
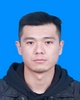
- Open Access
- Authors : Hang Ji , Wentai Bi , Jiaqi Yan , Shengwei Wu
- Paper ID : IJERTV12IS030081
- Volume & Issue : Volume 12, Issue 03 (March 2023)
- Published (First Online): 23-03-2023
- ISSN (Online) : 2278-0181
- Publisher Name : IJERT
- License:
This work is licensed under a Creative Commons Attribution 4.0 International License
ICTs-Driven Agriculture Contributes to the Mission of Carbon Reduction
Hang Ji
College of Economics and Management Henan Agricultural University
Henan, China
Jiaqi Yan
Department of Economics
National University Higher School of Economics Moscow, Russia
Wentai Bi
College of Economics and Management Henan Agricultural University
Henan, China
Shengwei Wu
School of Arts and Design Wenshan University Yunnan, China
Abstract ICTs drive agriculture to be more productive, sustainable and green. In the context of the global carbon reduction mission, how the agriculture industry will contribute becomes the main target of our observation. This paper surveys the global research findings on how digital technologies can contribute to modern agriculture and provides an in-depth discussion on the key technologies, key approaches and methods for digitally driven agriculture to help reduce carbon emissions. The study finds that ICTs-driven digital agriculture has an impact on carbon emission reduction from two main perspectives: digital technologies improve agricultural production efficiency and reduce carbon emissions; digital technologies better enable the measurement, labelling and trading of agricultural carbon sinks, which indirectly has a positive impact on global carbon management. The main ICT technologies mainly include the Internet of Things, blockchain, big data technology and cubic planting technology, on top of which we designed an agricultural resource management platform that can support the overall management of carbon resources and the measurement of green efficiency in agriculture.
Keywords: ICT; Digital Agriculture; Internet of Things; Carbon Reduction
-
INTRODUCTION
Food is the head of government, and an adequate and stable supply of food is the basic guarantee of world peace [1]. The international situation is volatile, with frequent climate extremes striking the region, greatly affecting agricultural production and trade, and further affecting regional security and stability. Generally, the challenges in agriculture include
1) The low level of education of rural workers leads to low
agricultural productivity [2]; 2) Impacts of climate variability and extreme weather on cereal production [3]; 3) Decrease in arable land and increase in food demand as the global population grows [4][5]; 4) Land area decrease with urbanization.
In general, there are 3 ways to reduce costs and increase efficiency in agriculture: 1) Improve land usage efficiency, including but not limited to large-scale land transfer, development of cubic agriculture, and cooperative economy;
2) Increase scientific and technological participation in agriculture, including but not limited to development of
advanced breeding technology, advanced field management ( such as drip irrigation technology, precision fertilization), and adoption of advanced agricultural machinery; 3) Establish globalized and strict sustainable development response strategies, such as carbon reduction targets, climate finance, etc. And Intelligent and Sustainable greenhouse farming provides the possibility for the above: using industrialized/commercialized means to set new goals for agricultural development, bringing together green energy systems, cubic multi-level production systems, intelligent and refined operation management systems, and Green Financial Aid systems on limited space, making food production more efficient, Greenhouse gas negative emissions, and agricultural operations more efficient. Provide more surplus labor for society and more healthy food, while having a positive impact on climate change.
This paper investigates digital involvement in agriculture, concludes the trends of digital agriculture, and argues that digitally driven agriculture will contribute to the UN Sustainable Development Goals (SDGs), especially in the area of climate change.
Based on desk analysis and a survey of real-world cases, we recognize IoT, SaaS platforms and Robotics as three core technologies, in addition to the importance of artificial intelligence (AI), drones and other technology applications. This mainly depends on two different technology paths: large- scale farms and modular greenhouses. The large-scale farm will involve more weather forecasting, remote sensing detection of crop growth and automated operating machines, while greenhouse farming reduces the reliance on forecasting technology and large intelligent equipment because more trace data and higher certainty are collected, while the operating platform is small and space is limited.
We propose a new architecture containing three subsystems: Solar Harvesting, Distributed Grid, Geothermal Circulation, Biogas Production, Cubic Integrated Production, and Carbon Trading, and dismantle the technology from four perspectives: " Device Network – Cloud – Application".
