
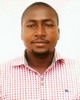
- Open Access
- Authors : Ibrahim A. Sukamari , Bitrus Kwaji , Jacob Alheri , Ichi-Osa Elvis Abia
- Paper ID : IJERTV9IS080335
- Volume & Issue : Volume 09, Issue 08 (August 2020)
- Published (First Online): 05-09-2020
- ISSN (Online) : 2278-0181
- Publisher Name : IJERT
- License:
This work is licensed under a Creative Commons Attribution 4.0 International License
Application of Water Quality Index to Assess the Potability of Some Domestic Water Supply Sources in Mubi North, Nigeria
Ibrahim A. Sukamari1*, Bitrus Kwaji2, Jacob Alheri1 and Ichi-Osa Elvis Abia3 1Depatment of Civil Engineering Technology, Federal Polytechnic Mubi 2Department of Geology and Mining Technology, Federal Polytechnic Mubi
3PG Student, Department of Civil Engineering, Federal University of Agriculture, Makurdi
Abstract:- This study set out to determine the potability of some common domestic water supply sources in Mubi North using Water Quality Index (WQI). Two major wards were selected (Barama/Gipalma and Barama) from where a total of seventeen (17) samples were collected monthly over a period of three months and analyzed for: temperature, turbidity, pH, total dissolved solids (TDS), electrical conductivity (EC), total hardness (TH), magnesium (+), calcium (+), nitrates (), sulphates (), phosphate (), chlorides (),
fresh water, but also to other environmental resources such as clean air, land and food. These resources would become even more difficult to access as population and the pollution it inevitably engenders impacts on the environment.
In order to guarantee public health, access to adequate water of good quality must be given to the people. However, with the current global economic challenges, governments have
dissolved oxygen (DO) and biochemical oxygen demand (BOD). Standards for drinking water quality as recommended by the World Health Organization (WHO) and Nigeria Industrial Standard (NIS) were adopted for reference. The weighted arithmetic water quality index (WAWQI) method was used to evaluate the WQI. Physicochemical analysis showed that the pH, TH, +, , , DO, and BOD for all samples in both Barama/Gipalma and Lokuwa met the limits of acceptability set by WHO and NIS. Turbidity and EC failed for open wells and river points in all locations, while TDS and
+ met the standards in boreholes and open wells but failed
in all river samples. values as analysed were all within permissible limit for all samples except river Mudzira which recorded a mean of 6.25 mg/L as against the 5 mg/L stipulated by WHO. The concentrations of in all samples failed the maximum permissible value stipulated by NIS except for the borehole in Barama/Gipalma. WQI determined that borehole waters from both Barama/Gipalma and Lokuwa are of good quality (WQI=45 and 31 respectively), open wells in Barama/Gipalma are of poor quality (WQI=70) while those in Lokuwa are of very poor quality (WQI=78). Finally, the river waters from both River Mudzira and Yedzaram were found to be unsuitable for drinking (WQI=123 and 116 respectively).
Key Words: Water quality index, drinking water quality, assessment of water quality, groundwater, surface water, Mubi.
INTRODUCTION
The rapid increase in population, urbanization, intense agricultural activities and accelerated industrialization in many parts of the world has put a tremendous burden on global fresh water supply (Abbasnia et al., 2019; Yisa, Jimoh, & Oyibo, 2012). With the worlds population projected to exceed nine billion by 2050 (United Nations, 2019), access to adequate fresh water supply for, especially domestic and agricultural purposes, will become more difficult except proper water resources management decisions and planning strategies are taken and implemented. In fact, the challenge is not just poor access to
been unable to put in place, and where available, unable to
maintain working municipal water supply systems in most developing countries of the world. The cost of installing these municipal water supply systems has prohibited their provision even by the governments of some economically well to do countries (Colombo & Karney, 2002). Consequently, the inability of the governments to provide adequate supplies of piped borne waters through municipality networks has forced the people to resort to obtaining supply mainly from simple traditional sources. These sources are typically open wells, boreholes, streams, ponds, rivers and direct collection from rain water.
Often, the waters collected from such sources are utilized with inadequate treatment or totally without treatment. Unfortunately, all of these sources are susceptible to pollution and contamination from both natural and anthropogenic influences.
