
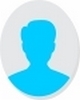
- Open Access
- Authors : Fatma R. Mahmoud , L.M. Abd El-Hafez , Yasser R. Tawfic , Naglaa G. Fahmy
- Paper ID : IJERTV12IS050077
- Volume & Issue : Volume 12, Issue 05 (May 2023)
- Published (First Online): 13-05-2023
- ISSN (Online) : 2278-0181
- Publisher Name : IJERT
- License:
This work is licensed under a Creative Commons Attribution 4.0 International License
Behaviour of Strengthened Columns with Basalt Fiber-Reinforced Polymers:Review
Fatma R. Mahmoud a,*, L.M. Abd El-Hafez b, Naglaa G. Fahmy a, c, Yasser R. Tawfic b
a Department of Civil Engineering, Faculty of Engineering, Nahda University, Beni Suef 0822284688, Egypt
b Department of Civil Engineering, Faculty of Engineering, Minia University, 61519 Minia, Egypt
c Department of Civil engineering, Higher Institute of Engineering and Technology New Minia, Egypt
Abstract- Fiber-reinforced polymer (FRP) composites have found increasingly widespread uses in the strengthening and retrofitting of RC structures during the last two decades. This paper offers a review on FRP materials used as strengthening for RC columns and discuss the properties of them. Also, the paper discuss the parameters affecting performance of columns which strengthening by FRP.
Keywords: FRP, basalt fiber, eccentric load, Square column, aspect ratio.
-
INTRODUCTION
Reinforced concrete (RC) is commonly used in construction all over the world. Columns carry loads from beams and slabs to the foundations. Existing compressed elements in reinforced concrete buildings are often influenced not only by compression, but also by bending moments and shear stresses. When designing eccentrically loaded concrete elements, design recommendations state that tensile stresses can exist in a portion of the cross section, necessitating extra reinforcing. If this impact is not considered, the load-carrying capability of an eccentrically loaded element may be inadequate. In this situation, the element should be strengthened [1]. Furthermore, because of the limited strength and ductility of concrete, columns may sustain damage from natural calamities such as earthquakes and fires. On the other hand, seawater, deicing chemicals, and freeze-thaw cycles can cause structural damage over time. The failure of one or more columns may cause the building to collapse [2, 3].
Since demolishing and reconstruction of RC Structures requires huge investments, strengthening became the most economical solution to improve the carrying load capacity and to assure the serviceability and safety of the structure, which concludes that Strengthening is mostly used for the purpose of increasing the load capacity of the structural elements, but sometimes its harder and more complicated to strengthen the structure than reconstructing of a new one. [4, 5]
Concrete is susceptible to cracking and has poor tensile strength and toughness; addressing these weaknesses in order to extend its service life and assure structural safety is a key challenge [6,7]. Corrosion of traditional steel reinforcing bars in reinforced concrete (RC) members in severe, corrosive coastal settings is also a big problem. Furthermore, the expense of repairing and rehabilitating degraded buildings caused by steel reinforcement corrosion can be high, and the epoxy coating on steel bars may cause a link between the concrete and the steel bars to collapse. To address corrosion issues, fiber-reinforced polymer (FRP) bars were created, which consist of glass, carbon, or aramid fibers wrapped in a matrix of epoxy, polyester, or phenolic thermosetting resins [8,9].
-
DEFINITION REPAIRING AND STRENGTHENING RC STRUCTURAL MEMBERS
Repair: To replace or repair deteriorating, damaged, or defective concrete structural materials, components, or parts. While, strengthening: the process of restoring weakening components or parts to their original design capability or improving the strength of concrete construction components or elements [10].
Retrofitting is the use of FRP materials to reinforce and/or restore load-bearing structural parts in existing structures. There are two sorts of retrofitting applications. The first category is "strengthening," which involves upgrading a structure's original strength or ductility to accommodate new services or levels of loading. This expansion may be required to make the structure comply with existing building codes, or it may be desirable owing to changes in the structure's planned use. The second sort of FRP retrofitting is "repairing." FRPs are employed in the latter situation to repair an existing and deteriorating structure in order to restore its load-carrying capacity, ductility, or stability to the level for which it was designed [11].
One of the most essential challenges in civil engineering is the strengthening of reinforced concrete columns. A change in structural use or the removal of some adjacent load-bearing structural members may be reasons for strengthening, as may degradation of the structure caused by environmental, technical, or other forms of attack, or when the column is sought to be used in a different manner than previously planned, or because it is damaged by external factors during its service [12, 13 ].
Because of the transverse confining stress, concrete confinement is an efficient technique for increasing the load-bearing capacity and ductility of a column. When concrete is exposed to laterally confining pressure, the compressive strength cc and associated strain cc are substantially larger than those of unconfined concrete co and co, as illustrated in Figure (1) [14].
Figure 1 Typical Improved Stress-strain Curve of Confined Concrete [14].