The second chapter of this paper analyzes current trends in agriculture, and cases of digital technology empowering modern agriculture. Next, we elaborate on the framework
model, an ICT-driven, climate-friendly, integrated governance system for digital agriculture in the third chapter. Then in the fourth chapter, we discussed the essential challenges and opportunities and concluded.
-
RELATED WORKS
-
Several trends of development of modern agriculture
-
Agriculture has been transformed from a "subsistence" to an open, profit-making economic entity. The land is the most primitive and fundamental factor of production and is often used as a tool for capitalists to exploit and enslave others. Therefore, with the development of human civilization, the land was distributed to individuals or families in a decentralized manner, and obviously, the goal of agricultural production was to provide food for the whole family. Except for a few countries and regions where there was substantial land affluence and therefore the ability to provide food for others, most countries and regions of the world were not yet able to guarantee basic food self- sufficiency. However, with advances in agricultural technology and the lack of growth in emerging markets, agriculture is gradually changing from a "food security base" to a "profit-making business".[6] This change in thinking is the fundamental driving force behind the emergence of digital technology, new economic organization models, new agricultural business models and "new farmers".
-
Horizontal intensification (cooperatives) and vertical integration (deep processing, whole industry chain) in the agriculture-related industry. Since agriculture has just undergone the transformation from "self-sufficiency" to "commercial profitability", most agricultural production is still at the stage of positive scale efficiency, therefore, driven by efficiency and profit, the agriculture-related industry has shown the basic characteristics of integration and expansion in both horizontal and vertical directions. expanded base characteristics. Originally farmers and producers relied on low-frequency buying and selling to maintain cooperation, and a series of production alliances or consumer alliances, cum cooperatives, have been derived to enhance their interests. Members act in concert to increase bargaining power or share costs against risk [7]. At the same time, to increase the transparency of the production supply chain and the added value of primary agricultural products, food processing companies and agricultural supply companies are coincidentally expanding upstrea and downstream of the industry chain to create a perfect benefit linkage system, to take in higher profits or more stable income [8]. Whether horizontal or vertical cooperation, agriculture-related industries have promoted the development and promotion of multiple mutually beneficial systems in the agricultural industry, such as cooperative units and contract farming models.
-
Agriculture becomes a technology-intensive industry (application of advanced technologies). Modern farms and agriculture show a completely different shape than before. Benefiting from the changes in agricultural development thinking and agricultural cooperation mechanisms, it has become possible to adopt high technology on a large scale for cost reduction and efficiency. Firstly, through various sensors and remote sensing devices, new farmers no longer need to
estimate and judge the state of agricultural operation through naked-eye observation; Secondly, based on information technology, intelligent devices and robots, farmers no longer need to fertilize, water and kill pests evenly on the whole field; through the assistance of intelligent systems and tools, new farmers can carry out field management and resource allocation with the least cost and the most accuracy; finally, through the use of advanced auxiliary tools, human capabilities will be greatly expanded to enable agricultural production to expand into areas that are difficult to reach by traditional farmers and overcome the constraints of natural conditions. The ultimate goal is to achieve maximum crop production, less use of fertilizers and pesticides, less negative environmental impact, a safer working environment and a safer food supply.
-
-
Example of technology and solutions in digital agriculture
Agricultural automation and robots are beginning to gain traction among farmers as demand grows and labor shortages occur around the globe. In the U.S. alone, crop production is decreasing by an estimated $3.1 billion per year due to labor shortages. ICT-driven digital agriculture can not only mitigate labor shortages but more importantly, use advanced predictive and decision-making technologies to conduct agricultural production more efficiently. We will explain the technology and applications in digital agriculture in the following scenarios.
