The average crystallite size (Da) is evaluate with the help of the average full-width at half maxima (FWHM) of reflection planes using the Debye-Scherrer equation [22],
Da = (k) / Cos (1)
Where is full-width half maxima, is the wavelength of CuK radiation (0.15406 nm), is the diffraction angle, and "k" is a numerical constant that is equal to 0.9 for a spherical atom. The results are repeated in Table 1. It is clear from the table that the average crystal size is Da is calculated to be 31.74 nm.
Fig. 1. The diffraction pattern of ZT nanoparticles.
Table 1. The data on structural and physical parameters of ZT nanoparticles
Figure 2, depicts the variation of Da and micro-strain as a function of diffraction angle. It is very clear from the figure that there exists a reciprocal relationship between micro- strain and Da. Similar kinds of observations were previously reported in the literature [23]. In addition, the lattice constant (a) is calculated after finding the inter- planar spacing (d) and miller indices (hkl) by using the following formula [23]:
a = d (p+k2+l2)1/2 (2)
The lattice parameter is found to be 8.391 Ã…. The X-ray
45 Crystallite size (Da) nm
Micro-Strain ()
Crystallite size (Da) nm
40
35
30
25
20
15
10
20 25 30 35 40 45 50 55 60 65
2 degree)
0.008
0.007
Micro-Strain ()
0.006
0.005
0.004
0.003
0.002
density (Dx) is evaluated using the formula Z M.W/NV, where Z is the number of molecules per unit cell (Z = 8), M.W is the molecular weight of the composition, N is Avogadros number (6.023 x 1023), and V is the Volume [23]. The calculated numerical value of Dx is 3.517 g/cm3 of ZT nanoparticles [24]. This may be attributed to the high homogeneity of prepared ZT nanoparticles. Moreover, the
Figure 2. The variation of micro-strain and Da of ZT nanoparticles
The inter-planer spacing is decreasing with an increase of 2 angle. In addition, the dislocation density is a parameter that evaluates the defects per unit area of the sample. The smaller value of indicates the low porous structure of ZT nanoparticles and vice versa. In the current study, the
average is ~ 1.72 × 1015 m2. The micro-strain at an average of all diffraction angles is noticed as 0.00346. The specific surface area (S) is a significant physical parameter for nanoparticles and is computed using the relation 6000/DaDx, where the symbols have their usual meaning [25]. In this study, the S is achieved to be ~ 54 m2/g. This kind of high value of S can be attributed to the smallest value of Da when compared with bulk materials. This larger S value in turn affects the electrical, optical, and morphological properties of nanoparticles.
WilliamsonHall (WH) plot (as shown in Figure 3) is drawn for cos versus 4sin to evaluate micro-strain () and crystallite size (Dp) using the following relation [26],
0.008
0.006
Cos
0.004
0.002
ZT
y = 0.00131 x + 0.00227
Cos = (0.9/D) + 4 Sin (3)
where the slope of a straight line offers micro-strain while Da is associated with to intercept parameter. This provides the correlation between strain and size of the crystallite. The results from WH plot express that the stain (0.00131) is almost consistent with the Scherrer strain from the diffraction pattern. The average Da is of the order of 61.08 nm which is almost in agreement with the Scherrer size.
-
Surface morphology
The surface morphology of ZT nanoparticles is analyzed by a field emission scanning electron microscope (FESEM) and a High-Resolution transmission electron microscope (HRTEM). The FESEM photographs of ZT nanoparticles are shown in Figure 4(a). From Figure 4(a), it is noticed that ZT shows well-defined spherical shapes and rods. The grain size (Ga) is determined using the linear intercept method [25]
0.8 1.2 1.6 2.0 2.4
4 Sin
Figure 3. The WH plot of ZT nanoparticles
Ga =
(4)
where L is the line length, N is the number of grains
intercepting the test line, and M is the magnification. The average grain size (Ga) is 135.03 nm. This confirms the presence of microspheres and rods. The energy dispersive X-ray analyzer (EDAX) (Figure 4(c)) reveals the presence of Zn, Ti, and O elements. HRTEM generally provides information about the existence of nanoparticles. The HRTEM images of ZT nanoparticles are shown in Figure 4(b). It is seen from Figure 4(b) that all ZT nanoparticles are spherical in shape. The average particle size is 112.57 nm. In HRTEM images a weak agglomeration is identified.
Figure 4. (a) The FESEM photos of ZT nanoparticles
Figure 4. (b) The HRTEM with SAED photos of ZT nanoparticles.
Figure 4. (c) The EDAX of ZT nanoparticles.