For this reason, regular monitoring of the quality of the water resources of such areas are therefore absolutely necessary in order to protect environmental health. Hence, assessment and evaluation of the quality status of the major domestic water supply sources in Mubi becomes important.
Assessment of water quality has been established to be a complex process undertaking multiple parameters capable of causing various stresses on the overall quality (A. H. Fathy, F. Abdel Hamid, A. Shreadah, A. Mohamed, & G. El- Gazar, 2012). Traditionally, approaches to assessing water quality are based on the comparison of experimentally determined parameter values with existing guidelines. But it is always a difficult task to evaluate water quality from a large number of samples, each containing concentrations for many parameters. Therefore, Water Quality Index (WQI), a technique that would provide the composite influence of individual water quality parameters on the overall quality of
water for human consumption from a study involving a large number of samples was developed. It became an invaluable tool that could be used to minimize the data volume to a great extent and simplify the expression of water quality status (TirkeyPoonam, BhattacharyaTanushree, & ChakrabortySukalyan, 2015; Yisa et al., 2012).
WQI is defined as a rating that reflects the composite influence of different water quality parameters determined from the point of view of the suitability of water for human consumption (Chaurasia et al., 2018; Howladar, Numanbakth, & Faruque, 2017). It can be evaluated on the basis of various physical, chemical and bacteriological parameters, thus enhancing its versatility as a tool (TirkeyPoonam et al., 2015).
This study therefore seeks to employ the use of water quality index to assess the quality status of some physicochemical water quality parameters in some common sources of domestic water supply in Mubi using the WQI approach.
MATERIALS AND METHODS
DESCRIPTION OF THE STUDY AREA
Mubi is the capital of Mubi North and it is the largest town in the northern senatorial zone of Adamawa state, Nigeria. It is geographically situated on latitude 10°1130 to 10°2230 and longitude 13°1300 to 13°3000, covering a total land mass of 506.4 2 (Martins & Gadiga, 2015; Wante & Anoliefo, 2014). It lies on the West bank of the Yedzaram River and the western flanks of the Mandara Mountains. River Yedzeram flows northward from its source at the Hudu Hills south-east of Mubi, receiving flow from river Mudzira at Digil and draining into the Lake Chad after about 330 kilometers (Wante & Anoliefo, 2014 cited Adebayo, 2004). Mubi has a climate classified as Equatorial Savannah with dry winter or tropical rainy according to Koppens code (Kottek, Grieser, Beck, Rudolf, & Rubel, 2006). This climate is determined by the influence of inter tropical discontinuity (ITD) movement as well as the effect of relief. Maximum temperatures of up to 40°C can be recorded in April while minimum temperatures can be as low as 18°C between December and January. The mean monthly temperature ranges from 26.7°C to 27.8°C. Rainall begins in April, progressing and reaching its peak in August/September and stopping most of the time in October. Average annual rainfall ranges between 998 mm and 1262 mm. The cultural life of people in Mubi is linked to farming, animal raring, milling exploration and small scale industries (Wante & Anoliefo, 2014 cited Adeayo, 2004).
Table1: GPS locations of sampling points
Coordinates |
||||
Locations |
Sampling points |
Codes |
Latitude |
Longitude |
Barama/ Gipalma |
Borehole 1 |
BBH1 |
10.270488 |
13.301522 |
Borehole |
BBH2 |
10.269205 |
13.298991 |
|
Borehole |
BBH3 |
10.276201 |
13.293584 |
|
Open well |
BOW1 |
10.278305 |
13.295461 |
|
Open well |
BOW2 |
10.277556 |
13.293907 |
|
Open well |
BOW3 |
10.276995 |
13.294378 |
|
River point |
BRP1 |
– |
– |
|
River point |
BRP2 |
10.286049 |
13.309413 |
|
River point |
BRP3 |
10.282322 |
13.309412 |
Lokuwa |
Borehole |
LBH1 |
10.27381 |
13.282938 |
Borehole |
LBH2 |
10.273209 |
13.282474 |
|
Borehole |
LBH3 |
10.276923 |
13.282734 |
|
Open well |
LOW1 |
10.273431 |
13.283414 |
|
Open well |
LOW2 |
10.273525 |
13.282823 |
|
Open well |
LOW3 |
10.27632 |
13.282195 |
|
River point |
LRP1 |
10.263408 |
13.27941 |
|
River point |
LRP2 |
10.269754 |
13.276962 |
|
River point |
LRP3 |
10.274787 |
13.262688 |
SAMPLING AND SAMPLE PREPARATION
SELECTION OF SAMPLE SITES
A reconnaissance survey was conducted with the aim of identifying the common domestic water supply sources in Mubi North and areas with good representation of urbanization and agricultural activities. From the surveys conducted, two major wards in Mubi (Barama/Gipalma and Lokuwa) were selected for the study, from which seventeen
(17) sampling points were selected. Common domestic water supply sources identified in Mubi include open wells, boreholes and river points. The seventeen (17) sampling points earmarked were distributed such that every source identified and selected was apportioned three (3) samples each except for river Mudzira.
