
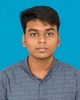
- Open Access
- Authors : Jeneetha Jebanazer , Rryan J , Anirudh Vasudevan
- Paper ID : IJERTV11IS060069
- Volume & Issue : Volume 11, Issue 06 (June 2022)
- Published (First Online): 13-06-2022
- ISSN (Online) : 2278-0181
- Publisher Name : IJERT
- License:
This work is licensed under a Creative Commons Attribution 4.0 International License
A Study on the Architecture of Quantum Computers
Mrs. Jeneetha Jebanazer1(PhD), Rryan J2, Anirudh Vasudevan3
1 Professor, Department of Electronics & Communication Engineering,Panimalar Engineering College, Chennai,Tamilnadu -600123
2UG sholar/ Department of CSBS
3UG sholar/ Department of CSE
SRM Institute of Science and Technology/ Kattankulathur
Abstract: Computers minimise human effort while also focusing on improving efficiency in order to advance technology. To improve machine efficiency, a variety of methods have been invented. Reducing the size of the transistors used in the systems is one such process. The use of quantum computers is another important strategy. When used to factor large numbers, it proved to be very accurate. It was discovered that it could decode codes in 20 minutes, while traditional computers took billions of years. This provided excellent motivation for concentrating on this topic. A quantum machine may have three states for a quantum bit, or qubit: 0, 1, and 0 or 1.The coherent state is the final state. This allows you to perform an operation on two different values at the same time. This, however, raises the issue of decoherence. Using quantum computers to perform the computation becomes difficult. A quantum computer should have five features: a scalable device, an initializable state, a long decoherence time, a universal set of quantum gates, and high measurement efficiency. The architecture of quantum computers is a new field of research. Quantum arithmetic, error management, and cluster-state computing also have an effect on it. The quantum algorithms would not be as effective without it. The algorithms used should be based on quantum parallelism to completely harness the power of a quantum computer. This paper briefly explores a few of the fundamental principles in quantum computing. The paper also looks at the various quantum computer architectures that have been proposed in the literature. The problem of building a functional large-scale quantum computer, however, remains unsolved.
Key Words: Classical computers, quantum computers, quantum computer systems
-
INTRODUCTION
Quantum computing is the use of quantum phenomena such as superposition and entanglement to perform computation. Computers that perform quantum computations are known as quantum computers. Quantum computers are able to solve certain computational problems, such as integer factorization (which underlies RSA encryption), substantially faster than classical computers. We experience the benefits of classical computing every day. However, there are challenges that todays systems will never be able to solve. For problems above a certain size and complexity, we dont have enough computational power to tackle them. To stand a chance at solving some of these problems, we need a new kind of computing.
-
QUANTUM BIT (QUBIT)
Bit is the most fundamental concept in computer science. There are two states for the bits in classical computation, which are 0 and 1. Nevertheless, the subjects are completely different in quantum space. There isn't a limited state for the electrons. As mentioned in the previous section, atomic particles behave like a wave and they do not have deterministic position. As a result, quantum information and computation benefited from many aspects such as its foundation, which is quantum bit or qubit.
-
QUANTUM COMPUTER PRINCIPLES
The superposition principle and entanglement form the backbone of quantum Computers.
-
SUPERPOSITION STATE
Electrical signals such as voltages reflect the 0 and 1 states as one-bit information in traditional computers. Four states are represented by two bits. 00, 01, 10, and 11 bits can represent 2n states, and n bits can represent 2n states. The one-bit information is represented in the quantum computer by a quantum bit called a "qubit," which is a two-state device. In traditional computers, an electron can be used as a qubit instead of an electrical signal. An electron's spin-up and spin-down reflect two states: 0 and 1, respectively. A photon may be used as a qubit, with the horizontal and vertical polarisation of the photon representing both states. Using qubits, quantum computers can perform arithmetic and logical operations as does a classical computer. The important difference, however, is that one qubit can also represent the superposition of 0 and 1 states. The superposition principle is the idea that a system is in all possible states at the same time, until it is measured as shown in Fig.1
Fig.1 Superposition Principle
-
ENTANGLEMENT
Two objects, when quantum mechanically entangled are strongly related to each other even if they are a vast distance apart as shown in Fig.2. Entanglement is another counter-intuitive phenomenon of quantum mechanics. When each particle's quantum state cannot be represented independently of the quantum state of the other, a pair or
group of particles is said to be entangled. Although the parts of the system are not in a definite state, the quantum state of the system as a whole can be represented. There is a special relation between two qubits when they are entangled. The results of the measurements will reveal the entanglement. The measurements on the individual qubits could result in a 0 or a 1. However, the result of one qubit's measurement will always be compared with the result of the other qubit's measurement. And when the particles are separated by a broad distance, this is still the case. The Bell states are an example of such states.