-
Optical Properties
-
FTIR analysis
In order to study the characteristic vibration bands corresponding to different bonds, the FT-IR spectra (Fig. 5) is used in the spectral range of 4004000 cm-1. It is well known that the characteristic vibration bands corresponding to metal-oxygen bonds be in the range of 400700 cm-1. As seen from the spectrum, peaks 464.84
cm-1 and 634.58 cm-1 are the characteristic bands of ZnTiO3, corresponding to the stretching vibration of the ZnO and TiO bond [26]. Also, in the bonds at 1529.41
2090.28 cm-1 and 2337.433742.81 cm-1 wave number range, the peaks were tailored owing to the OH stretching and bening vibrations of H2O molecules absorbed by the ZT nanoparticles [27]. So, the FT-IR spectrum confirms that ZnTiO3 nanostructures were produced according to appeared peaks.
ZT
100
3742.81
2090.28
Transmittence ( % T)
80
2337.43
1529.41
60
634.58
464.84
40
20
4000 3500 3000 2500 2000 1500 1000 500
Wavenumber cm-1
Figure 5. The FTIR spectra of ZT nanoparticles
-
UV-visible spectral analysis
-
The room temperature UVVis absorption spectra of ZnTiO3 nanoparticles were also measured in the range of 2001600 nm. Fig. 6 (a) shows the diffuse reflection adsorption spectra (DRS) of the ZnTiO3 nanoparticles at room temperature. The figure indicates that the ZnTiO3 nanoparticles show absorption maxima at 312.04nm, the direct optical band gap estimated from the absorption spectra of the ZnTiO3 nanoparticles is shown in Fig. 6(b). An optical band gap is obtained by plotting (h)2 vs h where is the absorption coefficient and h is photon energy. Extrapolation of the linear portion at (h)2 = 0 gives the band gaps of 3.19 eV for perovskite ZnTiO3 nanoparticles. The optical band gap of ZnTiO3 nanoparticles is smaller than those of the bulk ZnTiO3 (band gap 3.70 eV) [29].
m = 312.04 nm
1.6
Absorbance (a.u)
1.2
0.8
0.4
0.0
ZT
400 800 1200 1600
Wavelength(nm)
[2] M. Dadkhah, M. Salavati-Niasari, N. Mir, Synthesis and characterization of TiO2 nanoparticles by using new shape controllers and its application in dye-sensitized solar cells, Journal of Industrial and Engineering Chemistry, 20: (2014) 4033-4039 [3] M. Sabet, M. Salavati-Niasari, Deposition of cadmium selenide semiconductor nanostructures on TiO2 surface via different chemical methods and investigation of their effects on dye- sensitized solar cell efficiency, Materials Science in Semiconductor Processing, 27 (2014) 610-619. [4] N. Mir, M. Salavati-Niasari, TiO2 nanoparticle aggregations prepared by nitro-functionalized tripodal ligand as promising candidates for dye-sensitized solar cells, Materials Science in Semiconductor Processing, 27 (2014) 695-702. [5] M. Sabet, M. Salavati-Niasari, O. Amiri, Using different chemical methods for deposition of CdS on TiO2 surface and investigation of their influences on the dye-sensitized solar cell performance, Electrochimica Acta, 117 (2014) 489-504.Figure 6(a). The absorption spectra of ZT nanoparticles.
ZT
400
(h (eV Cm-1)2
300
200
100
Eg = 3.19 eV
0
1 2 3 4 5 6 7
Photon energy (eV)
Figure 6(b). The h versus photon energy of ZT nanoparticles.
4. CONCLUSIONS
The hydrothermal method in comparison to other synthetic methods of nanoparticle preparation has many advantages, for example: simple, available, and inexpensive. In this work, we have prepared the ZnTiO3 nanoparticles by hydrothermal method. A systematic study on the structural, morphological, and optical properties of ZnTiO3 nanoparticles was carried out using various analyses. FESEM images reveal that the ZnTiO3 have a mean particle size of about 135.03, with spherical-like shapes and rods. The average particle size was 112.57 nm using HRTEM images. The band gap was 3.19 eV estimated by UV-vis spectroscopy.
ACKNOWLEDGMENT
My special thanks go to SRMIST consultancy, SRM University, Chennai for providing me the necessary characterizations (XRD, FTIR, and UV-Vis), SEM with EDAX providing Yogi Vemana University, YSR Kadapa, and HRTEM with SAED providing STIC, Cochin University, Kerala.