-
STRENGTHENING METHODS
Bonding additional exterior materials to RC structural components is typically utilised to enhance the structural members' shear, flexural, and shear and flexural capabilities, or ductility. Although current strengthening procedures are more expensive than previous methods, they produce much superior outcomes in terms of enhancing the capacity and ductility of the reinforced part [15, 16].
The following are the most frequent strengthening procedures for reinforced concrete columns to boost their carrying capacity against axial loads and bending moments:
-
Column section enlargement using reinforced concrete jackets. This approach is quite inexpensive and does not require any particular building procedures, but the increase in column size acquired once the jacket is formed, as well as the duration of the construction process, make it unappealing.
-
Steel plate jacketing is being used. This approach has shown to be a successful retrofitting strategy and is extensively utilized in practice; nevertheless, steel corrosion in corrosive conditions and the necessity for heavy construction equipment make this method uneconomical. Furthermore, the Poisson's ratio of steel is larger than that of concrete during the early stage of loading, and this differential expansion leads in partial separation of the two materials, delaying the activation of confinement mechanisms. Furthermore, the high modulus of elasticity of steel applies a considerable percentage of the axial stress on a steel jacket, resulting in early buckling.
-
Fiber-reinforced polymer (FRP) has recently emerged as a new material to be used in structural engineering due to its appealing mechanical properties, such as high tensile strength, light weight, high resistance to corrosion, high fatigue endurance, low thermal coefficient, short installation period, easy application, and low maintenance cost [17].
4.1 Steel jacketing
-
MATERIALS OF STRENGTHENING
Concrete and steel jacketing are examples of traditional retrofit techniques. These methods are time-consuming and labor- intensive. They also increase the cross-sectional area of the structural column member. Another recent method of repair is the use
of fiber-reiforced polymers (FRP) due to their excellent mechanical properties, corrosion resistance, and durability, light weight, ease of application, reduced construction time, efficiency, and low life cycle cost [3].
The behaviour of reinforced or restored square columns was explored. Majid Matouq Assas [18] tested twenty-seven genuine concrete column models experimentally. This experimental investigation took four variables into account: the surface treatment of the original column, the grade of the filled concrete, the kind of steel jacket, and the thickness of the corrugated steel jacket. The ultimate load grew as the thickness of the corrugated steel jacket increased, and the rate of growth increased as well. As strengthening or repairing square columns, utilizing a corrugated steel jacket enhances the final capacity by about 46% when compared to using a flat steel jacket.
-
Fiber reinforced polymer
In 1975, fiber reinforced polymer (FRP) was first used as "reinforcement bars" in Russia. FRP is also known as "fiber- reinforced plastic," and it refers to materials that use either synthetic or natural fibers to automatically increase the stiffness and strength of a polymer model. FRPs used to strengthen and reinforce structures are 8 times as strong as traditional steel reinforcement bars [19].
Polymer composites are multi-phase materials created by mixing polymer matrix, fillers, and reinforcing fibers to create a bulk material with qualities superior to the individual basic components. The matrix might be thermoplastic (polypropylene, polyethylene, polystyrene, PVC (polyvinyl chloride), etc.) or thermosetting (polyvinyl chloride, for example) (polyester, vinyl ester, epoxy resins, etc.). Fillers are frequently used to add bulk to a material, reduce cost, reduce bulk density, or provide aesthetic aspects. Fibers are added to polymers to strengthen them and increase mechanical qualities such as stiffness and strength [20].
FRPs are seen as a viable alternative to steel reinforcement, particularly in concrete buildings subjected to a harsh environment or the impacts of electromagnetic fields. FRP reinforcement is made from a variety of materials, including carbon fiber (which has the finest mechanical qualities among all FRP composites), basalt fiber, aramid fiber, and glass fiber [21,22].
-
Carbon fiber reinforced polymer (CFRP)
CFRP has advantages such as light weight, corrosion resistance, high strength with a long fatigue life, and the ability to be installed with a small crew and standard equipment. Carbon fiber has a two-dimensional atomic structure that results in distinct characteristics longitudinally and transversely. The modulus and strength of the fiber are strong along the longitudinal axis, in contrast to the transverse axis, which gives minimal strength or modulus. When subjected to fatigue loading, these fibers show remarkable endurance in high temperature and moisture environments [6].
Mesbah, H. A., and R. Benzaid examine the behaviour of pre-damaged and undamaged reinforced concrete reinforced with CFRP composite sheets. The results revealed that repairing and reinforcing RC members using external CFRP composite sheets improved strength and deformability. As a result, there is a considerable rise in strain energy, indicating an increase in energy dissipation capacity [23].
-
Glass fiber reinforced polymers (GFRP)
Because GFRP bars are less expensive than other forms of FRP material, they are becoming increasingly popular in the building sector. Furthermore, the cost of GFRP bars has decreased in recent years, owing mostly to a broader market and increased competition. GFRP bars have been employed brilliantly as primary reinforcement in concrete bridges, parking garages, tunnels, and water tanks [20].