-
Cubic Production System: Cubic agriculture also named container farms grow crops in repurposed shipping containers and often uses vertical farming techniques. Cubic agriculture refers to the clever use of crop space, time differences and other biological characteristics to implement cubic planting and mixed stereo-culture methods such as intercropping, mixing, spread, hanging and framing, so that multiple crops can match and circulate with each other in an ecosystem. The earliest Cubic Production System named "mulberry-based fish ponds", "cane-based fish ponds" or "fruit-based fish ponds", which can be traced back to more than 2,000 years ago, and they use rivers and low-lying land to dig ponds to cultivate the foundation, ponds to raise fish, the base surface planting mulberry, cane, fruits, vegetables or forage [9]. Cubic agriculture effectively uses natural resource conditions to achieve increased yields and quality, significantly increases the economic value generated by crops, and improves soil physical and chemical properties. This approach is often combined with greenhouses where, with the help of IoT sensors, farmers can obtain more accurate information about greenhouse conditions, such as lighting, temperature, soil conditions and humidity, allowing for manual intervention in the greenhouse environment. And then maximize the use of spatial resources and counteract different seasonal conditions, thus maximizing economic benefits. A typical cubic production system would also
include the following 5 subsystemsShown in Figure 1
-
Lighting System Lighting and supplemental light for plants in the shed to promote growth, or insect trap lights to reduce pests for pesticide-free spraying
-
Water Pump System Provide water and power for sprinkler and drip irrigation performed for crop watering, cooling, fertilizing or insect killing
-
Temperature Control System Heaters are used to achieve a more suitable growing environment for the crop, especially in winter or at night when the temperature is low
-
Air System Fans are used to create a natural breeze or natural air circulation effect, and to replenish carbon dioxide needed for plant growth, etc.
-
Sensor Network Monitor temperature, humidity and light level of the air and soil inside the shed, and the temperature, humidity, wind speed, wind direction, light level and other environmental changes outside the shed at all times
Figure 1. Cubic Production System
-
-
Precision Agriculture and Variable Rate Technology Precision agriculture (PA), also known as
"prescription farming" or "variable rate technology"[10]. Again, PA is not a single technology, but rather a solution that encompasses a suite of technologies. PA is often tied to time, space, etc., and emphasizes the heterogeneity of different crops so that management techniques can be implemented to differentiate policies. It is based on the combination of soil, climate, and plant characteristics detected and detected by sensors, which are matched with location information to carry out specific replenishment for specific locations based on a model of the ideal impact requirements of the crop, thus ensuring optimal growth in each area, for each crop, at each moment. Variable Yield Technology (VRT) describes any technology that enables producers to vary crop input rates. VRT combines variable rate control systems with application equipment to apply inputs at precise times and/or locations to achieve site- specific input application rates. VRT can be classified by
category
-
Fertilizer VRT
-
Crop Protection Chemistry VRT
-
Soil Sensing VRT
-
Seeding VRT
-
Yield Monitor VRT
-
Irrigation VRT
-
-
Global Positioning System The Global Positioning
System GPS provides accurate geographic location, vehicle speed and precise time information anywhere in the
world and near-earth space. When GPS modules are installed in tractors, combines, sprayers, etc., the sexy thing happens: just enter the range coordinates and the machine automatically starts working; the user tells the onboard control system via collection or laptop, sets the path, and the machine follows the intelligent path autonomously [11]. This not only allows the operator to avoid driving but also to avoid human emotions through mechanical trust, which is very important in some scenarios. For example, avoiding repeated sowing and maintaining a constant furrow spacing. This will greatly improve the efficiency of farm cultivation and reduce the loss of seeds, pesticides and fertilizers, and it has proven to be easier to trust a machine than a person.
-
-
Sustainable Agriculture and Carbon Reduction
Carbon fixation by plants through photosynthesis is by far the largest contributor to climate change [12]. However, when we discuss carbon fixation by photosynthesis, we often do not count the amount of carbon fixed by crops, because the carbon fixed by photosynthesis of crops eventually returns to nature through edible decomposition or humus decomposition. Therefore, the contribution of agriculture to carbon emission reduction will only be realized from the following three paths: 1, green energy storage projects without affecting crop production; 2, scientific and technological inputs to reduce direct carbon emissions in the agricultural production process; 3, green agriculture access financial market. Therefore, it also gave birth to the "photovoltaic + agriculture" modern agricultural model.
Photovoltaic agricultre will combine the organic combination of traditional agricultural planting and photovoltaic power generation to achieve more efficient energy collection and more efficient food output by making full use of the vertical space of the land. Photovoltaic agriculture does not change the nature of the land and uses industrial and high-tech means to promote modern and efficient agriculture, including agricultural container planting and breeding, VTR irrigation, fertilization, harvesting, pest control and agricultural machinery power. On the one hand, photovoltaic power plants can use low-cost agricultural land to generate electricity directly or to supplement light at night; on the other hand, photovoltaic power generation systems, photothermal systems and new nano-biomimetic photovoltaic film technology are integrated and grafted into traditional greenhouses to convert wavelengths for crop absorption and enhance photosynthesis according to the needs of different plants growing on different wavelengths of light.