Fig.2 Entanglement
Two particles, for example, are formed in such a way that the system's total spin is zero. If one of the particles' spin is determined on one axis and found to be counterclockwise, a calculation of the spin of the other particle (along the same axis) will always show the spin to be clockwise. This is odd because it appears that one of the entangled particles "feels" a calculation on the other entangled particle and "knows" what the result should be, but this is not the case. This occurs without the entangled particles exchanging any information. And if they were billions of miles apart, the entanglement would still exist. A common misunderstanding is that entanglement could be used to instantaneously send information from one point to another. This is not possible because although it is possible to know the state of the other particle when measuring one, the measurement results of the individual particles are random. There is no way to predetermine the individual result, therefore it is not possible to send a message in this way.
temperatures as near to absolute zero as possible as shown in Fig. 4
Fig.4 Quantum Computers
The Fig.5 shows the internal blocks in the architecture of Quantum Computers. It comprises of Qubit Signal Amplifier followed by Microwave lines, Coaxial Lines, cryogenic isolators, Quantum Amplifiers, cryperm shield and Mixing Chamber
Fig.5 Internal Blocks of Quantum Computers
-
-
QUANTUM COMPUTER
Classical computers (laptop, tablet, phone) operate on bits- 0s and 1s. A quantum computer is fundamentally different. It uses a quantum bit (qubit) which can be both 0 and 1 at the same time as shown in Fig .3.
Fig.3 Quantum Computing
3.1 INSIDE QUANTUM COMPUTERS
Qubits are used in quantum computers to harness the force of quantum mechanics. The operations inside qubits, on the other hand, are insecure and highly sensitive. To date, qubits have had to meetsome unique and difficult criteria in order to work properly. They need a vacuum and
3.1.1 QUBIT SIGNAL AMPLIFIER
The active elements of lasers and optical amplifiers are examples of quantum amplifiers that use quantum mechanical methods to amplify a signal. One of the reasons for the use of digital signals in optical communications is the inevitable noise of quantum amplifiers, which can be deduced from quantum mechanics fundamentals. These are coded to a temperature of 4K towards the bottom, as we reach the actual chip the temperature drops to 0K or is in the order of few mK.
3.1.2. INPUT MICROWAVE LINES
This Protects the Qubits from thermal noise during the process of sending control and readout signals to the processor.
-
SUPER CONDUCTING COAXIAL LINES
. Super Conducting coaxial Lines Direct the Signal. In order to minimize energy loss, they are made up of super conductors.
-
CRYOGENIC ISOLATOR
Cryogenic Isolator operates at 4-8 GHz frequency range. They are designed to meet ultra-low temperature physics. They enable Qubit signals to go forward while preventing noise from Quantising Qubit Quality.
-
QUANTUM AMPLIFIERS
The amplification coefficient and uncertainty are the two most important properties of a quantum amplifier. The amplification coefficient and the uncertainty are not independent parameters; the higher the amplification coefficient, the higher the uncertainty (noise). In the case of lasers, the uncertainty relates to the active medium's enhanced spontaneous emission.
-
CRYOPERM SHIELD
The quantum processor bits inside a shield that protects it from the electromagnetic radiation in order to preserve the quality.
-
MIXING CHAMBER
Mixing Chamber provides the necessary cooling power to bring the processor and associated components down to a temperature of 15mK.
-
QUANTUM SUPREMACY
It is the point at which a quantum computer outperforms a traditional one. When you gradually increase the complexity of the circuit, at some point it becomes impossible for the classical computer to keep up with the quantum computer. Then we say we have achieved quantum supremacy. Google claimed to have achieved quantum supremacy in its Sycamore chip in Oct 2019. To demonstrate quantum supremacy, first we need to choose the Circuit then run it in on a Quantum Computer and finally simulate the same task on a classical computer as shown in Fig.6
Fig.6 Steps for Quantum Supremacy
-
CHALLENGES OF QUANTUM COMPUTING
-
We use superconductivity to create and maintain a quantum bit. Any heat in the system can introduce error, therefore quantum computers operate at temperatures close to absolute zero, colder than outer space. A huge infrastructure is required in order to maintain this very low temperature.
-
A quantum computer is highly sensitive to perturbations, noise, electrical interference and environmental effects. Almost anything can knock a quantum bit out of the delicate state of superposition. Quantum computers have high error rates because of those problems due to interference. Theyre powerful, but not reliable.
-
The construction of a quantum computer requires Helium-3, a nuclear research by-product, and special superconducting cables that are only made by the Japanese company Coax Co.
-
There exists a concept of decoherence- we can only keep the information in the quantum state for a particular time period. This puts a limit on the number of operations we can do in a row before you lose your information.
-
Quantum computers are still in its developmental stage. It will probably take many years before it can be used to solve real-world problems.
-
We still havent figured out the best tasks to give a quantum computer.
-
Quantum computers will one day change the world, but for now, its future remains uncertain.
-
-
-
REFERENCES
Jan 2006
[4] Richard P. Feynman, Simulating physics with computers, International Journal of Theoretical Physics, Vol. 21, Nos. 6/7, 1982. [5] Charles H. Benett, and David P. DiVincenzo, Quantum Information and computation, NATURE, Vo. 404, 16 March 2000.