
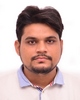
- Open Access
- Authors : Usman Khan
- Paper ID : IJERTV13IS120023
- Volume & Issue : Volume 13, Issue 12 (December 2024)
- Published (First Online): 04-01-2025
- ISSN (Online) : 2278-0181
- Publisher Name : IJERT
- License:
This work is licensed under a Creative Commons Attribution 4.0 International License
A Review on Infra Red Heating Application in Food Processing Industries
Usman Khan
Department Of Post Harvest Engineering and Technology Aligarh Muslim University Aligarh, Uttar Pradesh, India.
Abstracts: Compared to conventional heating, Infrared (IR) mechanization is highly energy-efficient, less consuming of water, and environment friendly. Infrared is also described by the uniformity of heating, high rate of heat transfer, low heating time, low energy dissipation, enhanced product quality, and food safety. Infrared mechanization is used in various food manufacturing processes for example Drying, boiling, heating, peeling, polyphenol recovery, freeze-drying, antioxidant recovery, microbiological inhibition, sterilization grains, bread, food roasting, manufacture of juices, and cooking food. This article reviews aspects of IR heating and presents a theoretical basis for IR heat processing of food materials and the interaction of IR radiation with food components. The effect of Infra red on food quality attributes is discussed in the context of samples and process parameters. Applications of IR heating in food processing operations and future research potential are also reviewed.
Keywords : Infra red (IR) heating, NIR (Near Infra red), MIR(Mid Infra red), FIR(Far Infra red), Infra red emitters, Wave guides.
-
INTRODUCTION
In food processing, heating is one of the most important thermal processes, and it is conducted by conduction, convection, and radiation (Saravacos et al., 2016). Heating is traditionally done with either a fuel source or an electrical source. Heat is transferred from the outside to the surface of the food by convection and conduction and the temperature on the surface of the food increases further as the heat is transferred to the food by conduction. The temperature of a heated substance starts to change physically and chemically as it increases in temperature (Lee et al., 2006).
The infrared region of the electromagnetic spectrum, which lies between visible light and microwaves, has wavelengths between 0.5 and 100 µm. Infrared beams are isolated into three kinds: Near-IR (NIR) whose frequency goes from 0.75 to 1.4 µm at temperatures under 400°C, and mid-IR (MIR) whose frequency is somewhere in the range of 1.4 and 3 µm at temperatures somewhere in the range of 400 and 1000°C and far-IR radiation (FIR) frequency of 3-1000 µm at temperatures over 1000°C (Rosenthal, 1992). As a result of infrared penetration, water molecules vibrate at a frequency of 60,000-150,000 MHz, and heat is generated.
When radiant energy dissipates as heat, the surface of the food is heated, and the penetration depth depends on various factors, including thickness, water activity, product components, and the wavelength of the infrared radiation (Sakai et al., 1994). Infrared technology has a number of benefits, including high energy efficiency, low water consumption, and environmental friendliness, according to
Pan and Atungulu (2010). In addition, it is also characterized by the homogeneity of heating with a high heat transfer rate, low heating time, low energy consumption, and improving product quality and food safety. It also features low energy costs and the size of infrared equipment is small as well as controlling factors with high accuracy (Nowak & Lewicki, 2004). Nindo and Mwithiga (2010) stated that infrared radiation does not pollute the environment compared to fossil fuels. In addition, it preserves vitamins, has very little flavor loss, and has no heating of the surrounding air. The Food and Drug Administration (FDA) has indicated that infrared radiation can be used in food processing, which is not ionizing.
-
INFRARED (IR) HEATING
-
Principle: Among the three heat transfer principles mentioned above (conduction, convection, and radiation), radiation is the main principle of IR heating. In Fig. 1(a), IR is an electromagnetic radiation whose wavelength ranges from 0.78 to 1000 µm; it lies between visible light and microwaves. There are three regions: near-IR radiation of wavelengths 0.78 to 1.4 µm, mid-IR radiation of 1.4 to 3.0 µm, and far-IR radiation of wavelengths 3.0 to 1000 µm. Some of the IR rays incident on a target surface of an object penetrate the objects body and are reflected. The rays penetrated by the objects body are further separated into two groups, shown in Fig. 1(b). Only absorbed rays increase the temperature of an object, as they activate molecules in the air. The ratio of absorbed to incident rays is known as absorptivity. Higher absorptivity usually translates into higher efficiency. In mechanical and electrical industries, almost all of the penetrated rays are absorbed by solid materials, which are less sensitive to IR wavelengths. The absorptivity of food is, however, strongly influenced by moisture content, as foods are porous and moist (Lampinen et al., 1991). MIR radiation is the second most well absorbed radiation type when compared to NIR radiation because FIR is highly absorbed by most food materials (Siegel and Howell, 1992). Taking the potato for example, with a 51% moisture content, the potato tissue absorbs almost 90% of MIR (1.52 µm), and its absorptivity increases as wavelength increases. However, in the NIR range, the potato tissue absorbs only one fourth as much NIR as it does MIR (1.52 µm) (Datta et al., 2005). Similarly, Sandu (1986) examined the relationship between absorption of wavelength and food components. Fig 2 compares the wavelength absorption of liquid water with the wavelength absorption of major food components (Sandu, 1986)
Fig. 1: (a) Wavelength range of IR radiation; (b) Classification of IR radiation after reaching the target material (Lee et al., 2010)
Fig. 2: Relation between absorption of wavelength and main food components compared to the infrared absorption of liquid water (Sandu, 1986)
-
Infrared Emitters for food processing
IR emitters can be broadly divided into three categories based on wavelength: short-wave, medium-wave, and long-wave emitters. As the short-wave emitters operate at very high emissive power and temperature, they may not be suitable for prolonged thermal processes of food materials due to the possibility of overheating. Medium-wave IR emits radiation spectrum ranging from 1.4 to 3.0 µm and could be used for
drying and curing of food products. Emitters generating long- wave IR are useful in low-temperature processes demanding a combination of convection and IR radiation heating. The thermal efficiency, heat transfer rate, and effect of radiation on heated materials are closely related to the range of wavelengths emitted from the heating source (Siegel and Howell, 1981). IR emitters can also be classified into electrical or gaseous type. Electrical IR emitters have a filament heated to a particular temperature. The electrical emitters made of ceramic or quartz can be manufactured with standardized energy values, such as 60, 100, 150, 200, and 500 W. A number of such emitters can be mounted on a panel to make a module, with the spacing between each emitter being selected to give the desired radiant intensity. In general, electric heaters are expensive and are convenient to manipulate the power distribution of a given system according to the design requirement. The types of electric IR emitters include reflector type emitters, incandescent lamps, quartz tubes, and resistance elements such as metallic tubes, ceramic tubes, and non-metallic rods.
Electrical IR emitters normally have a conversion efficiency from 78% o 85%, whereas gaseous IR emitters have the conversion efficiency of 4046% (Ramaswamy and Marcotte, 2005). Electrical emitters can reach a radiant intensity of up to 400 kW/m², while gas-fired emitters generally do not exceed the radiant intensity of 22 kW/m² (Nindo and Mwithiga, 2010).
Gaseous IR emitters powered by propane or natural gas are commonly used in industrial applications, which demonstrate lower operating cost and higher reliability and durability compared to electrical IR emitters. There are two types of gaseous IR emitters: direct or open flame IR emitters and catalytic flameless IR emitters. The open flame IR emitters release IR radiation via normal flame combustion. During the combustion process, a portion of the fire flame may emit energy in the form of visible light, causing the wastage of energy and fuel (MacConnell, 1972). Incomplete combustion may result in smoke and soot built-up on emitters or release very small particles of carbon into the environment, which
should be minimized. Hence, direct flame IR emitters have restricted usage in food processes because of the safety and environmental concerns.