At present, " Photovoltaic + Agriculture" mainly contains 3 types (as shown in Figure 2).
-
Fishery-photovoltaic:[13]PV equipment is mainly built on the lake or sea surface, and the light distribution system is adjusted according to the light characteristics. At the same time, it supports the growth of fish resting or other aquatic products under the light.
-
Agro-photovoltaic:[14]It mainly combines the production activities of vegetables, mushrooms, forestry and poultry with the photovoltaic electric field. It can not only solve the decomposition of solid waste organisms, but also improve the physical and chemical environment of planting soil, and can better realize the development of precision agriculture and low carbon production.
-
Pastoral-Photovoltaic:[15][16]This model is mainly applicable to pasture areas with poor land. By adopting a high bracket and adjustable tilting angle photovoltaic bracket system, the upper space is used for photovoltaic power generation, which covers less than 35% of the land, while the
-
lower space is used for staple food planting or livestock breeding such as cattle and sheep, and can realize small and medium-sized mechanical farming, supporting water-saving irrigation system and livestock growth monitoring system.
Figure 2. Schematic diagram of Photovoltaic Agriculture based Internet of Things [17]
Photovoltaic Agriculture gives full effect to the comprehensive land use benefits through the superposition of industries to achieve SDGs: improve agricultural management, effectively use land resources, higher crop productivity, and break the land dilemma of photovoltaic power generation. With 40MW installed capacity, it can generally cover 177 triplex greenhouses (each covering an area of 2200 square meters) totaling 430,000 square meters of standing land, which can reduce 56,800 tons of carbon dioxide and 477.6 tons of sulfur dioxide per year (as shown in Table I). Equivalent to planting nearly 520,000 trees and nearly 37,800 economy cars stopping driving for one year.
circulation, we provide energy management for planting or breeding; the cubic integrated production system provides multi-level and multi-dimensional planting and breeding of different crops and livestock; the biological humus is accelerated and decomposed by biogas production system and used to improve soil physicochemical environment; the excess energy and carbon credits are traded in the financial system. The combination of "green energy – green production – green finance" can not only greatly reduce carbon emissions in agricultural production, but also accelerate carbon emission
reduction through financial instrumentsshown in Figure 3
.
TABLE I. PHOTOVOLTAIC AGRICULTURE OUTCOMES A
Items
Units
Value
Annual power generation
kWh
70,000,000
Saving standard coal
tons
21,600
Emission reduction of CO2
tons
56,800
Emission reduction of sulfur dioxide
tons
477.6
Emission reduction of nitrogen oxides
tons
160.8
Emission of soot
tons
325.6
A. Take the installed capacity of 40 MW as an example
-
-
TECHNICAL FRAMEWORK FOR ICT-DRIVEN DIGITAL AGRICULTURE
-
System Architecture
We offer a new framework, an ICT-driven integrated governance system for modern agriculture. By integrating two subsystems: solar energy harvesting and geothermal
-
Energy Management Module. Connect two energy systems, solar and geothermal, and realize the functions of energy collection, situational awareness and energy distribution. Including but not limited to the light sensing subsystem, thermal cycle monitoring subsystem, thin film light splitting subsystem, plant supplemental light subsystem, temperature control subsystem, etc.
-
Cubic Agriculture Module. Above-ground multi-level crop planting is achieved by matching the constraints of different plants on light, temperature and air conditions; this can be supplemented by breeding or special categories of plants planted above or below ground. A typical cubic agriculture scenario is to grow sun-loving cash crops on the bottom level, conventional vegetable cultivation on the middle level relying on supports, shade-loving and wind- sheltered crops or poultry farming on the ground level, and earthworm farming and potato cultivation on the ground level. Likewise, the substrate can be a fish pond.
-
Green Finance Module. The green financial system is what distinguishes our discussion from traditional pure engineering. Numerous pieces of evidence show that the introduction of green financial tools will facilitate the application of more capital, talent, and technology in agriculture, and provide the impetus for green and sustainable agricultural development. Just as Silicon Valley Bank has
supported the entire world innovation valley, green agricultural tools will also provide stable financial support, sufficient green incentives, and a foundation for higher efficiency development in agriculture [18].