Depending on the strength augmentation desired, two procedures are commonly used to strengthen beams: flexural strengthening and shear strengthening. In many cases, it may be necessary to provide both strength gains. FRP sheets or plates are put on the stressed face of a beam to reinforce it. Glass fibers have been widely employed in the aerospace sector for over 50 years due to their extremely high strength-to-weight ratio. They are also extensively used in wind turbine blades and naval engineering [24].
-
Aramid fiber reinforced polymers (AFRP)
Aramid fibers are heat-resistant and strong synthetic fibers containing molecules branded by relatively rigid polymer chains. It possesses strong synthetic fibers, high strength and elastic modulus, heat resistance, 40% less density than GFRP, and a somewhat higher cost. AFRP absorbs moisture and remains sensitive throughout manufacture until soaked with a polymer matrix. Because of its strong resilience to alkaline conditions and lower cost than CFRP reinforcing bars, AFRP is a preferable choice. The breaking strength of AFRP at high loading rates is equivalent to and 40% greater than that of other FRP materials, resulting in just a 13% drop in strength after 100,000 cycles [19].
-
Basalt fiber reinforced polymers (BFRP)
A new category of FRP composites has recently evolved as a result of the availability of basalt fibers, which are produced by extrusion from molten basalt volcanic rock. Basalt fibers are non-toxic, natural, eco-friendly, and environmentally safe. Basalt
fibers outperform glass fibers in terms of material properties (such as tensile strength and elastic modulus) and are substantially less expensive than carbon fibers. As a result, basalt FRP (BFRP) composites are seen as feasible, long-term replacements for traditional FRP composites for future RC structural strengthening applications. However, the usefulness of BFRP composites as a strengthening material to improve the structural performance of weak RC structures has received limited attention in the literature [25].
As demonstrated in Figure 2, BFRP has superior mechanical, chemical, and cost-performance characteristics when compared to CFRP, GFRP, AFRP, steel bars, and other materials. For example, BFRP has greater strength and modulus, a similar cost, and greater chemical stability than E-glass FRP; a wider range of working temperatures and a much lower cost than carbon FRP (CFRP); and more than five times the strength and one-third the density of commonly used low-carbon steel bars [26].
Figure 2 Stress-strain relationship of different FRP [26].
BFRP, these fibers are resistant to heat, have a high tensile strength, and have a long lifespan. Other advantages include strong acid resistance, outstanding electro-magnetic characteristics, resistance to corrosion, resistance to radiation and UV light, and good vibration resistance [17, 27]. Basalt FRP bars are a great choice for bridge girder reinforcement because they reduce slab weight while providing high corrosion resistance, decreasing repairs, and significantly increasing use [28].
Basalt is a common rock in the Maharashtra Deccan Trap. It is a natural, hard, thick, dark, and brown to black volcanic igneous rock created by solidifying molten lava. Fibers can be created from it because it is accessible in solid rock, and because of sophisticated processes and developments in the building sector. Researchers began working on basalt rock, and the technology of manufacturing fibers by melting the basalt rock at high temperatures of 15001700o C to make the continuous basalt fiber was established. Straps, sheets, chopped fiber, mats, nets, and rods can also be made from the fibers [7, 28-30].
Thorhallson, et al. looked into "concrete column strengthening by wrapping basalt fiber matrix." They only cast 100- and 200-mm cylinders and tested them on BFRP-confined cylinders for tensile coupon test and compression test. Specimens were wrapped in single, double, and three layer wraps of basalt fiber. They concluded that confined cylinders exhibit good ductile behavior and have a higher load bearing capacity than unconfined specimens [31].
li>
A Hybrid Composite
The flexural characteristics of carbon-basalt (C/B) hybrid composites generated by evaluating several stacking sequences of CFRP and BFRP laminate resulted in a considerable influence of fiber arrangement on flexural strength, fatigue behavior, and impact performance. In all of the circumstances studied, hybridization leads to a 73% increase in strength over BFRP. The maximum flexural strength and modulus are obtained when the carbon layers are arranged on the compressive side of the laminate. Furthermore, when basalt layers were put in both tensile and compressive zones, the highest ductility performance was attained. In fact, while there was a drop in strength compared to FRP based only on carbon fibers (approximately 14%), there was a higher compliance than in the CFRP instance due to the regulated reduction in total stiffness due to the introduction of basalt fibers [32].
-
-
THE PARAMETERS AFFECTING PERFORMANCE OF COLUMNS WHICH STRENGTHENING BY FRP
The most parameters effect on the performance of confined columns using FRP:
-
Shape of column section
Sakol Suon et al. discovered that changing the cross-section shape from non-circular to circular or decreasing the cross- sectional aspect ratio from 2 to 1 practically linearly increased the load bearing capacity of BFRP-confined specimens. Although the normalized ultimate axial strain of BFRP-confined specimens was typically reduced when the cross-section was changed from circular to non-circular, a clear association could not be seen from the available data [33].
