
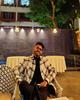
- Open Access
- Authors : Aschin Dhakad, Shruti Singh
- Paper ID : IJERTV12IS080080
- Volume & Issue : Volume 12, Issue 08 (August 2023)
- Published (First Online): 05-09-2023
- ISSN (Online) : 2278-0181
- Publisher Name : IJERT
- License:
This work is licensed under a Creative Commons Attribution 4.0 International License
Advancements in Multi-Port MIMO Antenna Systems for Wireless Communication
Aschin Dhakad
Electronics and Telecommunication Engineering, RV College of Engineering®, Bengaluru, India
Shruti Singh
Electronics and Telecommunication Engineering, RV College of Engineering®, Bengaluru, India
Abstract – This paper presents a comprehensive review of innovative multi-port Multiple-Input Multiple-Output (MIMO) antenna designs, as highlighted in referenced works spanning 2009 to 2017. The discussed designs address key challenges in wireless communication, including frequency reconfigurability, compactness, isolation improvement, and directional coverage. Notably, frequency and port reconfigurable MIMO slot antennas for WLAN applications offer adaptability across diverse frequency bands. Multiband MIMO antennas cater to indoor communication needs across GSM, DCS, and LTE standards, optimizing spectrum utilization. Dual-band multiport MIMO slot antennas focus on enhancing WLAN performance. Techniques such as metamaterial mushroom structures are explored to boost isolation in closely spaced antenna configurations. Compact planar endfire MIMO arrays and directional slot antennas demonstrate strategies for achieving high directivity. Additionally, wideband slot arrays are evaluated to enhance MIMO efficiency in indoor WLAN scenarios. The study also introduces a compact wideband MIMO antenna, showcasing innovations in form factor and bandwidth. In summary, this paper presents a consolidated overview of advancements in MIMO antenna systems, offering insights into their contributions to contemporary wireless communication requirementsThe first set of references introduces frequency and port reconfigurable MIMO slot antennas tailored for WLAN applications. These designs offer versatile performance by dynamically adapting to different frequency bands. Another group of works focuses on multiband MIMO antennas suitable for indoor applications across diverse standards like GSM, DCS, and LTE. These designs optimize spectrum utilization in constrained environments.
Keywords – Multiple-input Multiple-output (MIMO), Wireless Local Area Network (WLAN).
-
INTRODUCTION
The need for high-performance antenna systems has risen dramatically as a result of the unrelenting advancement of wireless communication technology. Multiple-Input Multiple- Output (MIMO) systems have come to light among the many antenna designs as a viable way to satisfy the needs of contemporary wireless applications. Multiple antennas are used by MIMO technology at the transmitter and receiver ends to increase spectral efficiency, data speeds, and overall system reliability. The issues given by bandwidth restrictions, compactness, isolation augmentation, and directional coverage have been addressed in this context by a wide variety of novel MIMO antenna designs.
The topic of MIMO antenna systems has seen a number of academic contributions, each concentrating on a different way to improve wireless communication. Notably, attention has been drawn to frequency and port reconfigurable MIMO slot antennas because of its versatility across several frequency bands [1]. The dynamic spectrum usage needs of contemporary communication protocols, including Wireless Local Area Networks (WLANs), are met by these antennas. In addition, multiband MIMO antennas have been developed to support GSM, DCS, and LTE standards for indoor wireless applications [2]. These solutions help to seamless indoor wireless communication by effectively exploiting the spectrum resources that are already available.The evolution of MIMO technology has also prompted the development of dual-band multiport MIMO slot antennas, optimizing performance in WLAN scenarios [3]. The quest for improved isolation between closely spaced antenna elements has led to the exploration of metamaterial mushroom structures [4]. These structures enhance isolation while maintaining compact antenna configurations. Similarly, the pursuit of compactness has resulted in the design of planar endfire MIMO arrays [5] and directional slot antennas [6], both offering high directivity while minimizing the physical footprint.
Wideband slot arrays have been looked into as a way to improve MIMO performance for indoor wireless spaces [9]. These arrays take care of WLANs' demand for reliable connectivity, especially in cramped indoor environments. One of the highlighted papers is a unique compact wideband MIMO antenna design that shows improvements in form factor and bandwidth usage [10]. This design has a small footprint yet a large operational bandwidth.
We provide a thorough analysis and comparative study of the above ground-breaking MIMO antenna designs in this research. We hope to provide insights into the expanding multi-port MIMO antenna landscape and their ability to address the shifting issues facing contemporary wireless communication systems by synthesizing the contributions from these references.
-
ANTENNA DESIGN
In an effort to improve wireless communication performance, a multi-band reconfigurable MIMO slot antenna is suggested, taking inspiration from the
developments described in the referred publications. By incorporating qualities including frequency agility, compactness, and better isolation, this antenna design strives to meet the expectations of contemporary wireless applications.