REFERENCES:
[1] Y. Xiong, B. Wang, W. Hu, L. Dai, Facile synthesis and characterization of erythrocyte-like Y-doped PbWO4 mesocrystals and their photocatalytic activity, Materials Characterization 105 (2015) 2429. [6] N. Mir, M. Salavati-Niasari, Photovoltaic properties of corresponding dye-sensitized solar cells: Effect of active sites of growth controller on TiO2 nanostructures, Solar Energy, 86 (2012) 3389-3397. [7] M. Masjedi, N. Mir, E. Noori, T. Gholami, M. Salavati-Niasari, Effect of Schiff base ligand on the size and the optical properties of TiO2 nanoparticles, Superlattices and Microstructures, 62 (2013) 21-30. [8] S. Mandizadeh, M. Bazarganipour, A low-cost and eco-friendly viable approach for green synthesis of barium hexaferrite nanostructures using palm oil, M. Salavati-Niasari, Ceramics International, 40 (2014) 15680-15685. [9] K.J. Klabundl, Nanoscale Materials in Chemistry, Wiley- Interscience, New York, 2001 51-55. [10] A.P. Alivisatos, Semiconductor clusters, nanocrystals, and quantum dots, Science, 271 (1996) 933-937. [11] M. Nirmal, L.E. Brus, Luminescence photophysics in semiconductor nanocrystals, Acc. Chem. Res. 32 (1999) 407-414.
[12] N.A. Hill, Why Are There so Few Magnetic Ferroelectrics, J. Phys. Chem. B 104 (2000) 6694-6709. [13] A.J. Millis, Lattice effects in magnetoresistive manganese perovskites, Nature, 392 (1998) 147-150. [14] S. Ke, X. Cheng, Q. Wang, Y. Wang, Preparation ofaphotocatalyticTiO2/ZnTiO3 coating on glazed ceramic tiles, Ceramics Internationa l40 (2014) 88918895. [15] H. Xu, S. Ouyang, L. Liu, P. Reunchan, N. Umezawa, J. Ye, Recent advances in TiO2-basedphotocatalysis, J. Mater. Chem. A, 2 (2014) 12642-12661. [16] C.F. Shih, W.M. Li, M.M. Lin, K.T. Hung, Microelectrode Study of Pore Size, Ion Size, and Solvent Effects on the Charge/Discharge Behavior of Microporous Carbons for Electrical Double-Layer Capacitors, J. Electrochemical Soc. 156 (2009) 7-12. [17] F. Ansari, F. Soofivand, M. Salavati-Niasari, Utilizing maleic acid as a novel fuel for synthesis of PbFe12O19 nanoceramics via solgel auto-combustion route, Materials Characterization, 103 (2015) 11-17. [18] S. Mandizadeh, F. Soofivand, M. Salavati-Niasari, Solgel auto combustion synthesis of BaFe12O19 nanoceramics by using carbohydrate sugars as a novel reducing agent, Advanced Powder Technology 26 (2015) 1348-1354. [19] S.F. Wang, F. Gu, M.K. Lu, C.F. Song, S.W. Liu, D. Xu, D.R.Yuan, Preparation and characterization of solgel derived ZnTiO3 nanocrystals, Materials Research Bulletin 38 (2003) 1283-1288.
[20] Y.S. Chang, Y.H. Chang, I.G. Chen, G.J. Chen, Y.L. Chai, Synthesis and characterization of zinc titanate nano-crystal powders by solgel technique, Journal of Crystal Growth 243 (2002) 319326. [21] W.G. Klemperer, S.D. Ramamurthy, Molecular growth pathways in silica solgel polymerization, vol.121, Pittsburgh, PA,. in: C.J. Brinker, D.E. Clarke, D.E. Ulrich, Material Research Society Symposium Proceedings, (1988) 1-33. [22] Maddaiah M, Naidu KCB, Rani DJ, et al. Synthesis and characterization of CuO-doped SrTiO3 ceramics. J Ovonic Res. 2015; 11:99106. [23] Chandra Babu Naidu K, Madhuri W. Microwave processed NiMg ferrite: studies on structural and magnetic properties. J Magn Magn Mater. 2016;420:109116. [24] Reddy VN, Babu Naidu KC, Subbarao T. Structural, optical and ferroelectric properties of BaTiO3 ceramics. J Ovonic Res. 2016;12:185191. [25] Chandra Babu Naidu K, Madhuri W. Microwave assisted solid state reaction method: investigations on electrical and magnetic properties NiMgZn ferrites. Mater Chem Phys. 2016; 181:432 443. [26] H.Q. Fan, H.E. Kim, Japanese Journal of Applied Physics, 41 (2002) 6768 6772 [27] Tai Duc Tran & Moon Il Kim, Organic-Inorganic Hybrid Nanoflowers as Potent Materials for Biosensing and Biocatalytic Applications. Bio Chip J. 12, 268279 (2018)