SAMPLE COLLECTION AND ANALYTICAL METHODOLOGY
Sampling was done monthly over a period of three (3) months from all seventeen (17) selected sampling points for all three common water supply sources identified. Simple grab samples from the rivers were collected 48 hours after a rain at commonly used water collection points. Open wells and the boreholes were purged so that the samples collected were representatives of groundwaters at the sampling locations. Purging of the boreholes was achieved using existing infrastructure where waters from the boreholes were pumped for a duration of twenty minutes before samples for analysis were collected. Purging and sampling of the open wells was achieved using a decontaminated bailer, such that waters from the open wells were collected and discarded until the wells were considered stabilized and ready for sampling. Sampling procedure followed the protocol described in Triplett et al (2006).
All water samples were collected into 1-liter capacity acid- cleaned High Density Polyethylene (HDPE) bottles with strict adherence to the sampling protocols described by Leo
M. L. Nollet (2007), Barcelona, (2014) and Standard methods (APHA, AWWA, & WEF, 2017) and analyzed independently. Samples for BOD analyses were collected in specialized glassware. Replicate samples were collected from each sampling location as prescribed in Triplett et al (2006).
On-site analyses of temperature was done using a Celsius thermometer; pH, electrical conductivity (EC) and dissolved oxygen (DO) were conducted using HACH® sensIon 156 Portable multi-parameter. Turbidity was measured using a HI 93703 portable microprocessor Turbidity meter (HANNA Instruments). Before measurements, all equipment were adequately calibrated according to standards and all reagents used were of analytical grade.
Water samples for laboratory analyses were collected with regard to protocols and transported to the Department of
Animal Production Laboratory of Adamawa State University (ADSU) Mubi. Samples for metal analysis were preserved with 3 ml concentrated HNO3 per liter in the field
Having done that, the relative weighting of each parameter was determined by computation using the formula:
to bring pH to less than 2 (Begum, Mondal, Ferdous, Zafar,
=
(3)
& Ali, 2014; David K. et al., 2011). The concentrations of
Where
unit weight of tested parameter
4
4
4
4
major anions (SO 2-, NO3 -, Cl- and PO 3-), total dissolved
=
= Standard value stipulated by an agency
solids (TDS), Total Hardness (TH) and BOD in water
samples were determined according to standard methods
= Constant of proportionality, determined by:
(APHA, AWWA, & WEF, 2017). The elemental
= 1
1
(4)
concentrations (i.e. for Mg2+, Ca2+) in water samples were determined by an Atomic Absorption Spectrophotometer
Further
more, the index for each parameter tested was
AAS (Buck Scientific, VPG 210) following procedure as reported by David K. et al., (2011).
COMPUTATION OF WATER QUALITY INDEX (WQI)
This study adopted the Weighted Arithmetic Water Quality Index (WAWQI) method because of its flexibility, in that it is suitable for use in assessing the quality for both groundwaters and surface waters (Ingale & Jadhal, 2017). In as much as the geometric aggregation method developed by Brown et al (1973) was believed to be better than the arithmetic aggregation because it was considered to be more sensitive when a single variable exceeds the norm; it has its disadvantage in that if the value of one of the variables is close to zero, whatever the weighting of the variables, the WQI will tend to 0 (Kachroud, Trolard, Kefi, & Jebari, 2019). Therefore, the WAWQI method has found more application by researchers (Ahmad, 2014; Imneisi & Aydin, 2016; Ingale & Jadhal, 2017; Oni & Fasakin, 2016; Ranawat, Singh, Chourasiya, & Bhatnagar, 2017; Tripathi & Shukla, 2017; Yogendra & Puttaiah, 2008). The method uses the most commonly measured water quality variables, such as temperature, pH, turbidity, dissolved oxygen, biochemical oxygen demand, total phosphates, nitrates etc. to classify the general water quality according to its degree of purity. For this study, thirteen (13) important water quality parameters were chosen and standards for drinking water quality as recommended by the World Health Organization (WHO) (WHO, 2017) and Nigeria Industrial Standard (NIS) (SON, 2007) were adopted for reference (Table 2). The computation of the WQI, using WAWQI method as proposed by Brown et al (1972) was implemented using the following equations:/p>
calculated from the product and summed up to obtain
from where, finally, the overall WQI was calculated by aggregating the quality rating with the unit weight linearly.