The effect of strengthening is better in circular columns than in non-circular columns, where the non-circular geometry of the cross sections creates non-uniform stresses distributions, which reduces the confinement effect on column behavior [34]. For square and rectangular columns, corner radius is a significant variable affecting FRP confinement. As the corner radius increases, the effective confinement area of the core concrete also increases, and FRP provides more uniform confining pressure, which improves the column's strength [34- 38]. Sakol et al. investigate the influence of corner radius on the behavior of RC columns strengthened with BFRP in various cross-sectional shapes. Increasing the corner radius or the number of BFRP layers enhanced the maximum condition of reinforced concrete [33]. Saravanan, et al. studied the behavior of the RC columns by adopting hybrid FRP rovings. The column's cross-section measures up to 150 x 380 mm, and the shorter face radius of 75 mm is cast. The results showed that compressive strength increased by up to 25% more than the control column [39].
-
Fiber orientations
The influence of CFRP jacket thickness and different eccentricities was explored in research done by Waryosh, W.A., Rasheed, M.M., and Al-Musawi, A.H. The test program included twelve specimens with square cross sections (120 mm × 120 mm) that were manufactured and tested under eccentric compressive stress up to failure. The specimens with two hunched heads had a total length of 1230 mm. There were two fiber orientations (0°, 90°) with respect to an axis perpendicular to the column axis. The results showed that reinforced specimens outperformed un-strengthened specimens. It can also be noted that there is a minimal difference between specimens strengthened by two layers (0°, 90°) and those strengthened by a single layer (90°). It can be observed that FRP reinforced by two layers (0°, 90°) gives a greater reaction to compassion than FRP reinforced by one layer (90°) alone [17].
Saravanan S. and colleagues investigated the strength of columns using hybrid FRP roving. Four chosen wrapping patterns are wrapped around the column of different specimens of the same size for the study, and the strength produced from the wrapping pattern is compared to that of the unconfined column in figure 6. The cross section of the column, measuring up to 150x380mm, and the shorter face radius of 75mm are cast. Glass, basalt, and jute fibers are employed in the experimental stages. After detailed investigation, the specimen CBGJ wrapped with Basalt, Glass, and Jute fiber had higher axial compressive strength than the other specimens chosen for study, and the CBGJ wrapping pattern had 25% higher compressive strength than the unconfined column [39].
-
Strength concrete
Ahmed Shaban Abdel-Hay evaluated the overall behavior of R.C. square columns with inadequate concrete at the top section and CFRP reinforcement. Only the top of the column was wrapped. An experimental program was carried out on ten square columns measuring 200 mm by 200 mm by 2000 mm. One was a control specimen, while the other nine were CFRP- enhanced specimens. The compressive strength of the upper section, the height of the upper reinforced concrete part, and the height of the CFRP-wrapped part of the column were the major parameters investigated in this study. He discovered that partial strengthening of square columns with CFRP is allowable and results in satisfactory column carrying capacity [40].
Vincent and Ozbakkaloglu [37] employed three different concrete strengths to determine the effect of concrete strength on the behavior of FRP-concrete columns under axial compression. They axially loaded 55 cylindrical specimens of 152 mm diameter and 305 mm height. CFRP tube-encased concrete and CFRP-wrapped concrete were employed. The study indicated that proper FRP confinement improves the ductility of high- and ultra-high-strength concrete specimens.
-
Magnitude of eccentricity
Hadi tested the impact of eccentrically loaded configurations on the behavior of FRP-concrete columns using two distinct eccentrically loaded configurations, one under 25 mm eccentric load and one under 50 mm eccentric load. Three of the columns were strengthened with steel bars, while the remaining six were constructed entirely of plain concrete. Three of the six straight
columns were wrapped in unidirectional carbon, while the remaining three were covered with woven E-glass. The study found that eccentric loading might significantly reduce the maximum failure load of FRP-confined concrete columns as compared to concentric loading columns. However, when tested concentrically and eccentrically, FRP-confined columns demonstrated greater load capacity and ductility than internally strengthened concrete columns [41].
According to the experimental study [42], all damages in eccentrically loaded GFRP-RCCs exhibited crushing of the concrete in the compression zone, regardless of the eccentric loads. GFRP bars can improve ductility in large-eccentricity specimens, but this impact is minimal or nonexistent in small-eccentricity specimens. The ductility of the GFRP-reinforced concrete columns increased with increasing eccentricity in the descending region of the load-deformation curve.
-
Type of FRP materials
The most noteworthy conclusion of the study is that GFRP-RC columns and beams are more ductile than their steel- reinforced equivalents because they absorb more energy through their ability to bend well in the post-peak collapse area. It was also demonstrated that the strain ductility of GFRP-reinforced members is significantly greater than that of steel RC columns [26]. The replacement of steel reinforcement with similar amounts of GFRP rebars and ligatures results in a reduction in total axial capacity. Increased load eccentricity reduces axial capacity even further [27, 42].