The Soltani et al. [1] suggested frequency and port reconfigurable MIMO slot antenna has an impact on the antenna structure. In order to meet the changing needs of wireless communication standards, this design enables versatility by enabling dynamic adaption to various frequency bands. To assure compatibility with GSM, DCS, and LTE indoor applications, the idea of multiband operation is further integrated, as shown in Yang et al.'s work [2]. Utilizing the spectrum in the best possible way improves communication effectiveness.
The dual-band multiport MIMO slot antenna concept developed by Soltani et al. [3] is utilized to guarantee dependable performance in WLAN settings. Improved variety and capacity, which are necessary for high-quality wireless communications, are achieved by the antenna by using several ports. Additionally, isolation augmentation is accomplished through the incorporation of metamaterial mushroom structures, as suggested by Zhai et al. [4], resulting in a decrease in interference and an improvement in system performance as a whole.
This design, which is inspired by Capobianco et al.'s [5] work, which presented a compact MIMO array of planar endfire antennas, takes into account compactness, a crucial factor in contemporary wireless devices. The suggested antenna enables effective space utilization without sacrificing performance by using small, planar parts.
Fig. 1. Geometry of the single antenna element
Figure 1 displays the suggested antenna element. It is printed (r = 3.38, tan = 0.0027) on a Rogers 4003C substrate. The antenna is 36 by 27 by 0.8 millimeters in dimension. A reflector, a straight metal strip serving as the director, and a meander dipole make up the antenna. Additionally, a parasitic strip is positioned in between the dipole and the reflector to enhance impedance matching. Antenna size is decreased by folding the driven dipole, which operates in half-wavelength mode. The concave parabolic reflector, which is used to achieve unidirectional radiation at the lower band, is also created to reduce the size of the antenna while keeping the same directivity as that of employing a conventional straight reflector. For the upper band, a metal
ectivity.
strip is introduced to improve impedance matching and dir
Fig. 2 Equivalent circuit model
A 50- coaxial cable supplies power to the antenna. The two arms of the meander dipole are connected to the inner and outer conductors of the coaxial cable, respectively. The corresponding circuit model is displayed in Fig. 2 to describe the operation on the basis that the dipole antenna is the source of coupling [11]. The characteristic impedance Zs is related to the width wp and the electrical length s is related to the length lp. To increase the coupling between the dipole and reflector, the strip might actually be thought of as a microstrip transmission line [12]. The driven dipole could therefore link more power to the reflector, increasing its output. The coupling could be modified to get the desired result by altering the distances d1 and d2.
In conclusion, the multi-band reconfigurable MIMO slot antenna design that has been proposed combines the concepts of frequency reconfigurability, multiband operation, improved isolation, and compactness, taking inspiration from the developments noted in the works cited. This cutting-edge antenna system has the ability to provide increased connection, spectrum efficiency, and overall performance in order to satisfy the changing requirements of wireless communication systems.
-
ANTENNA ARRAY DESIGN
The small antenna that is suggested has a wide bandwidth and strong directivity, making it ideal for MIMO array construction. There shouldn't be much signal correlation between the antenna elements of a suitable MIMO array. Therefore, it is important to position each element correctly in order to minimize coupling between antenna parts. Fig. 3 shows two possible arrangements for the directional antenna components. For comparison, the simulated S-parameters are also displayed in Fig. 4. Array-1's placement allowed for a compact geometry and a low mutual coupling, as shown in Fig. 4.
Fig. 3. Two antenna array designs
Fig. 4. Simulated S-parameter of Array-1 and Array-2.
For Array-1, the mutual coupling between adjacent and opposite elements is below -14 dB and -18 dB, but for Array-2, it is only below -11 dB and -10 dB. The mutual interaction between opposed elements is significantly decreased by the square loop structure of Array-1. Additionally, Array-1's overall dimensions are merely 85 by 85 millimeters (0.68 0 by 0.68 0
by 0.68 0).
Fig. 5. Simulated and measured S-parameters of the proposed MIMO array
-
RESULTS
The 3-D electromagnetic simulator ANSYS HFSS 15.0 from a commercial source is used for simulation. And Fig. 1 includes a list of the optimized settings. A MIMO antenna prototype is created and tested in order to validate the suggested design. Fig. 8 shows a fake snapshot of the scene. Using an Agilent N5230A network analyzer, the S-parameters are monitored. And in the anechoic chamber, the radiation pattern, gain, and efficiency are measured. Only one port's findings are shown because of the symmetrical structure.