The rating and grading of water quality based on results obtained from the WAWQI method was done with reference to Table 2.
Table 2: water quality rating as per WQI
Water quality index level |
Water Quality status |
Grade |
0-25 |
Excellent water Quality |
A |
26-50 |
Good water Quality |
B |
51-75 |
Poor water Quality |
C |
76-100 |
Very poor water Quality |
D |
>100 |
Unsuitable for Drinking |
E |
Source: (Brown et al, 1972; Chatterjee & Raziuddin, 2007; Imneisi & Aydin, 2016; Tyagi, Sharma, Singh, & Dobhal, 2013)
Table 3: Drinking water quality standards for selected
Selected Parameters |
Recommending Agency |
Standards |
Relative weight Wn |
Turbidity |
WHO |
5.0 |
0.1931124 |
pH (mg/L) |
WHO |
6.5-8.5 |
0.12069525 |
TDS (mg/L) |
WHO/NIS |
500 |
0.001931124 |
EC |
WHO |
500 |
0.001931124 |
TH (mg/L) |
WHO |
500 |
0.001931124 |
Mg (mg/L) |
NIS |
20 |
0.0482781 |
Ca (mg/L) |
WHO |
75 |
0.01287416 |
Nitrates |
WHO/NIS |
50 |
0.01931124 |
Sulphate |
NIS |
100 |
0.00965562 |
Phosphate |
WHO |
5 |
0.1931124 |
Chloride |
WHO |
250 |
0.003862248 |
DO |
WHO |
>5 |
0.1931124 |
BOD |
WHO |
5 |
0.1931124 |
Selected Parameters |
Recommending Agency |
Standards |
Relative weight Wn |
Turbidity |
WHO |
5.0 |
0.1931124 |
pH (mg/L) |
WHO |
6.5-8.5 |
0.12069525 |
TDS (mg/L) |
WHO/NIS |
500 |
0.001931124 |
EC |
WHO |
500 |
0.001931124 |
TH (mg/L) |
WHO |
500 |
0.001931124 |
Mg (mg/L) |
NIS |
20 |
0.0482781 |
Ca (mg/L) |
WHO |
75 |
0.01287416 |
Nitrates |
WHO/NIS |
50 |
0.01931124 |
Sulphate |
NIS |
100 |
0.00965562 |
Phosphate |
WHO |
5 |
0.1931124 |
Chloride |
WHO |
250 |
0.003862248 |
DO |
WHO |
>5 |
0.1931124 |
BOD |
WHO |
5 |
0.1931124 |
parameters according to WHO and NIS
=
(1)
, the quality rating or sub index was calculated by:
= 100()
()
(2)
Where = Quality rating for the water quality parameter
= Estimated value of the parameter at a given sample station
= Standard permissible value for the parameter
= Ideal value for the parameter in pure water ( is zero for all parameters except for pH and dissolved oxygen which are 7.0 and 14.6 mg/L respectively).
For n water quality parameters, quality index or sub index
corresponding to parameter is a number reflecting the relative value of this parameter in the polluted water with respect to its standard value.
RESULTS AND DISCUSSION
The results of the physicochemical parameters for the different samples of borehole water, open well water and river points obtained from Barama/Gipalma and Lokuwa as analyzed are presented in Tables 4 and 5 respectively. The WHO guidelines (WHO, 2017) and the NIS guidelines (SON, 2007) were recognized and used as recommending agencies standard in the water quality analysis and in the computations of the WQIs (Table 3).