Jianwei Tu, et al., as well as Hany Tobbi, et al., investigated the effects of longitudinal reinforcement ratio, stirrup configuration (spirals versus hoops), and spacing on the load-carrying capacity and failure modes of GFRP-RC columns. They discovered that the load-carrying capacity of longitudinal GFRP bars accounted for 3%7% of the ultimate load-carrying capacity of the columns. Reduced stirrup spacing may prevent longitudinal bar buckling and increase the ductility and load-carryng capacity of GFRP-RC columns. Setting the GFRP compressive strength to 35% of the GFRP maximum tensile strength yields a reasonable estimate of the ultimate load-carrying capacity of GFRP-RC columns [43, 44].
Thong M. Pham et al. examined the structural performance and failure mechanisms of FRP-constrained concrete covered with various FRP configurations. It has been discovered that partly wrapped specimens have a lower compressive strength but a larger strain than fully wrapped specimens. Non-uniformly wrapped specimens, on the other hand, had greater compressive strength and axial strain than completely wrapped specimens. The actual rupture strain of the FRP jackets varies depending on the wrapping configuration. The strain efficiency factor in the whole wrapping arrangement is larger than that in the partial wrapping configuration but less than that in the non-uniform wrapping arrangement [45].
G. Campione et al. wanted to see how well basalt fiber wraps worked when applied externally to cylindrical compressed concrete specimens to boost the material's strength and ductility. In compression, 26 cylinders were tested: 4 control cylinders without confinement, 14 cylinders wrapped with BFRP, and 8 cylinders wrapped with CFRP. The test findings also reveal that specimens confined with BFRP display strain-softening behavior, with small increases in resistance but a large rise in ultimate strain (up to 5 times the peak strain of unconfined concrete), which correlates to FRP failure and concrete crushing. Specimens contained in CFRP display strain hardening behavior with a considerable improvement in strength and ductility, the latter of which is equal to that of basalt fibers [46].
ACI 440.2R [47] specifies an NSM system as round or rectangular bars or plates inserted and cemented into concrete surface grooves. A suitable adhesive should be used to bind the FRP bar into the groove so it can be cured in place. The glue allows shear transmission between the concrete substrate and the NSM FRP. ACI 440.2R evaluates FRPs exclusively under tension and disregards any contribution of FRP bars or strips in concrete under direct compression.
While FRP materials can withstand compressive loads, the use of FRP for compression raises a number of concerns, according to ACI 440.2R. Micro-buckling of fibers can occur if the laminate has any resin vacancies. If laminates are not correctly attached or secured to the substrate, they might buckle, and misaligned fibers in the field can result in very unreliable compressive strengths. It is permissible, however, for FRP tension reinforcement to compress owing to moment reversals or changes in load pattern. On the other hand, the compressive strength of the FRP reinforcement should not be ignored. Based on the same logic, ACI 440.1R [48] disregards the compressive contribution of interior FRP bars.
On the other hand, multiple experimental experiments have shown that internal FRP bars may withstand large compressive strain if enough lateral support is supplied. Tobbi et al. [45] investigated large-scale columns and discovered that glass fiber reinforced plastic (GFRP) bars could be used in compression members if appropriate transverse bars were provided to prevent bar buckling. Fillmore and Sadeghian [49] discovered that the elastic modulus of GFRP bars in compression is somewhat greater than that in tension; nonetheless, compressive strength was reached at 67% of tensile strength. Furthermore, Khorramian and Sadeghian [50] demonstrated that GFRP bars may be viewed as load-bearing longitudinal reinforcement of concrete columns and that disregarding their influence is not essential.
NSM FRPs are also beneficial for concrete columns that are significantly bent, and their efficacy is increased by using FRP wraps. A total of 21 plain concrete cylinders (150 mm x 300 mm) were produced, reinforced, and tested. Multiple GFRP bars (#4) were installed in 20 mm by 20 mm surface grooves, and unidirectional basalt FRP (BFRP) was employed to wrap the
specimens. The results demonstrate that concrete specimens containing NSM bars did not exhibit any evidence of crushing until the concrete bulged and broke extensively. The FRP wrap dramatically altered the behavior of the NSM specimens, raising both peak load and its associated strain by avoiding buckling of NSM bars and prolonging the contribution of NSM bars. The hybrid system of longitudinal NSM GFRP bars and lateral BFRP wrapping improved the performance of concrete specimens [51].
-
Thickness of fiber
S. Karthikraja et al. want to know the strength and deflection impact of external wrapping of glass fiber in a short column. In comparison to the reference column, the GFRP columns enhanced ultimate stress and ultimate axial strain. When four layers of completely wrapped columns were compared to a reference column without wrapping, the maximum ultimate axial stress rose by 6%. Full wrapping with four layers of GFRP gives greater confinement to the concrete column. Vertical strip wrapping with four layers provides greater containment than complete wrapping with four layers [52].