-
-
Detailed Modular Design
Figure 3. ICT-driven Integrated Governance System for Digital Agriculture
instructions to the workbench on time. This reduces the
From another perspective, we can have a clearer understanding of ICT-driven digital agriculture. End-point – Channel – Cloud service Platform – Scenarios. This would be a clearer way of thinking to understand our framework. We can also call it Device Network Cloud – Application(shown in Figure 4).
-
Device. Here the device includes two types, sensors and a workbench. But here we are only interested in all information acquisition and situational awareness functions and do not consider the intervention role of the device. This includes temperature sensing (in the air and the soil), light sensing (to support the work of the light-harvesting system, the light splitting system and the light filling system), CO2 and nitrogen sensing, pressure sensing, etc. This is both the sensing of the environment and the monitoring of agricultural work. The many sensors form a sensing network that digitizes and dataizes agriculture so that even a person without any agricultural experience can understand the current process, the ripeness of each tomato, and the optimal growth needs of each apple tree.
-
Network. The Network can be explained as the Channels, sharing information and distributing instructions. Even on farms of hundreds of square miles or inaccessible mountain abysses, advanced networks with low power, high transmission speed, ubiquity, low latency, and high security can deliver inormation to decision-makers and
need for human resources and also expands human
capabilities [19].
-
Cloud. Digital agriculture requires the assistance of ICT not only in data collection and transfer, but also in information processing and decision-making beyond the capabilities of ordinary people. Based on big data, complex systems and multi-objective decision-making dilemmas, cloud storage, cloud computing and cloud services become core tools to solve these. An experienced farmer or scientist only needs to set the desired goal, and then cloud computing can help him parse out the most reasonable task assignment and operation path. For example, let harvesters complete the task of harvesting wheat in the shortest path while ensuring that they do not cause harm to other people's wheat fields, not to mention forfeiting their legitimate gains.
-
Application. Applications can likewise be divided into two categories, one for the issuance of orders (management portal) and one for the execution of orders (workbench). Either scenario focuses only on "human & decision" centered information presentation and instruction execution. For example, an expert can get information about the operation status of a farm from a dashboard, know the growth status of a corn plant from an information map, or give a task order to a harvester from a remote cockpit.
Figure 4. ICT-driven Integrated Governance System for Digital Agriculture
-
-
-
DISCUSSION AND CONCLUSIONS
ICT-driven agriculture transforms traditional agricultural activities into data collection and situational awareness, data transmission analysis and deposition,
data identification and decision-making, and agricultural activity execution and exchange, which changes agriculture dramatically. With the application of advanced ICT such as automated devices, GPS and satellite internet, and drones, agriculture will become a new technological showcase and will have a huge positive impact on agriculture, rural areas, and farmers, and make a huge contribution to the security of food supply, ecological environment, and the climate change for the world.
But while the future of ICT-driven digital agriculture is promising, several challenges have come to light:
-
System Integration is Hard. Agricultural activities exhibit typical regionalization, which is closely related to climate, geography, culture, etc. This has a huge impact on the way on the portfolio of technologies or solutions. Digital agriculture cannot solve all problems, and certainly no uniform solution or standard exists.
-
Youth Participation in Agriculture. Digital agriculture frees humans from agricultural production, but also requires a higher quality of new farmers [20]. Meanwhile, nearly 1 billion of the world's 1.2 billion youth aged 15-24 live in developing countries, with 500 million of these young people living in rural areas [21]. Agricultural skills training, technology extension and development incentives for young people are crucial.
-
Digital Infrastructure in Rural. With industrialization, agriculture has been neglected for a long time. The primary obstacle to the development of digital agriculture is the lack of digital infrastructure, especially for the public, which is critical to the digital transformation of agriculture. There is also an urgent need for new economic systems and models for agriculture, and a better agricultural financial system. Making agriculture a truly profitable industry is not only the future of digital agriculture, it is the fundamental driver of digital agriculture moving forward.