ANALYSIS OF PHYSICOCHEMICAL PARAMETER
Temperature
All mean temperature values recorded for all the sources sampled from both Barama/Gipalma and Lokuwa locations fall below the standard set for maximum permissible water temperature by WHO based on palatability and aesthetic objectives (WHO, 2017). Mean temperature values of 28.28, 27.40 and 28.00, were recorded for borehole, open well and river points respectively at Barama/Gipalma. Similarly, mean temperature values at Lokuwa for borehole, open well and River Yedzeram were recorded as 28.00, 27.95 and 28.05, respectively. Driscol (2002) reported that water temperatures generally increase with the depth of wells. This fact explains why the temperatures of the boreholes were higher than those of the open wells which are characteristically shallower. Temperature has been reported to affect the physical, chemical and biological nature of the water (Kale, 2016). Saito, Hamamoto, Ueki, Ohkubo, & Moldrup (2017) reported that a temperature increase of 7 can be responsible for changes in concentration of between 4% to 31% in magnesium, potassium, sodium and other important parameters in groundwater. Significant impacts induced on groundwater by increasing the temperature from 25.00, through to 60.00 has also been reported by Bonte et al (2013). They observed significant increase on pH, dissolved organic carbon (DOC), As, B, and F; with no consistent effects on Ca, Na, Fe, Mg, Cu, Zn and Mn.
The rivers are seasonal and hence they experience alternating flooding and droughts situations. Characteristically, flow rates in such rivers are usually high and does not facilitate the effects of high water temperatures on the concentration of DO and the amount of dissolved materials. The alternating flooding and droughts situations would however continually affect the water quality through dilution or concentration of dissolved substances respectively (Khatri & Tyagi, 2015; Prathumratana, Sthiannopkao, & Kim, 2008; Tian et al., 2019; van Vliet & Zwolsman, 2008).
The temperature values obtained in this study were reported in a similar study by Oyem et al (2014) and Meride & Ayenew (2016). Oyem et al., (2014) concluded that their values are consistent with temperatures prevailing and obtainable in the tropical belt.
Table 4: Mean values of water quality parameters from Barama/Gipalma and river Mudzira
parameters |
Borehole |
Open- well |
River |
Temperature, Temp.(oC) |
28.28 |
27.40 |
28.00 |
Turbidity, Turb. (NTU) |
3.36 |
6.72 |
13.75 |
pH |
7.76 |
8.20 |
7.88 |
210.62 |
371.15 |
579.82 |
|
Electrical Conductivity, EC (µS/ml) |
396.45 |
575.54 |
604.86 |
Total Hardness, TH (mg/l) |
101.55 |
159.90 |
159.26 |
Magnesium, Mg (mg/l) |
9.59 |
14.72 |
23.46 |
Calcium, Ca+ (mg/l) |
15.14 |
27.22 |
20.32 |
Nitrates, NO – (mg/l) 3 |
5.37 |
11.13 |
9.24 |
Sulphates, SO4 – (mg/l) 2 |
100.21 |
240.80 |
191.48 |
Phosphate. PO43- (mg/l) |
0.18 |
0.82 |
6.25 |
Chlorides, Cl- (mg/l) |
7.62 |
12.04 |
6.80 |
Dissolved Oxygen, DO (mg/ |
6.15 |
7.89 |
6.90 |
Biochemical Oxygen Dem BOD (mg/l) |
0.15 |
1.45 |
2.67 |
Table 5: Mean values of water quality parameters from Lokuwa and river Yedzaram
parameters |
Borehole |
Open- well |
River |
Temperature, Temp.(oC) |
28.00 |
27.95 |
28.05 |
Turbidity, Turb. (NTU) |
1.82 |
8.52 |
13.89 |
pH |
7.23 |
8.70 |
8.15 |
Total Dissolved Solid, TDS (mg/l) |
242.66 |
448.48 |
548.91 |
Electrical Conductivity, EC (µS/ml) |
432.22 |
591.02 |
620.30 |
Total Hardness, TH (mg/l) |
96.82 |
182.03 |
177.69 |
Magnesium, Mg (mg/l) |
8.23 |