The catalytic IR emitter emits radiation heat over a wide range of wavelengths without any visible flame. Typically, these emitters have radiant intensity varying from 6 to 28 kW/ m², emitting MIR and FIR radiation with the heating-up period varying between 180 and 300 s and the radiation efficiency ranging from 30% to 75% (Das and Das, 2010). The gaseous IR emitters are available in several sizes for industrial application. For example, a catalytic IR emitter with an effective heating area of 30 cm by 30 cm can generate IR heat with an isothermal surface temperature of the emitter at 500, which corresponds to a peak wavelength of 3.7 µm by assuming the emitter as a blackbody (Li et al., 2014c).
Table 1: Penetration depth of near-infrared energy into food products (Adapted from: Ginzberg, 1969)
Product
Spectral peak (µm)
Depth of penetration (mm)
Dough, Wheat
1.00
4-6
Bread, Wheat
1.00
11-12
Biscuit
1.00
4
Biscuit
0.88
12
Grain, Wheat
1.00
2
Carrot
1.00
1.5
Tomato paste
1.00
1
Potatoes
1.00
6
Potatoes
0.88
15-18
Apples raw
1.16
4.1
Apples raw
1.65
5.9
Apples raw
2.36
7.4
radiation (Gordon et al., 2002; Kandilli and Ulgen, 2009; Quaschning and Muriel, 2002). Normally, elliptical heaters are used for focused heating, such as soldering or for hot forming steel, while parabolic heaters are used for cooking and drying paint. The following two IR guides were built for a variety of purposes in addition to those listed above. Jun and Irudayaraj (2003) developed a waveguide to focus IR rays emitted from several IR heat sources into a narrow area, as shown in Fig. 4. Wave guide systems reduce the power used for one lamp to produce the same total power, and they use an optical filter to selectively heat selected wavelengths. This wave guide system with an optical filter is capable of providing an efficient heating process. Yang et al., (2014) developed an infrared guide system for delivering IR rays through curved paths using optical fibers. If this waveguide is employed along with IR heating, then the utility of IR heating can be even greater.
The efficiency of gaseous IR emitters depends on the size of the heater and the separation distance between the heater and the object being heated (Lia et al., 2005; Nindo and Mwithiga, 2010). Detailed descriptions of emitter types, designs, selections, and characteristics are reviewed by Das and Das (2010).
-
Design of Wave guides
IR lamps, which emit IR energy in all directions, dont provide a reliable heat source since the rays are not emitted to the back side of the appliance. For an effective heating process, IR rays from the lamp should be focused on the target surface. There are a variety of heating types that use the IR lamp. Elliptical and parabolic heaters are commonly used. An elliptical heater typically uses the IR lamp as a focal point. An elliptical reflector gathers wave energy from one focal point to the other, as shown in Fig. 3A. This principle generates a narrow area of collectivity with the exception of the direct radiation. For example, in order to destroy kidney stones, in the medical field, a condensed acoustic pulse is gathered by elliptical reflectors (Andriyanov and Bondarenko, 1986), and elliptical reflectors also have been used to guide light in optical applications (Hou et al., 2013). A parabolic reflector has an IR lamp located on the focal point of the parabolic reflector to heat a wider area since the parabolic reflector collimates the reflected IR rays, as shown in Fig. 3B. This collimating characteristic of the parabolic reflector is also defined by mathematics. Parabolic reflector is also have been commonly employed in antenna systems (Culter, 1947). Sunlight reflecting systems have also employed parabolic rIeJfEleRcTtoVrs13inISo1r2d0e0r2t3o efficiently use solar
Fig. 3: Reflectors to improve the heating efficiency (A) Elliptical reflector (B) Parabolic reflector .
Fig. 4: Waveguide to focus IR rays emitted from several IR heat sources into a narrow (Jun and Irudayaraj, 2003) .
-
Effect of Infrared on food molecular constituents
When IR radiation is impinged on a food surface, it is absorbed by organic materials at discrete frequencies that correspond to intramolecular transitions between energy levels depending on the chemical bonds present. Energy absorption mechanisms occur at wavelengths that correspond to the incident radiation energy. In the MIR and FIR regions, the wavelength range is 2.5-100 µm (Rosenthal, 1992; Sakai and Hanzawa, 1994). The most efficient way foods absorb MIR and FIR energy is through stretching modes of vibration, which causes them to radiate heat. In the agricultural food product processing industry, NIR radiation with high temperatures can cause discoloration and quality degradation as a result of non-specific control, so if NIR is used, the temperature ought to be carefully monitored. Typically, the FIR is characterized by low temperatures and low energy emission; if the temperature is too low, the energy emitted may not be sufficient for food processing. IR radiation can be used at temperatures between 150 and 2200°C, with peak wavelengths between 7 and 1.2 µm. A foodstuffs absorption properties are mostly determined by three factors: its water content, its thickness, and its physicochemical nature. Most foodstuffs exhibit high transmission at wavelengths of 2.5 µm (Sandu, 1986). Furthermore, studies have shown that foodstuffs exhibit abrupt increases in NIR transmissivity when the moisture content is lower, while fresh and dry apples exhibit similar absorption spectrums above 3mm wavelengths (Ginzberg, 1969; Kruse et al., 1962). Ginzberg (1969) also demonstrated that with increasing thickness of foods, both transmissivity and absorption decrease simultaneously. Foodstuff thickness and wavelength will vary according to their transmissivity and absorptivity depending on the application. IR processes could benefit from thin slices due to their high transmissivity, whereas NIR with its higher transmissivity may be preferred over both MIR and FIR. In some food products, NIR radiation may be too hot, decreasing absorption and increasing transmisibility. This is particularly relevant for processing thin materials. Depending on how thin a material is, it might have low NIR absorption as it dries. This is because most of the radiation energy can be reflected by the thin layer.
-
Advantages and Disadvantages of Infrared heating The following are five main advantages of IR heating:
-
High energy efficiency: This type of radiation provides heating with high energy efficiencies since the energy is delivered directly to the target object without requiring a delivery medium or dispersing energy to a large area. An IR lamp can provide an energy efficiency of about 80%90%.
-
Convenience: Because IR heating devices are small compared to other heating devices, manufacturers can have a larger working space. In addition, IR heating devices are simple to set up.
-
Clean: With IR heating, there is no need for any medium. This ensures that the air remains clean, a major advantage for the food processing industry.
-
Fast Response: The response speed of an electric IR heater is very fast, so the control of the operating temperature can be achieved with ease. It is easy to change the heating power by merely changing the voltage.
-
Inexpensive: In many industries, IR heating technology has gained popularity because of its lower investment requirement than alternative heating methods.
However, IR heating has several disadvantages, including the inability to heat the interior part of the target object when it has a complex shape. Because the radiation is not uniformly distributed throughout the target object, IR heating is not used for heating the interior of the object. It can be improved by using IR lamps (heat sources) and reflectors. In addition, the heating area can be controlled by the reflector design (Lee and Yang, 2018). A second weakness is the fact that the IR lamp is easily damaged by external environments, so protection of the IR heat source is necessary.