Akshay P. Mote and H. S. Jadhav explore the axial loading behavior of R C short columns enhanced with BFRP wrap. In order to strengthen RC columns, fourteen columns were cast and tested to failure under axial force. The columns were bonded using BFRP sheets in single- and double layer arrangements. According to the testing results, the ultimate load of columns with BFRP wrapping in various configurations and layers is greater than that of columns without BFRP wrapping. Ultimate load increases are 57%, 69%, 17%, 36%, 13%, and 26% for single-layer full wrap columns, double-layer full wrap columns, single- layer horizontal strip columns, double-layer horizontal strip column wrap columns, and single-layer vertical strip column wrap columns, respectively. The increases in Ductility Index for columns single layer full wrap, double layer full wrap, single layer horizontal strip, double layer horizontal strip wrap, single layer vertical strip, and double layer vertical strip wrap are 49%, 100%, 35%, 65%, 47%, and 63%, respectively [53].
BFRP layers are one of the important elements that determine the behavior of concrete columns. The increase in ultimate load for columns of single layer full wrap, double layer full wrap, and single layer partially wrapped, double layer partially wrapped is 11.90%, 23.71%, 9.75%, and 14.89% [54].
-
Radius of corners
Modifying the geometry of square-to-circular and rectangular-to-elliptical columns eliminates corner stress concentration in prisms and improves confinement efficacy. Following FRP-wrapping of shape-modified columns, axial load and pseudo- ductility will be significantly increased [3].
ArathiKrishna et al. investigate the load-bearing capability of BFRP-restricted concrete columns. Specimen failure might be classified into three categories. The first is the compression failure mode. This is a brittle failure condition due to compression failure. This sort of breakdown is most common in standard reinforced-concrete components. The second kind of failure is laminate rupture, which may be seen in certain specimens as a rupture of BFRP laminates with abrupt failure. The third mechanism of failure is ductile failure, which happens when BFRP laminates rupture. These kinds of failures are caused mostly by issues with the corner radius. To avoid such failure, give the columns a suitable corner radius, which is rounded corners of more than 20 mm [54].
Experiments [33] revealed that increasing the corner radius boosted the efficiency of BFRP confinement in square and rectangular specimens. The ultimate strength increased approximately linearly as the corner radius increased, whereas the ultimate strain decreased. The ultimate strains for circular specimens, which are a special case of square specimens with 75 mm corner radius, did not follow the declining trend and had larger ultimate axial strains than the other square specimens with 0, 13, or 26 mm corner radius.
CONCLUSIONS
The behavior of FRC under static loading conditions were comprehensively reviewed. The following conclusions of the literature review are made:
-
FRP strengthening increases the fatigue life of RC columns by improving absorbed energy and strain and reducing crack propagation.
-
Strengthening refers to efforts made to improve an existing structure's load bearing capacity and/or impact resistance in order for it to meet the required performance level.
-
The degree of improvement in the axial strength and strain capacities of concrete columns due to confinement with FRP materials is dependent on many parameters, such as column slenderness ratio, cross-section shape, and concrete strength, the method used to manufacture the tube, fiber properties, fiber orientation, and FRP thickness.
-
From the observed literatures, it is clear that increase in the number of layers of BFRP wrap improves the maximum load.
-
The cross-section ratio, on the other hand, has a significant influence on confinement. The BFRP performs better in square sections than in rectangular sections, using the same strengthening technique.
-
The behavior of the specimens is significantly improved in both cases of fully and partially strengthened columns when compared to the control columns.
-
there is a sharp decline in the axial deflection value as long as we increase the value of eccentricity. By contrast, there is a significant increase in the value of lateral deflection while we increase the value of eccentricity.
REFERENCES
[1] Daugeviius, M., Valivonis, J., Beinaraviius, A., Skuturna, T., & Budvytis, M. (2013). Experimental investigation of the load carrying capacity of eccentrically loaded reinforced concrete elements strengthened with CFRP. Procedia Engineering, 57, 232-237. [2] El-Kholy, A. M., & Dahish, H. A. (2016). Improved confinement of reinforced concrete columns. Ain Shams Engineering Journal, 7(2), 717-728. [3] Parvin, A., & Brighton, D. (2014). FRP composites strengthening of concrete columns under various loading conditions. Polymers, 6(4), 1040-1056. [4] Ouyang, L. J., Gao, W. Y., Zhen, B., & Lu, Z. D. (2017). Seismic retrofit of square reinforced concrete columns using basalt and carbon fiber-reinforcedpolymer sheets: A comparative study. Composite Structures, 162, 294-307.
[5] Jain, P., Bansal, B., K., and Sharma, A. (2019). Efficiency of Strengthening RC Column with Different Slenderness Ratio using Basalt Fabric.International Journal of Engineering Science and Computing, 9, 19575 19578.
[6] Tejal, S., Pallavi, K., Arvind, K., Akshata, B., & BG, B. (2020). Study of Confinement of a Column by Carbon Fibre Reinforcement Polymer Mesh. [7] Wang, H. L., & Zhong, Y. H. (2014). Research status and proposals of basalt fiber reinforced concrete. In Advanced Materials Research (Vol. 834,pp. 730-737). Trans Tech Publications Ltd.