13.24 |
24.17 |
Calcium, Ca+ (mg/l) |
9.84 |
21.76 |
21.65 |
Nitrates, NO – (mg/l) 3 |
6.59 |
12.95 |
11.44 |
Sulphates, SO4 – (mg/l) 2 |
91.03 |
198.60 |
150.94 |
Phosphate. PO43- (mg/l) |
0.20 |
0.22 |
3.37 |
Chlorides, Cl- (mg/l) |
9.19 |
12.86 |
6.96 |
Dissolved Oxygen, DO (mg/l |
6.76 |
7.85 |
6.88 |
Biochemical Oxygen Dem BOD (mg/l) |
0.16 |
0.88 |
2.65 |
Turbidity
Turbidity in water refers to its degree of cloudiness or clarity. It relates to the ability of water to allow or impede the penetration of light due to the scattering effect of suspended particulate matter in the water. These suspended particulate matter may include clay, silt, fine organic and inorganic matter, or microorganisms (Abba, Abubakar, & Bwade, 2016; World Health Organization, 2017). For groundwater supplies, if turbidity is greater than 1 NTU, it is important to determine whether there is a potential risk for such water to cause health problems. Consequently, groundwaters with a turbidity greater than 1 NTU should be sampled for bacteria and nitrate (World Health Organization, 2017). The WHO standard for turbidity concerning potability of water is a value of not more than 5 NTU. All boreholes samples analyzed for Barama/Gipalma and Lokuwa were observed to fall below the permissible maximum limit of 5 NTU (Tables 4 and 5). Barama/Gipalma boreholes recorded a mean turbidity of 3.36 NTU while Lokuwa boreholes recorded a mean turbidity value of 1.82 NTU. The mean turbidity values recorded for open wells in Barama/Giplama (6.72 NTU) and Lokuwa (8.52 NTU) and for Rivers Mudzira (13.75 NTU) and Yedzaram (13.89 NTU) did not meet the WHO/NIS standard for domestic water supply. Results obtained for turbidity in groundwater by this study is in agreement to Seli, Ankidawa, Ishaku, &
Aminu (2019) and Yada, Lami, Usman, Bulama, & Zira (2017).
pH
The pH of water is a measurement of the degree of acidity or alkalinity in the water; or a measure of the concentration of hydrogen ion in water. The pH of water has significant impact on health and the environment. A pH of less than 6.5 inhibits the human body from the intake of vitamins and minerals and when it exceeds 8.5 the water becomes caustic and irritating (Gupta, Pandey, & Hussain, 2017). The pH of the water samples analyzed are as recorded in Tables 4 and
500
400
300
200
100
0
500
400
300
200
100
0
600
600
400
400
200
200
Concentration (mg/L)
Concentration (mg/L)
Concentration (mg/L)
Concentration (mg/L)
5. All results fall within the acceptable limits for pH requirements as set by WHO. The mean pH for boreholes in Barama/Gipalma recorded 7.76 and that of boreholes in Lokuwa recorded 7.23; River Mudzira and Yedzaram recorded 7.80 and 8.15 respectively. Meanwhile, the open
wells samples recorded the highest mean values for pH with the open wells in Barama/Gipalma recording pH of 8.20 while those in Lokuwa recorded pH of 8.70 (Fig. 2). The results obtained in this study are in agreement to those obtained by Alexander (2008), Ishaku et al (2012), Yusuf & Alkali (2018) and Seli et al. (2019).
Total Dissolved Solids
The amount of solids present in a water body is an important indicator of pollution from both organic and inorganic contaminants. The higher the concentrations of solids in a given water the higher the degree of contamination irrespective of its source. Water containing high TDS is of inferior palatability and may produce unfavorable physiological reaction in the transient consumer (David K. et al., 2011; Kumar, Reddy, Jayaveera, & Pradesh, 2016).