-
-
-
COMPUTATIONAL MODELLING OF INFRARED HEATING PROCESS
When combined with other heating methods, computer modelling of IR heating can provide a valuable tool for optimizing IR food processing and developing IR heating equipment. In order to predict temperature and moisture changes in food materials involved in various IR heating operations, ordinary differential equations or partial differential equations can be numerically solved for suitable boundary and initial conditions, which account for heat and mass transfer in food materials. The major challenges in simulating the IR heating of food materials come from the complexity of the optical properties of foods, determining the incident radiative heat flux at the food surface, radiative energy extinction inside food, and combining the heat transfer phenomena of conduction and convection (Krishnamurthy et al., 2008b; Pan and Atungulu, 2011). A common interest of modelling IR heating has been to predict the IR heat absorption or, in other words, the extent of IR- induced heat penetration in food materials (Datta and Ni, 2002; Tanaka and Uchino, 2010; Prakash, 2011). Recent studies of the penetration of IR radiant energy into a food surface have assumed either a finite or zero penetration depth (Datta and Ni, 2002). In the former case IR power absorption by food was considered as an exponential decay function penetrating from the surface to the interior of food materials, and the approach treats IR radiation power in a similar way to microwave power (Datta and Ni, 2002; Tanaka and Uchino, 2010).
-
OTHER INDUSTRIES EMPLOYING INFRARED HEATING APPLICATION
One of the most widely used applications of IR heating is home heating during the winter months. In general, gas-fired IR heaters are used for large spaces, but electrical IR heaters are common in houses and offices because they provide a comfortable heating temperature (not too hot nor too cold) and can be controlled easily and quickly. (Kalina and Watkinson, 2001) show an electrical IR heater. IR home heaters use IR lamps and reflectors in order to emit IR rays toward a target direction. This application requires a lower temperature than food processing since high temperatures will damage human skin. The temperature requirements for many industrial applications are higher than those required for food processing. It is necessary to heat metal materials to a temperature of about 1000°C in the metal forming industry in order to get the material to flow, so that a product can be produced with the heated metal material. During the forging process, a work piece receives compression forces frequently from two dies with the desired shape. This is one of the popular manufacturing processes used to shape bulk metal materials. In order to reach the target shape within the dies, the work piece must deform. If the material has a high yield stress, it is very difficult to deform the work piece, and the dies or work piece can even be seriously damaged. A convection furnace is usually used to heat up the work piece to a high temperature so that the material can flow freely for fitting the die shape without damaging the dies. Recently, IR heating has received attentions because of its fast heating characteristic and convenience. Kadolkar et al. (2004) reported an application of an IR heating device for a forging process of aluminum Alloys. When compared with convection furnaces, they improved both heating rates and energy efficiency. Lee et al. (2014) used IR heating for sheet metal forming. They developed reflectors to collect IR rays on a target surface in order to partially heat the target area in the whole material. Because this application heats only a limited portion of the material (rather than the entire material), it can save 90% of the energy of a conventional convection heating method (Lee et al., 2017). In the food processing industry, uniformity of heating is extremely important. This method of creating a large temperature gradient differs from IR localized heating because it creates a large temperature gradient. IR heaters can increase the productivity of sheet metal forming since they are small and light, and improve the working environment by making the IR heaters a part of the robot arm. A very important application of IR heating is in the drying of paint specifically for the automotive industry. Painting in the automotive industry plays a significant role in protecting the automobile from corrosion and the environment.
-
APPLICATION OF INFRARED HEATING FOR FOOD PROCESSING.
It has been demonstrated that IR-based processing has various benefits over conventional food processing, such as improving food quality and safety, reducing energy consumption, and reducing waste water generation. Several recent studies have looked at different IR food processes, including dehydrating, blanching, peeling, baking, roasting, pasteurizing, sterilizing, and decontaminating.
-
Infrared Baking
A baking industry product that uses IR heating shortens processing times, improves quality, and consumes less energy (Sumnu and Ozkoc, 2010). As Skjoldebrand (2001) explains, the heat and mass transfer process occured at IR bakes in three distinct phases. The first phase includes an increase in surface temperature to as high as 100°C accompanied by little weight loss. When the moisture is transferred from the interior to the exterior of baked foods during the second period, an evaporation zone forms. During the final stage of baking, the central temperature of the product increases and finally reaches about 98-99°C. About 25% of the total baking time is spent in this phase. In addition to microwave heating and hot air heating, IR baking can be used to improve heat distribution and the speed of the food heating. Many recent studies have investigated the use of microwves and infrared light for baking in order to improve the quality of baked goods and reduce baking time (Datta et al., 2007; Zhang et al., 2005). Combining IR heating with microwave baking enhances spatial uniformity and overall baking rate by using IR heating at different times and locations. Rapid surface heating and moisture removal from a products surface is promoted by IR radiation, which facilitates color and crust formation.
Fig. 5: IR baking machine (A) Industrial IR oven used in baking process (Baker Pacific corporation) (B) Home baking oven (Panasonic corporation) .
-
Infrared Roasting and Grilling
The roasting of food is also a widely used cooking method in which food is heated to achieve maillard browning and caramelization as an aid to creating flavor. Similar to baking, the roasting process has some differences from the latter. Meat and vegetables usually get roasted on an open fan, whereas bread gets baked in a closed oven. Baking requires a lower temperature than roasting to achieve a flavorful crust on the surface of the food, and the roasting process often involves coating the fan with fat (oil or butter), The purpose of this is to reduce the loss of moisture during the baking process. Baked foods, such as muffins and cakes, expand and fill the free space during the baking process, but their structure does not change. Roasted foods are usually heated by convection. A few studies have found that IR radiation is beneficial in the roasting process. The study by Kumar et al. (2009) suggests that using IR radiation for roasting is more energy efficient and convenient than heating food with electrical heat. This is because IR radiation directly heats the target food. By using infrared heating, the efficiency of conversion increased from 50% to 80% without a noticeable effect on quality. The IR radiation used by Kim et al. (2006) and Park et al. (2009) replaced the conventional roasting process of green tea, and their studies have shown that these methods affect the chemical properties of green tea; According to their results, IR heat treatment caused the polyphenolic content of green tea to be increased significantly. Since closed ovens used in roasting processes are also used for heating, combining IR heat treatment with the hot air inside is a worthwhile topic to study. A roasting rate also has an impact on food product quality (Yang et al., 2010; and Mosayebi et al., 2018) optimization model accounts for the effects of IR power and hot air temperature. In grilling, food is placed over an open flame (indirect heating), similar to roasting, but it is not cooked in a closed oven. Grilling is a traditional cooking method in which the food is cooked on a grill rack. When food is grilled, seasoning can be added. Grilling uses a great deal of energy and is also a faster cooking method than roasting, so it is utilized for large foods like steaks and lamb chops. Roasting is slower, but suitable for large meals. Grilling uses fire as a heat source traditionally. Recently, IR grilling has gained attention. By using IR, one can control the power much more easily than with fire, IR grilling is able to improve energy efficiency, and IR is convenient, as well as easy to clean. The role of the IR grilling, which is the first stage of cooking, is to induce crust formation in meat patties.
Fig. 6: IR grilling system (A) IR roaster (Jireh plus corporation) (B) indoor IR grill (Philips corporation) .
Braeckman et al. (2009) have developed a multi-step cooking process involving IR grilling and hot air cooking. Cooking products that are available to home cooks to use in roasting and grilling are made from commercially available coatings that work as barriers to reduce fat and water loss during the second stage of hot air cooking. Fig. 6A shows a portable IR roaster called Bocaboca (Jireh plus). This product can be used for coffee beans and almond nuts. The indoor IR grill shown in Fig. 6B is made by Philips corporation. Its advantages of cleanliness and compactness make it ideal for home use.