-
S-Parameters
The simulated and measured S-parameters are displayed in Figure 5. To the central frequency of 2.64 GHz, the measured 10-dB impedance bandwidth is 23.9% (0.63 GHz, 2.32-2.95 GHz). As can be seen, the coupling |S12| for elements that are close to one another is less than -17 dB, while the coupling |S13| for elements that are far apart achieves better than -21 dB throughout the whole band. With the exception of a minor frequency shift in the lower band that may have been caused by fabrication tolerance, there is good agreement between the simulated and measured results. The measured outcomes suggest that the suggested antenna array satisfies the isolation and impedance matching requirements for WLAN, LTE 2600, and WiMAX MIMO applications.
Fig. 5. Simulated and measured S-parameters of the proposed MIMO array
-
Radiation Characteristics
Figure 6 displays the measured and modelled radiation patterns in the xoy-plane (E-plane) and yoz-plane (H-plane). This demonstrates that the directional beam may be produced within the band. The 3-dB beamwidths in xoy-plane and yoz-plane are 78° and 142°, respectively.
Fig. 6. Simulated and measured radiation pattern of the proposed MIMO array in
xoy-plane and yoz-plane: (a) 2.4 GHz, (b) 2.7 GHz, (c) 2.8 GHz.
At 2.4, 2.7, and 2.8 GHz, the antenna obtains an FBR of 17.4 dB, 12.5 dB, and 11.4 dB. Fig. 7(a) shows the measurement and plotting of the antenna's gain. At 2.5 GHz, 5.5 dBi of gain is seen to be the maximum gain, and the gain fluctuates between
3.0 and 5.5 dBi. Within operating bandwidth, a flat gain of more than 3.0 dBi is attained. Meanwhile, Fig. 7(a) shows antenna efficiency as well. The efficiency measured is better than 83% across the entire band, and the maximum efficiency is at 2.6 GHz, according to the graph, which also shows the measured efficiency.
Fig. 7. Simulated and measured results: (a) gain and efficiency, (b) ECC.
-
-
CONCLUSION
It is suggested to use a tiny, broadband planar MIMO antenna. The impedance matching of the single element is significantly
enhanced by the addition of a parasitic strip between the driven dipole and reflector. To address the low gain at upper band and increase the operational bandwidth, a metal director is also added. A four-element MIMO array is built to have a wide bandwidth, low correlation, and a small size by positioning the antenna element correctly. Due to the observed results, the low profile planar construction and tiny footprint of the MIMO array are ideal for many wireless portable device applications. The MIMO array has a compact size of 0.68 0 by 680 by 0.0064 0.
REFERENCES
[1] S. Soltani, P. Lotfi, and R. D. Murch, A port and frequency reconfigurable MIMO slot antenna for WLAN applications, IEEE Trans. Antennas Propag., vol. 64, no. 4, pp. 12091217, Apr. 2016. [2] Y. Yang, Q. Chu and C. Mao, "Multiband MIMO antenna for GSM, DCS, and LTE indoor applications," IEEE Antennas Wireless Propag. Lett., vol. 15, pp. 1573-1576, 2016. [3] S. Soltani, P. Lotfi and R. D. Murch, "A dual-band multiport MIMO slot antenna for WLAN applications," IEEE Antennas Wireless Propag. Lett., vol. 16, pp. 529-532, 2017. [4] G. Zhai, Z. N. Chen and X. Qing, "Enhanced isolation of a closely spaced four-element MIMO antenna system using metamaterial mushroom," IEEE Trans. Antennas Propag., vol. 63, no. 8, pp. 3362-3370, Aug. 2015. [5] A. D. Capobianco et al., A compact MIMO array of planar endfire antennas for WLAN applications, IEEE Trans. Antennas Propag., vol. 59, no. 9, pp. 34623465, Sep. 2011. [6] A. C. K. Mak, C. R. Rowell, and R. D. Murch, Low cost reconfigurable Landstorfer planar antenna array, IEEE Trans. Antennas Propag., vol. 57, no. 10, pp. 30513061, Oct. 2009. [7] S. S. Jehangir and M. S. Sharawi, "A single layer semi-ring slot Yagi- like MIMO antenna system with high front-to-back ratio," IEEE Trans. Antennas Propag., vol. 65, no. 2, pp. 937-942, Feb. 2017. [8] H. T. Hu, F. C. Chen and Q. X. Chu, "A compact directional slot antenna and its application in MIMO array," IEEE Trans. Antennas Propag., vol. 64, no. 12, pp. 5513-5517, Dec. 2016. [9] J. R. Costa, E. B. Lima, C. R. Medeiros, and C. A. Fernandes, Evaluation of a new wideband slot array for MIMO performance enhancement in indoor WLANs, IEEE Trans. Antennas Propag., vol. 59, no. 4, pp. 1200-1206, Apr. 2011. [10] A. Al-Rawi et al., A new compact wideband MIMO antenna-The double-sided tapered self-grounded monopole array, IEEE Trans. Antennas Propag., vol. 62, no. 6, pp. 33653369, Jun. 2014.