[8] Widiarsa, I. B. R., & Hadi, M. N. (2013). Performance of CFRP wrapped square reinforced concrete columns subjected to eccentric loading. Procedia Engineering, 54, 365-376. [9] Karim, H., Sheikh, M. N., & Hadi, M. N. (2016). Axial load-axial deformation behaviour of circular concrete columns reinforced with GFRP bars and helices. Construction and Building Materials, 112, 1147-1157. [10] ACI Committee 546, "Concrete Repair Guide", ACI 546R-96, American Concrete Institute, 2001. [11] Naser, M. Z., Hawileh, R. A., & Abdalla, J. A. (2019). Fiber-reinforced polymer composites in strengthening reinforced concrete structures: A critical review. Engineering Structures, 198, 109542. [12] Petra, S., Ivanab, L., Petrc,S. and paveld, S. (2017). The Strengthening of Reinforced Concrete Structures" Key Engineering Materials. V. 738, 239- 248. [13] Ahmed, S.K. (2018). Strengthening Reinforced Concrete Columns by Fiber Reinforced polymer (FPR) A General Review. Journal of Engineering and Applied Sciences, 13, 9582-9596. [14] Mosheer, K. A. M. (2016). Strengthening and Rehabilitation of Reinforcement Concrete Square Columns Confined with External Steel Collars. Kufa Journal of Engineering, 7(1). [15] Wu, Y. F., & Wei, Y. Y. (2010). Effect of cross-sectional aspect ratio on the strength of CFRP-confined rectangular concrete columns. Engineering Structures, 32(1), 32-45. [16] Aktas, G., & Gunaslan, S. E. (2017). Strengthening methods for reinforced concrete sections with Fiber reinforced polymers. IOSR J. Mech. Civ.Eng, 14, 17-22.
[17] Waryosh, W.A., Rasheed, M.M. and AL-musawi, A.H. (2012). Experimental Study of Reinforced concrete Columns Strengthened with CFRP under Eccentric Loading ", Journal of Engineering and Development, 16, 421-440. [18] Assas, M. M. (2014). Efficiency of corrugated steel jackets for strengthening or repairing square section concrete columns. Life Science Journal, 11(8s). [19] Amran, Y. M., Alyousef, R., Rashid, R. S., Alabduljabbar, H., & Hung, C. C. (2018, November). Properties and applications of FRP in strengtheningRC structures: A review. In Structures (Vol. 16, pp. 208-238). Elsevier.
[20] Das, S. C., & Nizam, M. (2014). Applications of fiber reinforced polymer composites (FRP) in civil engineering. International Journal of Advanced Structures and Geotechnical Engineering, 3(3), 299-309. [21] Sangeetha, P., & Sumathi, R. (2010). Behaviour of glass fibre wrapped concrete columns under uniaxial compression. International Journal of Advanced Engineering Technology, 1(1), 74-83. [22] Gudonis, E., Timinskas, E., Gribniak, V., Kaklauskas, G., Arnautov, A. K., & Tamulnas, V. (2013). FRP reinforcement for concrete structures: state- of-the-art review of application and design. Engineering Structures and Technologies, 5(4), 147-158. [23] Mesbah, H. A., & Benzaid, R. (2017). Damage-based stress-strain model of RC cylinders wrapped with CFRP composites. Advances in concrete construction, 5(5), 539. [24] Dasgupta, A. (2018). Retrofitting of concrete structure with fiber reinforced polymer. International Journal, 4(9), 42-49. [25] Li, J. J., & Liu, Y. C. (2013). On Research of Basalt Fiber Used in Asphalt Concrete. In Advanced Materials Research (Vol. 791, pp. 456-459). Trans Tech Publications Ltd. [26] Zhishen, W., Xin, W., & Gang, W. (2012). Advancement of structural safety and sustainability with basalt fiber reinforced polymers. CICE2012, Rome, 13, 15-29. [27] Afifi, M. Z., Mohamed, H. M., & Benmokrane, B. (2014). Axial capacity of circular concrete columns reinforced with GFRP bars and spirals. Journal of Composites for Construction, 18(1), 04013017. [28] Urbanski, M., Lapko, A., & Garbacz, A. (2013). Investigation on concrete beams reinforced with basalt rebars as an effective alternative of conventional R/C structures. Procedia Engineering, 57, 1183-1191. [29] Jaysing, G. P., and Joshi, D.A. (2013). Review on Application of Basalt Fiber in Civil Engineering, II, 54-58. [30] Li, Z., Ma, J., Ma, H., & Xu, X. (2018, September). Properties and applications of basalt fiber and its composites. In IOP Conference Series: Earth and Environmental Science (Vol. 186, No. 2, p. 012052). IOP Publishing. [31] Thorhallsson, E. R., Konradsson, A., & Kubens, S. (2011). Strengthening of concrete columns by wrapping basalt fiber matrix. In Nordic Concrete Research Symposium (pp. 457-462). [32] Monaldo, E., Nerilli, F., & Vairo, G. (2019). Basalt-based fiber-reinforced materials and structural