-
Infrared Blanching
Blanching is a method of scalding foods in hot water it involves three procedures preheating, blanching, and cooling. It is usually applied as a pre treatment before drying, freezing, or canning food. A blanching process is usually applied to fruits and vegetables in order to remove peels, modify textures, or inactivate enzymes. But the heat treatment can cause tissue damage, protein denaturement, and color loss. In order to eliminate the side effects of blanching, low temperature conditions are generally used so as to mitigate the quality problem. Blanching usually results in damage to tissue cells, protein denaturation, and color loss due to heat treatment. In the food industry, a low-temperature procedure is more time-consuming than a high-temperature procedure, reducing productivity. Hot steam has been considered an efficient heat source for the blanching process, especially for mass production system. The IR blanching system developed by Pan and McHugh (2004) requires less space than conventional blanching equipment, and it reduces processing time and improves product quality. The machine also requires a shorter working time than conventional blanching equipment. After the dry blanching of apple slices, the authors conducted a later study (Zhu et al., 2007) to examine the effects of dipping the slices in ascorbic acid, citric acid, and calcium chloride before the blanching. When compared to non-dipped cases, the dipping treatment proved capable of controlling solid loss and promoting a brighter color. The IR blanching machine used in Zhu and Pan (2009) was able to complete dry-blanching and dehydration simultaneously for apple slices by combining IR blanching and a natural gas dehydration process. Fig 7 shows the IR blanching machine used in the study. The radiation intensity and thickness of the slice have been reported as important factors in the dry-blanching and dehydration process. Bingol et al. (2014) examined the effects of IR blanching on French fries, comparing conventional water blanching to IR blanching. Nutrient retention is an important consideration when blanching to retain nutrients. Galindo et al. (2005) observed microscopic cell damage in carrot slice after IR blanching and water blanching because the degree of damage to food particles throughout the blanching process contributes to the final products quality. Based on the study, it was discovered that IR radiation caused significant cell damage only on the surface of the food, while water caused damage in the middle layer. The IR and water radiations are absorbed almost equally on the surface and the middle layer
of the food is much more damaged by the water. According to Vishwanathan et al. (2013), by using IR blanching followed by IR assisted hot air drying they significantly increased vitamin C retention in carrots compared to samples that were just water blanched.
Fig. 7: IR blanching system (Pan and McHugh, 2004; Zhu et al., 2007; Zhu and Pan, 2009)
-
Infrared Drying
IR energy is being used for drying processes that have moved beyond the early industrial applications to process high value and value-added foods and agricultural products, such as fruits, vegetables, grains, spices, and juices (Pan and Atungulu, 2011). IR radiation can be used to quickly dry products that contain a lot of moisture if they can be spread thinly on a flat surface or reduced in thickness. Different carbohydrates, fats, proteins, and water absorb different amounts of energy, which may also affect the performance of IR drying. Water molecules in foods absorb light at wavelengths similar to those emitted by IR sources in order to achieve effective drying. Furthermore, it is essential for the IR energy incident on the receiving surface to be distributed uniformly, a situation that involves optimizing the configuration of the IR emitters and any reflectors. Heat sources that operate in the infrared reion are often enclosed in a chamber with highly reflective surfaces in order to increase their energy efficiency. This method of drying is advantageous in that it does not need any heating medium for transmitting thermal energy from the source to the target. Food materials with complex shapes and sizes cannot always be dried using IR, which works best with thin, flat materials. IR heating has been combined with other drying methods in order to overcome these limitations, and these methods are considered more effective than radiation or convection heating alone. IR heating in drying processes is typically used for combined heat and hot air drying, sequential IR and freeze drying, and vacuum IR drying (Lin et al., 2009; Nimmol et al., 2005; Pan and Atungulu, 2010). It was shown that using IR and hot air together to dry had a greater synergic effect than using just IR or hot air (Afzal et al.,1999). Nimmol et al. (2005) proposed an FIR vacuum drying system for heat- sensitive materials and found that vacuum drying along with
proper use of FIR radiation could improve both the energy efficiency of the process and the quality of dried products compared with a conventional drying process. In general, a vacuum IR drying system consists of four major components: a drying chamber, IR heaters, a vacuum pump, and a control system. The insulated drying chamber is designed to withstand lower levels of pressure, and a vacuum pump is used to maintain vacuum in the drying chamber. IR heaters inside the drying chamber can supply IR thermal radiation to the drying products, and the operation of the IR radiator is adjusted by the control system. Typically, there are two methods that can be used to control the vacuum IR drying operations. In the first method the surface temperature of a drying product or the temperature of a drying medium presented in a drying chamber determines the heating time and power intensity of the IR heaters (Nimmol et al., 2005, 2007; Swasdisevi et al., 2007). This control strategy is generally applied to a product that could be damaged or sensitive at higher temperatures. Alternatively, IR heating can be controlled by adjusting the energy input to the IR heaters (Afzal & Abe, 1998; Afzal et al., 1999; Mongpraneet et al., 2002), and thus the output of the radiation intensity, which is the radiation power output per unit area, can be regulated to a desired level.
-
Infrared Dry Peeling
IR dry peeling utilizes radiant energy to promote skin separation without requiring the assistance of any chemicals or heating media such as hot water or pressurized steam. In the typical IR dry peeling process, intense thermal radiation generated from high-power IR emitters heats the fruit surface and results in reduced skin rupture strength and accumulated internal vapor under the skin membrane (Li and Pan, 2014a). When the internal vapor accumulates to a certain level under skin, cracks can occur because the skin shear stress induced by the accumulated vapor pressure exceeds the critical rupture stress in the skin membrane (Li et al., 2014a). At a microscopic scale, thermal effects of IR heating are recognized by scanning electron microscope (SEM) images as the melting of extracellular cuticles, collapse of surface cellular layers, thermal expansion of cell walls, and severe degradation of middle lamella and cell wall structures in pericarp tissues, all of which contribute to the loosened skin quantified as the increased peel stiffness, reduced peel adhesiveness, and altered transition temperature by texture analysis (TA) and dynamic mechanical analysis (DMA) (Li et al., 2014a,b,c; Wang et al., 2014). The IR peeling process delivers high-quality finished products with better peelability and firmer peeled product that satisfy the needs of processors and consumers, and reduces peeling loss compared to conventional lye and steam peeling (Pan et al., 2009; Li et al., 2014a). IR peeling could produce at least 20% more premium tomato products than steam peeling and eliminate the usage of water and chemicals of lye peeling. Because no chemicals are used, the removed skins, a common by-product from peeling, can also be easily reutilized as value-added food ingredients (Li et al., 2014b; Li and Pan, 2014b).
Pilot-scale application of an IR dry peeling system for tomatoes produced a percentage of fully peeled tomatoes of 85%, a peeling yield of 82%, and an average thickness of
peeled-off skin of about 0.75 mm when heating for a residence time of 125 s (Pan et al., 2015). Systematic development and successful demonstration of IR dry peeling technique have been carried out on tomatoes, clingstone peaches, and pears (Pan et al., 2015; Li et al., 2014c). Compared to conventional lye peeling, IR dry peeling of tomatoes, peaches, and pears showed a lower peeling loss, a thinner thickness of peeled skin, and a firmer texture of peeled products while achieving a similar ease of peeling (Li, 2012; Li et al., 2014c) (Fig. 8).
Fig. 8: Infrared-peeled tomatoes (left) had better peelability, firmness, and reduced loss compared to steam-peeled tomatoes (right). Courtesy: JBT Corporation, Madera, CA, USA .
Fig. 9: Microbial inactivation system for hotdogs (Huang and Sites, 2008).