REFERENCES
[1] Shaw, D. John. "World food security." A History since (1945): 7. [2] Oduro-Ofori, Eric, Anokye Prince Aboagye, and Naa Aku Elfreda Acquaye. "Effects of education on the agricultural productivity of farmers in the Offinso Municipality." (2014). [3] Rosenzweig, Cynthia, et al. "Climate change and extreme weather events-Implications for food production, plant diseases, and pests." (2001). [4] Vos, Rob, and Lorenzo Giovanni Bellù. "Global trends and challenges to food and agriculture into the 21st century." Sustainable food and agriculture (2019): 11-30. [5] Bajelj, Bojana, et al. "Importance of food-demand management for climate mitigation." Nature Climate Change 4.10 (2014): 924-929.
[6] Shrestha, Rudra Bahadur, and Devendra Gauchan. "Agribusiness Competitiveness of Smallholder Agriculture for Structural Transformation in South Asia." (2020). [7] Manikas, Ioannis, George Malindretos, and Socrates Moschuris. "A community-based Agro-Food Hub model for sustainable farming." Sustainability 11.4 (2019): 1017. [8] Briones, Roehlano M. The structure of agricultural trade industry in developing countries. No. 2013-15. PIDS Discussion Paper Series, 2013. [9] Rana, S. S., and Pankaj Chopra. "Integrated farming system." Department of Agronomy, College of Agriculture, CSK Himachal Pradesh Krishi Vishvavidyalaya: Palampur, India (2013). [10] Varella, Carlos Alberto Alves, José Marinaldo Gleriani, and Ronaldo Medeiros dos Santos. "Precision agriculture and remote sensing." Sugarcane. Academic Press, 2015. 185-203. [11] Yousefi, Mohammad Reza, and Ayat Mohammad Razdari. "Application of GIS and GPS in precision agriculture (a review)." International Journal of Advanced Biological and Biomedical Research 3.1 (2015): 7-9. [12] Rosgaard, Lisa, et al. "Bioengineering of carbon fixation, biofuels, and biochemicals in cyanobacteria and plants." Journal of Biotechnology 162.1 (2012): 134-147. [13] Li, Peidu, et al. "Effect of the temperature difference between land and lake on photovoltaic power generation." Renewable Energy 185 (2022): 86-95. [14] Mengjing, Hou. "Principle and modeling analysis of an agro photovoltaic system using filter film and light reflection." Journal of Chinese Agricultural Mechanization 43.3 (2022): 127. [15] Sánchez-Pantoja, Núria, Rosario Vidal, and M. Carmen Pastor. "Aesthetic perception of photovoltaic integration within new proposals for ecological architecture." Sustainable cities and society 39 (2018): 203-214. [16] Sánchez-Pantoja, Núria, Rosario Vidal, and M. Carmen Pastor. "EU-funded projects with actual implementation of renewable energies in cities. Analysis of their concern for aesthetic impact." Energies 14.6 (2021): 1627. [17] K. Huang et al., "Photovoltaic Agricultural Internet of Things Towards Realizing the Next Generation of Smart Farming," in IEEE Access, vol. 8, pp. 76300-76312, 2020, doi: 10.1109/ACCESS.2020.2988663. [18] Rhodes, Christopher J. "Feeding and healing the world: through regenerative agriculture and permaculture." Science progress 95.4 (2012): 345-446. [19] Economic Research Office, General Policy Division, Information and Communications Policy Bureau. Information and Communications in Japan : White Paper[R/OL]. [2023-03-11]. https://www.soumu.go.jp/johotsusintokei/ whitepaper/eng/WP2016/2016-index.html. [20] Geza, Wendy, et al. "Youth participation in agriculture: a scoping review." Sustainability 13.16 (2021): 9120. [21] UN Development Program. Fast Facts: Youth and Climate Change. November 2015. Available online: https://reliefweb.int/sites/reliefweb.int/files/resources/FF-Youth- Engagement-Climate%20Change_Nov2015.pdf (accessed on 10 March 2023).Authors background
Your Name |
Title* |
Research Field |
Personal website |
Hang Ji |
PhD candidate |
Smart Agriculture and Economics |
|
Wentai Bi |
PhD |
Agriculture Economics |
|
Jiaqi Yan |
PhD candidate |
Cyber-Physical System and Economics |
|
Shengwei Wu |
Professor |
Development and Economics |