applications in civil engineering. Composite Structures, 214, 246-263. [33] Suon, S., Saleem, S., & Pimanmas, A. (2019). Compressive behavior of basalt FRP-confined circular and non-circular concrete specimens. Construction and Building Materials, 195, 85-103. [34] Gao, P., un, D., Zhao, Y., Hong, L., Wang, Z., & Chen, T. (2021). Effect of the corner radius on the axial compressive performance of large steel- reinforced concrete columns confined by carbon fibre-reinforced polymer. Engineering Structures, 239, 112303. [35] Berthet, J. F., Ferrier, E., & Hamelin, P. (2005). Compressive behavior of concrete externally confined by composite jackets. Part A: experimental study. Construction and building materials, 19(3), 223-232. [36] Raval, R., & Dave, U. (2013). Behavior of GFRP wrapped RC Columns of different shapes. Procedia Engineering, 51, 240-249. [37] Mostofinejad, D., Moshiri, N., & Mortazavi, N. (2015). Effect of corner radius and aspect ratio on compressive behavior of rectangular concrete columns confined with CFRP. Materials and Structures, 48(1), 107-122. [38] Yeh, F. Y., & Chang, K. C. (2004, August). Size and shape effect on FRP confinements for rectangular concrete columns. In 13th World Conference on Earthquake Engineering, Vancouver, BC, Canada, paper (No. 657). [39] Saravanan, S., Sakthieswaran N and Shiny, B.G. (2015). A Study on Hybrid FRP Wrapped Axially Loaded RC Capsule Column" International Journal of Research in Advent Technology, 3, 94-98. [40] Abdel-Hay, A. S. (2014). Partial strengthening of RC square columns using CFRP. HBRC Journal, 10(3), 279-286. [41] Sun, L., Wei, M., & Zhang, N. (2017). Experimental study on the behavior of GFRP reinforced concrete columns under eccentric axial load. Construction and Building Materials, 152, 214-225. [42] Elchalakani, M., Ma, G., Aslani, F., & Duan, W. (2017). Design of GFRP-reinforced rectangular concrete columns under eccentric axial loading. Magazine of Concrete Research, 69(17), 865-877. [43] Tu, J., Gao, K., He, L., & Li, X. (2019). Experimental study on the axial compression performance of GFRP-reinforced concrete square columns. Advances in Structural Engineering, 22(7), 1554-1565. [44] Tobbi, H., Farghaly, A. S., & Benmokrane, B. (2012). Concrete Columns Reinforced Longitudinally and Transversally with Glass Fiber-Reinforced Polymer Bars. ACI Structural Journal, 109(4). [45] Pham, T. M., Youssed, J., Hadi, M. N., & Tran, T. M. (2016). Effect of different FRP wrapping arrangements on the confinement mechanism. Procedia Engineering, 142, 307-313. [46] Campione, G., La Mendola, L., Monaco, A., Valenza, A., & Fiore, V. (2015). Behavior in compression of concrete cylinders externally wrapped with basalt fibers. Composites Part B: Engineering, 69, 576-586. [47] ACI (American Concrete Institute). (2017). Guide for the Design and Construction of Externally Bonded FRP Systems for Strengthening Concrete Structures. ACI 440.2R-17., ACI. Farmington Hills, Michigan, USA. [48] ACI (American Concrete Institute). (2015) Guide for the Design and Construction of Structural Concrete Reinforced with Fiber-Reinforced Polymer (FRP) Bars, ACI 440.1R-15. ACI. Farmington Hills, Michigan, USA [49] Fillmore, B., & Sadeghian, P. (2018). Contribution of longitudinal glass fiber-reinforced polymer bars in concrete cylinders under axial compression. Canadian Journal of Civil Engineering, 45(6), 458-468. [50] Khorramian, K., & Sadeghian, P. (2017). Experimental and analytical behavior of short concrete columns reinforced with GFRP bars under eccentric loading. Engineering structures, 151, 761-773. [51] Sadeghian, P., & Fillmore, B. (2018, April). A hybrid composite system for strengthening concrete columns. In Structures Congress 2018. American Society of Civil Engineers. [52] Karthikraja, S., Sasthika, P., Nandakumaar, A., Santhanakumar, M., & Deventhiran, K. (2016). Deformation characteristics of glass fiber reinforced polymer wrapped axially loaded short circular HSC column. Indian Journal of Science and Technology, 9(16), 1-5. [53] Mote, A. P., & Jadhav, H. S. (2014). Experimental Study of Axially Loaded RC Short Columns Strengthened With Basalt Fiber Reinforced Polymer (BFRP) Sheets. International Journal of Engineering Research and Applications, 4(7), 89-92.. [54] ArathiKrishna, Jacob, M., and Mohan, K.R. (2018). Experimental Study on Strengthening of RC Short Columns with BFRP Sheets. International Journal of Engineering &Technology. 7, 48-52.