-
Infrared Microbial inhibition
In the food industry, microbial inactivation processes have become more important as food demand has increased and distribution channels have become more diverse. It is imperative that pathogenic and spoilage organisms are inactivated before food products are packaged to prevent food decay during the distribution process. One of the most effective ways to inactivate microorganisms is to provide
heat energy to kill the bacteria. In the inactivating process, since the thermal energy should not damage the final quality of the food product, the heating temperature in the process of microbial inactivation should be optimized. Because of this, IR heating is the best method of microbial inactivation due to its ease of control and rapid heating. It was shown, for example, that IR heating in a chamber reduces bacterial contamination more quickly than conductive heat (Sawai et al., 2003). On the basis of the above advantages, IR heating has a considerable potential as a method for inactivating microorganisms in food processes. Huang and Sites (2008) developed an IR heating process for inactivation of surface- contaminated for hotdogs. Fig. 9 shows the schematics of their automatic temperature control machine for microbial inactivation (Huang and Sites, 2008). Control of the surface temperature and the holding time during heat treatment are critical factors that contribute to microbial inactivation quality. This technique can also be used for other food products. Inactivation of bacteria processes is also affected by the wavelength of IR treatment because of the effects on water molecules in bacteria (Hamanaka et al., 2006).
In order to improve the efficiency of the inactivation process, Jun and Irudayaraj (2003) used an optical filter in conjunction with a selective IR heating platform to inactivate corn meal microbially. We selected an appropriate range of wavelength from IR radiation using an optical filter. In experiments, selective IR heating provided more potential energy to fungal spores compared to general IR heating methods, and numerical modelling accurately predicted the experimental results. IR heating is faster than other heating methods, but not as rapid as microwave in terms of microbial inactivation rate (Hebbar et al., 2003). A combination of IR heating with other heat sources is also possible in microbial inactivation processes. Tanaka et al. (2007) conducted combined IR and hot air heat treatment for microbial inactivation of strawberries.
-
Infrared Thawing
Many food products are frozen to be stored and transported as refrigeration use increases and food transportation becomes more common. In order to prepare frozen foods for further processing, they need to be thawed. Thawing affects food quality significantly. Food products that are over- thawed result in wasting heating energy, which damages product quality. Food products that are under thawed cannot be used in subsequence processes (Backi, 2018). There are many thawing methods, including hot air convection, water, high pressure, microwaves, and infrared heaters, but IR heating is also useful for thawing (Sakai and Hanzawa, 1994). Hong et al. (2009) studied the thawing process using combination of air and IR radiation for pork. They investigated process parameters that affect the quality of the food. In the study, increasing air velocity tended to increase thawing loss compared to the case of increasing IR power, and it was important to control the thawing temperature and humidity to prevent change of color and surface drying. Liu et al. (1999) completed a thawing process for frozen tuna using infrared radiation and applied heat transfer equations to predict the thawing temperature and optimize the process. In a separate study, Seyhun et al. (2009) explored a combined
microwave and infrared heating method for thawing frozen potatoes. Their model showed good agreement with experimental results in predicting thawing temperatures. As shown by these modelling studies, controlling thawing temperature and rate is essential to minimizing food damage. In addition to the examples provided above, IR heating has many other advantages that can be applied to other food processing procedures. The study of Rim et al. (2005) demonstrated that IR heat treatment was effective in increasing the antioxidant activity of peanut shell extracts. The study determined that 5 minutes of IR heat treatment was sufficient to increase antioxidants activities. In food manufacturing, thermal IR heating is used to maintain the temperature of food, as temperature is a major factor affecting taste. A portable food serving machine is shown in Fig. 10. It is equipped with an infrared heater at the top, which keeps food at the proper temperature.
Fig. 10: Food serving machine with IR heat lamp to maintain the food temperature (Lee, 2020) .
-
Infrared Heating for improving food safety
Heat is normally used for treating food materials to inactivate microorganisms and achieve pasteurization or sterilization. However, due to exposure to high temperature, there can be a significant loss in the sensory and nutritional quality of the food (Krishnamurthy et al., 2010a). IR heating can be effectively used for pathogen inactivation, in particular, for surface pasteurization applications. Its application in improving food safety is gaining popularity due to its higher thermal efficiency and faster heating rates and response time in comparison to conventional heating (Krishnamurthy et al., 2008b). IR heating can be used to inactivate bacteria, spores, yeasts, and molds in both liquid and solid foods. Resistance of bacteria, yeasts, and molds to IR heating may be different due to their structural and compositional differences. Inactivation mechanisms of microorganisms by IR heating are similar to those of ultraviolet light and microwave heating, i.e., a combination of thermal and DNA damage effects. Treatment of microorganisms via IR heating results in breakage of cell walls, cytoplasmic content leakage, cytoplasm shrinkage and degradation in mesosome, and damage to the cytoplasm as evidenced by transmission
electron microscopy (Krishnamurthy et al., 2010b). The efficacy of microbial inactivation by IR heating depends on IR power level; heating rate, peak wavelength, and bandwidth of IR heating source; product physical shape and dimensions; types of microorganisms; physiological phase of microorganisms (exponential growth or stationary phase); and types of food materials (Krishnamurthy et al., 2010a). Absorption of IR radiation by water molecules in the microorganism is a significant factor in their inactivation resulting in a rapid increase in temperature (Hamanaka et al., 2006). Increase in the power of the IR heating source increases the temperature and total energy absorbed by microorganisms, leading to microbial inactivation in a short time (Molin and Ostlund, 1975; Bingol et al., 2011). An increase in sample dimension slows the temperature increase of the food sample down and therefore decreases the microbial inactivation efficiency (Hashimoto et al., 1991; Sawai et al., 1997). Microbial components in food absorb certain wavelengths of IR radiation at a higher rate than others. Therefore, inactivation of microorganisms using selective bandwidth IR heating can ensure pathogen inactivation and minimize changes in food quality (Krishnamurthy et al., 2010b; Jun and Irudayaraj, 2004; Hamanaka et al., 2006). IR heating is more effective in the surface pasteurization of vegetative cells compared to conventional conductive or convective heating method (Sawai et al., 2000).
Several studies on use of IR heating for different products and pathogens have been conducted. IR heating was used for surface pasteurization of cottage cheese and sterilization of wheat surface (Rosenthal et al., 1996). Jun and Irudayaraj (2004) applied IR heating for disinfection of fungal spores in agricultural materials. Huang (2004) used IR heating to pasteurize the surface of turkey frankfurters contaminated with Listeria monocytogenes. IR heating has been applied for the pasteurization of oysters, Japanese noodles, and secondary pasteurization of boiled fish paste (Sakai and Mao, 2006), as well as for the surface decontamination of strawberry (Tanaka et al., 2007), paprika powder (Staack et al., 2008a,b), inactivation of Staphylococcus aureus in milk (Krishnamurthy et al., 2008a), L. monocytogenes on hot dogs (Huang and Sites, 2008), and Salmonella enteritidis on almonds (Brandl et al., 2008). Bingol et al. (2011) pasteurized almonds with IR, which reduced the Pediococcus population size by more than 5-log without affecting the appearance, texture, flavor, and overall quality of pasteurized almonds. IR heating followed by tempering for 120 min resulted in 2.5-and 8.3-log reductions of Aspergillus flavus spores in rough rice (Wang et al., 2014b). IR heating can also be applied on process lines to pasteurize food-contact surfaces for elimination of fungal growth and microorganisms to improve the shelf life of food products, such as, e.g., the pasteurization of baking trays (Krishnamurthy et al., 2010b).
Table 2: Commercial food applications using IR heating system (Source: Erdogdu et al., 2010)
Equipment
Type of application
Food processed
Baking/ Heating
Gas oven
Batch
Bread
Tunnel oven
Continuous
Fish paste, oyster, egg liver
Drum oven
Continuous
Coffee
Cooker
Batch
Sweet potato, chestnut
Drying
Tunnel dryer
Continuous
Vegetables, flaked dried bonito, semidried noodle
Drum dryer
Batch
Green tea
Freeze dryer
Continuous
Liquid foods
Pneumatic dryer
Continuous
Particulate foods
Thawing
Tunnel thawing equipment
Continuous
Frozen fish paste
Thawing device
Batch
Frozen sushi & other frozen foods, tuna
Pasteurization
Conveyor pasteurizer
Continuous
Packed foods
Peeling
Infrared dry peeling
Continuous and Batch
Tomatoes & Potatoes
Blanching
Infrared dry blancher
Infrared dry blancher
Strawberry, carrots, etc.
-
-
FUTURE WORKS
To ensure product quality control in industrial applications, the durability of the IR heat source is essential. Therefore, managing the durability of the IR heat source is vital. The cooling system, reflectivity, and lifetime of IR lamps should be more carefully controlled than those of IR heat sources heated by gas. Infrared lamps have a life span that is strongly affected by the voltage. Frequent voltage changes or over- voltage operations severely reduce the life span of lamps; IR lamps typically have a life span of 10,000 hours. In addition to thermal deformation of the quartz tube, an IR lamp must be operated with a cooling system to prevent overheating and thermal deformation. It is possible that the thermal deformation of the glass tube may present a safety hazard. Studies on monitoring and cooling systems of IR heating systems have not been adequately reported, meaning more research is needed. Also, maintaining the reflectivity of the reflector is important to keep the efficiency of the heating process since the reflectivity directly affects the reflected radiation. Reflectors are manufactured with anodized aluminum alloys to have a high reflectivity. However, achieving both high reflectivity and durability of the reflector is challenging. A new coating process was recently presented by Hu et al. (2018) to achieve high reflectivity in dusty and moist environments. This issue also requires further research. Numerical modelling is one of the essential methods to optimize the process. Some studies have resulted in analytic solutions (Hernández et al., 2000; Li, 2010; GarcÃa Alvarado et al., 2014; Swasdisevi et al., 2009; Shahari et al., 2016), and the analytic solutions are able to provide insight into the processes and fast solutions. However, the analytic solutions are based on strong assumptions, and it is not easy to obtain three-dimensional analytic solutions.
-
CONCLUSION
This Review article discusses IR heating applications in food processing, the design of IR heaters, numerical simulations of IR heating, and applications for other industries as well as food processes. The same principles apply to all industrial fields, including radiation theory, reflector design, and heat transfer balance, but the mass transfer phenomenon and wavelength sensitivity are particularly relevant to the food industry. In this seminar report, the reviewed content presents a general overview of IR heating technologies and gives examples of how they are used in industrial applications. IR heating technology has dramatically developed over time, and is used in many fields. IR heating in food processing and other industries can be more widely and effectively utilized when combined with new developing technologies. However, comprehensive research is still needed to improve the efficiency and reach of IR heating. Food-processing technologies based on infrared technology could open new avenues for delivering desirable foods to consumers while conserving natural resources. In addition to selective IR heating, IR can be used in conjunction or in sequence with other technologies to create higher-quality food products. Selective heating may also eliminate the need for preservatives. It is also possible to combine IR with other treatment methods to offer diverse food-processing advantages, such as improved process efficiency, product characteristics, and safety.
-
REFERENCES
-
Aboud, S. A., Altemimi, A. B., RS Al-HiIphy, A., Yi-Chen, L., & Cacciola, F. (2019). A comprehensive review on infrared heating applications in food processing. Molecules, 24(22), 4125.
-
Afzal, T. M., & Abe, T. (1998). Diffusion in potato during far infrared radiation drying. Journal of food engineering, 37(4), 353-365.
-
Afzal, T. M., Abe, T., & Hikida, Y. (1999). Energy and quality aspects during combined FIR-convection drying of barley. Journal of food engineering, 42(4), 177-182.
-
Almeida, M. F. (2005). Modeling infrared and combination infrared- microwave heating of foods in an oven. Cornell University.
-
Andriyanov, Y. V., & Bondarenko, O. N. (1986). Focussing of a spherical acoustic pulse by an elliptical reflector in medical equipment for the contactless fragmentation of kidney stones. Biomedical Engineering, 20(3), 77-80.
-
Backi, C. J. (2018). Methods for (industrial) thawing of fish blocks: A review. Journal of Food Process Engineering, 41(1), e12598.
-
Bingol, G., Wang, B., Zhang, A., Pan, Z., & McHugh, T. H. (2014). Comparison of water and infrared blanching methods for processing performance and final product quality of French fries. Journal of Food Engineering, 121, 135-142.
-
Bingol, G., Yang, J., Brandl, M. T., Pan, Z., Wang, H., & McHugh, T.
H. (2011). Infrared pasteurization of raw almonds. Journal of Food Engineering, 104(3), 387-393.
-
Braeckman, L., Ronsse, F., Hidalgo, P. C., & Pieters, J. (2009). Influence of combined IR-grilling and hot air cooking conditions on moisture and fat content, texture and colour attributes of meat patties. Journal of food engineering, 93(4), 437-443.
-
Brandl, M. T., Pan, Z., Huynh, S., Zhu, Y., & McHugh, T. H. (2008). Reduction of Salmonella Enteritidis population sizes on almond kernels with infrared heat. Journal of food protection, 71(5), 897-902.
-
Cutler, C. C. (1947). Parabolic-antenna design for microwaves. Proceedings of the IRE, 35(11), 1284-1294.
-
Das, I., & Das, S. K. (2010). Emitters and infrared heating system design. Infrared Heating for Food and Agricultural Processing, Z. Pan and GG Atungulu, Eds, 57-88.
-
Datta, A. K., & Ni, H. (2002). Infrared and hot-air-assisted microwave heating of foods for control of surface moisture. Journal of Food Engineering, 51(4), 355-364.
-
Datta, A. K., Geedipalli, S. S., & Almeida, M. F. (2005). Microwave combination heating. Food Technology.
-
Datta, A. K., Sahin, S., Sumnu, G., & Keskin, S. O. (2007). Porous media characterization of breads baked using novel heating modes. Journal of Food Engineering, 79(1), 106-116.
-
Galindo, F. G., Toledo, R. T., & Sjöholm, I. (2005). Tissue damage in heated carrot slices. Comparing mild hot water blanching and infrared heating. Journal of food engineering, 67(4), 381-385.
-
Ginzburg, A. S. (1969). Application of infra-red radiation in food processing.
-
Gordon, J. M., Feuermann, D., & Huleihil, M. (2002). Laser surgical effects with concentrated solar radiation. Applied physics letters, 81(14), 2653-2655.
-
Hamanaka, D., Uchino, T., Furuse, N., Han, W., & Tanaka, S. I. (2006). Effect of the wavelength of infrared heaters on the inactivation of bacterial spores at various water activities. International journal of food microbiology, 108(2), 281-285.
-
Hashimoto, A. (1991). Effect of Far Infrated Radiation on Pasteurization of Bacteria Suspended in Phosphate-Buffered Saline. Kagaku Kogaku Ronbunshu, 17, 627-633.
-
Hebbar, H. U., Nandini, K. E., Lakshmi, M. C., & Subramanian, R. (2003). Microwave and infrared heat processing of honey and its quality. Food Science and Technology Research, 9(1), 49-53.
-
ong, G. P., Shim, K. B., Choi, M. J., & Min, S. G. (2009). Effects of air blast thawing combined with infrared radiation on physical properties of pork. Food Science of Animal Resources, 29(3), 302-309.
-
Hou, Z., Li, X., Huang, Y., & Ho, S. T. (2013). Physics of elliptical reflectors at large reflection and divergence angles I: Their design for nano-photonic integrated circuits and application to low-loss low- crosstalk waveguide crossing. Optics Communications, 287, 96-105.
-
Huang, L. (2004). Infrared surface pasteurization of turkey frankfurters. Innovative Food Science & Emerging Technologies, 5(3), 345-351.
-
Huang, L., & Sites, J. (2008). Elimination of Listeria monocytogenes on hotdogs by infrared surface treatment. Journal of food science, 73(1), M27-M31.
-
Jun, S., & Irudayaraj, J. (2003). A dynamic fungal inactivation approach using selective infrared heating. Transactions of the ASAE, 46(5), 1407.
-
Jun, S., & Irudayaraj, J. (2004). Explore the mechanism of selective infrared heating on disinfection of fungal spores. App. Eng. Agriculture, 20, 481.
-
Kadolkar, P. B., Lu, H., Blue, C. A., Ando, T., & Mayer, R. (2004, April). Application of rapid infrared heating to aluminum forgings. In Proceedings of the 25th Forging Industry Technical Conference of the Forging Industry Association and the Forging Industry Educational and Research Foundation (pp. 19-21).
-
Kalina, L.A., and Watkinson,C. J., US patent No.D456067, S1, 2001.
-
Kandilli, C., & Ulgen, K. (2009). Review and modelling the systems of transmission concentrated solar energy via optical fibres. Renewable and Sustainable Energy Reviews, 13(1), 67-84.
-
Kim, S. Y., Jeong, S. M., Jo, S. C., & Lee, S. C. (2006). Application of far-infrared irradiation in the manufacturing process of green tea. Journal of agricultural and food chemistry, 54(26), 9943-9947.
-
Krishnamurthy, K., Jun, S., Irudayaraj, J., & Demirci, A. (2010a). Infrared Radiation Heating for Food Safety Improvement. Infrared Heating for Food and Agricultural Processing, 225.
-
Krishnamurthy, K., Jun, S., Irudayaraj, J., & Demirci, A. L. I. (2008a). Efficacy of infrared heat treatment for inactivation of staphylococcus aureus in milk. Journal of food process engineering, 31(6), 798-816.
-
Krishnamurthy, K., Khurana, H. K., Soojin, J., Irudayaraj, J., & Demirci,
-
(2008b). Infrared heating in food processing: an overview. Comprehensive reviews in food science and food safety, 7(1),
2-13.
-
Krishnamurthy, K., Tewari, J. C., Irudayaraj, J., & Demirci, A. (2010b). Microscopic and spectroscopic evaluation of inactivation of Staphylococcus aureus by pulsed UV light and infrared heating. Food and Bioprocess Technology, 3(1), 93-104.
-
Kruse, P. W., McGlauchlin, L. D., & McQuistan, R. B. (1962). Elements of infrared technology: Generation, transmission and detection. New York: Wiley.
-
Kumar, C. M., Appu Rao, A. G., & Singh, S. A. (2009). Effect of infrared heating on the formation of sesamol and quality of defatted flours from Sesamum indicum L. Journal of food science, 74(4), H105- H111.
-
Lampinen, M. J., Ojala, K. T., & Koski, E. (1991). Modeling and measurements of infrared dryers for coated paper. Drying Technology, 9(4), 973-1017.
-
Lee, E. H. (2020). A review on applications of infrared heating for food processing in comparison to other industries. Innovative Food Processing Technologies: A Comprehensive Review, 431-455.
-
Lee, E. H., & Yang, D. Y. (2018). Design and analysis of infrared heating with overlapping heater array scheme for industrial application. Heat Transfer Engineering, 39(15), 1344-1354.
-
Lee, E. H., Yang, D. Y., & Ko, S. (2017). A study on infrared local heat treatment for AA5083 to improve formability and automotive part forming. Journal of Materials Engineering and Performance, 26(10), 5056-5063.
-
Lee, E. H., Yang, D. Y., & Yang, W. H. (2014). Numerical modeling and experimental validation of focused surface heating using near- infrared rays with an elliptical reflector. International Journal of Heat and Mass Transfer, 78, 240-250.
-
Lee, S. C., Jeong, S. M., Kim, S. Y., Park, H. R., Nam, K. C., & Ahn, D.
U. (2006). Effect of far-infrared radiation and heat treatment on the antioxidant activity of water extracts from peanut hulls. Food chemistry, 94(4), 489-493.
-
-
-
Li, B. X., Lu, Y. P., Liu, L. H., Kudo, K., & Tan, H. P. (2005). Analysis of directional radiative behavior and heating efficiency for a gas-fired radiant burner. Journal of Quantitative Spectroscopy and Radiative Transfer, 92(1), 51-59.
-
Li, X. (2012). A Study of Infrared Heating Technology for Tomato Peeling: Process Characterization and Modeling (PhD. Dissertation). University of California, Davis. Department of Biological and Agricultural Engineering.
-
Li, X., & Pan, Z. (2014a). Dry-peeling of tomato by infrared radiative heating: part I. Model development. Food and Bioprocess Technology, 7(7), 1996-2004.
-
Li, X., & Pan, Z. (2014b). Dry peeling of tomato by infrared radiative heating: Part II. Model validation and sensitivity analysis. Food and Bioprocess Technology, 7(7), 2005-2013.
-
Li, X., Zhang, A., Atungulu, G. G., Delwiche, M., Milczarek, R., Wood, D., … & Pan, Z. (2014c). Effects of infrared radiation heating on peeling performance and quality attributes of clingstone peaches. LWT-Food Science and Technology, 55(1), 34-42.
-
Lin, Y. L., Li, S. J., Zhu, Y., Bingol, G., Pan, Z., & McHugh, T. H. (2009). Heat and mass transfer modeling of apple slices under simultaneous infrared dry blanching and dehydration process. Drying technology, 27(10), 1051-1059.
-
Liu, C. M., Sakai, N., & Hanzawa, T. (1999). Three dimensional analysis of heat transfer during food thawing by far-infrared radiation. Food Science and Technology Research, 5(3), 294-299.
-
MacConnell, J. D. (1972). Low Temperature Catalytic Heaters. Platinum Metals Review, 16(1), 16-21.
-
Molin, G., & Östlund, K. (1975). Dry-heat inactivation of Bacillus subtilis spores by means of infra-red heating. Antonie van Leeuwenhoek, 41(1), 329-335.
-
Mongpraneet, S., Abe, T., & Tsurusaki, T. (2002). Far infraredvacuum andconvection drying of welsh onion. Transactions of the ASAE, 45(5), 1529.
-
Mosayebi, M., Kashaninejad, M., & Najafian, L. (2018). Optimizing physiochemical and sensory properties of infrared-hot air roasted sunflower kernels using response surface methodology. Journal of Food Quality, 2018.
-
Nimmol, C., Devahastin, S., Swasdisevi, T., & Soponronnarit, S. (2005). Drying kinetics and quality of carrot dried by far-infrared radiation under vacuum condition. In International Agricultural Engineering Conference.
-
Nimmol, C., Devahastin, S., Swasdisevi, T., & Soponronnarit, S. (2007). Drying of banana slices using combined low-pressure superheated steam and far-infrared radiation. Journal of food engineering, 81(3), 624-633.
-
Nindo, C., ≈ Mwithiga, G. (2010). Infrared drying. Infrared heating for food agricultural processing.
-
Nowak, D., & Lewicki, P. P. (2004). Infrared drying of apple slices. Innovative Food Science & Emerging Technologies, 5(3), 353-360.
-
Pan and Atungulu, (2010). Commercialization of food processing using infrared. In: Case Studies in Novel Food Processing Technologies: Innovations in Processing, Packaging and Predictive Modelling. Woodhead Publishing Limited.
-
Pan, Z., & Atungulu, G. G. (2010). Infrared heating for food and agricultural processing. CRC Press.
-
Pan, Z., & Atungulu, G. G. (2011). Combined infrared heating and freeze-drying. Infrared heating for food and agricultural processing, 117-140.
-
Pan, Z., Atungulu, G. G., & Li, X. (2014). Infrared heating. In Emerging technologies for food processing (pp. 461-474). Academic Press.
-
Pan, Z., Khir, R., Godfrey, L. D., Lewis, R., Thompson, J. F., & Salim,
-
(2008). Feasibility of simultaneous rough rice drying and disinfestations by infrared radiation heating and rice milling quality. Journal of food engineering, 84(3), 469-479.
-
-
Pan, Z., Li, X., Bingol, G., McHugh, T. H., & Atungulu, G. G. (2009). Development of infrared radiation heating method for sustainable tomato peeling. Applied engineering in agriculture, 25(6), 935-941.
-
Pan, Z., Li, X., Khir, R., El-Mashad, H. M., Atungulu, G. G., McHugh,
T. H., & Delwiche, M. (2015). A pilot scale electrical infrared dry- peeling system for tomatoes: Design and performance evaluation. Biosystems engineering, 137, 1-8.
-
Park, J. H., Lee, J. M., Cho, Y. J., Kim, C. T., Kim, C. J., Nam, K. C., &
Lee, S. C. (2009). Effect of farinfrared heater on the physicochemical characteristics of green tea during processing. Journal of food biochemistry, 33(2), 149-162.
-
Prakash, B. (2011). Mathematical modeling of moisture movement within a rice kernel during convective and infrared drying (Doctoral dissertation, University of California, Davis).
-
Quaschning, V., & Muriel, M. B. (2002). Solar power-Photovoltaics Or Solar Thermal Power Plants?. Vgb Powertech-International Edition-, 82, 48-52.
-
Ramaswamy, H. S., & Marcotte, M. (2005). Food processing: principles and applications. CRC Press.
-
Rim, A. R., Jung, E. S., Jo, S. C., & Lee, S. C. (2005). Effect of far- infrared irradiation and heat treatment on the antioxidant activity of extracts from peanut (Arachis hypogaea) shell. Journal of the Korean Society of Food Science and Nutrition, 34(7), 1114-1117.
-
Rosenthal, I. (1992). Infrared radiation. In Electromagnetic Radiations in Food Science (pp. 105-114). Springer, Berlin, Heidelberg.
-
Rosenthal, I., Rosen, B., & Bernstein, S. (1996). Surface pasteurization of cottage cheese. Milchwissenschaft (Germany).
-
Sakai, N., & Hanzawa, T. (1994). Applications and advances in far- infrared heating in Japan. Trends in food science & technology, 5(11), 357-362.
-
Sandu, C. (1986). Infrared radiative drying in food engineering: a process analysis. Biotechnology progress, 2(3), 109-119.
-
Saravacos, G., & Kostaropoulos, A. E. (2016). Design of food processes and food processing plants. In Handbook of Food Processing Equipment (pp. 1-50). Springer, Cham.
-
Sawai, J., Fujisawa, M., Kokugan, T., Shimizu, M., Igarashi, H., Hashimoto, A., & Kojima, H. (1997). Pasteurization of bacterial spores in liquid medium by far-infrared irradiation. Journal of chemical engineering of Japan, 30(1), 170-172.
-
Sawai, J., Sagara, K., Hashimoto, A., Igarashi, H., & Shimizu, M. (2003). Inactivation characteristics shown by enzymes and bacteria treated with farinfrared radiative heating. International journal of food science & technology, 38(6), 661-667.
-
Sawai, J., Sagara, K., Kasai, S., Igarashi, H., Hashimoto, A., Kokugan, T., … & Kojima, H. (2000). Far-infrared irradiation-induced injuries to Escherichia coli at below the lethal temperature. Journal of Industrial Microbiology and Biotechnology, 24(1), 19-24.
-
Seyhun, N. A. D. . D. E., Ramaswamy, H., Sumnu, G., Sahin, S., & Ahmed, J. (2009). Comparison and modeling of microwave tempering and infrared assisted microwave tempering of frozen potato puree. Journal of food engineering, 92(3), 339-344.
-
Siegel, R., & Howell, J. R. (1981). Thermal radiation heat transfer, Hemisphere, Washington, D.
-
Siegel, R., & Howell, J. R. (1992). Thermal radiation heat transfer. NASA STI/Recon Technical Report A, 93, 17522.
-
Skjoldebrand, C., (2001). Infrared heating. In Thermal technologies in food processing. In: Richardson, P. (Ed.), Woodhead Publishing, Cambridge, UK, 208-228.
-
Staack, N., Ahrné, L., Borch, E., & Knorr, D. (2008a). Effect of infrared heating on quality and microbial decontamination in paprika powder. Journal of Food Engineering, 86(1), 17-24.
-
Staack, N., Ahrné, L., Borch, E., & Knorr, D. (2008b). Effects of temperature, pH, and controlled water activity on inactivation of spores of Bacillus cereus in paprika powder by near-IR radiation. Journal of food engineering, 89(3), 319-324.
-
Sumnu, S. G., & Ozkoc, S. O. (2010). Infrared Baking and Roasting. Infrared heating for food and agricultural processing, 203.
-
Swasdisevi, T., Devahastin, S., Ngamchum, R., & Soponronnarit, S. (2007). Optimization of a drying process using infrared-vacuum drying of Cavendish banana slices. Optimization, 29(3), 810.
-
Tanaka, F., Uchino, T. (2010). Heat and Mass Transfer Modeling of Infrared Radiation for Heating. In Infrared Heating for Food and Agricultural Processing. In: Pan, Z., Atungulu, G. (Eds.), CRC Press, pp. 41-55.
-
Tanaka, F., Verboven, P., Scheerlinck, N., Morita, K., Iwasaki, K., & Nicolaï, B. (2007). Investigation of far infrared radiation heating as an alternative technique for surface decontamination of strawberry. Journal of Food Engineering, 79(2), 445-452.
-
Vishwanathan, K. H., Giwari, G. K., & Hebbar, H. U. (2013). Infrared assisted dry-blanching and hybrid drying of carrot. Food and Bioproducts Processing, 91(2), 89-94.
-
Wang, B., Khir, R., Pan, Z., El-Mashad, H., Atungulu, G. G., Ma, H., … & Wu, B. (2014b). Effective disinfection of rough rice using infrared radiation heating. Journal of food protection, 77(9), 1538-1545.
-
Wang, Y., Li, X., Sun, G., Li, D., & Pan, Z. (2014). A comparison of dynamic mechanical properties of processing-tomato peel as affected by hot lye and infrared radiation heating for peeling. Journal of food engineering, 126, 27-34.
-
Yang, J., Bingol, G., Pan, Z., Brandl, M. T., McHugh, T. H., & Wang,
H. (2010). Infrared heating for dry-roasting and pasteurization of almonds. Journal of Food Engineering, 101(3), 273-280.
-
Zhang, J., Datta, A. K., & Mukherjee, S. (2005). Transport processes and large deformation during baking of bread. AIChE Journal, 51(9), 2569- 2580.
-
Zhu, Y. I., Pan, Z., & McHugh, T.H. (2007). Effect of dipping treatments on color stabilization and texture of apple cubes for infrared dryblanching process. Journal of food processing and preservation, 31(5), 632-648.
-
Zhu, Y., & Pan, Z. (2009). Processing and quality characteristics of apple slices under simultaneous infrared dry-blanching and dehydration with continuous heating. Journal of food engineering, 90(4), 441-452.