Parameters
Parameters
Parameters
Parameters
Barama/G
Lokuwa
Barama/G
Lokuwa
Barama/G
Lokuwa
Barama/G
Lokuwa
0
0
Temp
Turb pH TDS EC TH
Mg Ca NO3 SO4 PO4
Cl
DO
BOD
Temp
Turb pH TDS EC TH
Mg Ca NO3 SO4 PO4
Cl
DO
BOD
Concentration (mg/L)
Concentration (mg/L)
Temp
Turb pH TDS EC TH
Mg Ca NO3 SO4 PO4
Cl DO BOD
Temp
Turb pH TDS EC TH
Mg Ca NO3 SO4 PO4
Cl DO BOD
Fig. 1: Comparison of parameter concentration in borehole Fig. 2: Comparison of parameter concentration in Samples from Barama/Gipalma and Lokuwa open well samples from Barama/Gipalma and Lokuwa
800
600
400
200
0
800
600
400
200
0
Parameters
Parameters
Barama/G
Lokuwa
Barama/G
Lokuwa
Fig. 3: Comparison of parameter concentration in river samples from Barama/Gipalma (Mudzira) and Lokuwa (Yedzaram)
Water with low TDS concentrations also tastes flat and insipid and therefore may also be unsuitable for drinking (Howladar et al., 2017). At TDS levels above 300 mg/L taste and odor is amplified and TDS become noticeable to consumers, and as the concentration increases, water becomes increasingly unacceptable. High levels of TDS may aesthetically be unsatisfactory for bathing and washing (APHA et al., 2017).
With reference to the results presented in Tables 4 and 5, the TDS values observed for the groundwater sources all fall within the acceptable limits for both WHO and NIS standards of less or equal to 500 mg/L for domestic supply. However, samples from the open wells having TDS values of 371.15 mg/L (Barama/Gipalma) and 448.48 mg/L (Lokuwa) ( Fig. 2) may not make the first choice for a domestic supply source in the availability of alternatives due to possible amplification of taste and odor. Both river samples did not meet the standard concentration allowable in domestic supplies as stipulated by both WHO and NIS. Barama/Gipalma samples recorded mean TDS value of
579.82 mg/L while Lokuwa samples recorded mean TDS value of 548.91 mg/L. Oni & Fasakin (2016) reported similar results for TDS in groundwater sampled in the vicinity of municipal wastes dumpsites in Ado Ekiti, Nigeria. Yada et al. (2017) reported very low values for TDS in their study while Yusuf & Alkali (2018) reported values greater than 1000 mg/L. Yusuf & Alkali (2018) attributed the high TDS values to the impact of the heavy agricultural activities taking place in the vicinity of the water they sampled.
Electrical Conductivity
EC is a measure to the capacity of water to conduct electrical current. Its value is directly proportional to the concentration of salts dissolved in the water and hence to the TDS (Meride & Ayenew, 2016). EC is also a function of the water temperature, that is, the higher the temperature, the higher the electrical conductivity would be. A temperature increase in a solution will cause a decrease in its viscosity with a consequent increase in the mobility of the ions in it (Barron & Ashton, 2007). Additionally, an increase in temperature may also cause an increase in the number of ions in solution due to dissociation of molecules. Consequently, a temperature rise of one degree Celsius in water may trigger a 2-3% increase in the EC of that water (Idiata, 2015). EC is closely related to the salinity in water and thus it is considered a good indicator of total salinity in water (Idiata, 2015; Rusydi, 2018).
With reference to Tables 4 and 5, EC values that are acceptable with respect to the WHO standard are for the boreholes in Barama/Giplama and Lokuwa. These sources recorded mean values of 396.45 mg/L and 432.22 mg/L respectively. The open wells and the river points all recorded mean values beyond the limit of acceptance as presented in Table 3. Interestingly, Yusuf & Alkali (2018) reported similar values for EC.
Total Hardness
Total hardness in water is primarily due to the excess of Ca, Mg and Fe salts (Bairu, Tadesse, & Amare, 2013). It is an important water quality parameter whether the water is intended for domestic, industrial or agricultural purpose. Hard water has been linked to, in addition to impacts stated by Guillemant et al. (2000), have tendency to influence mortality, in particular, cardiovascular mortality in addition to reproductive failure, cancer and Alzheimers disease (Sengupta, 2013). Total hardness recorded for this study in all groundwater (boreholes and open wells) and surface water (rivers) all lie within the permissible limits of 500 mg/L (as CaCO3) as per WHO standards. These results are consistent with similar studies conducted around Mubi (Alexander, 2008; Ishaku, Kaigama, & Onyeka, 2011).
Magnesium and Calcium
The desirable limits for magnesium and calcium for drinking water is 20 mg/L and 75 mg/L respectively according to NIS and WHO standards. Analyses on samples collected from all locations and from the three sources considered in this study reveal that all groundwater sources (boreholes and open wells) are acceptable for domestic use as per the standards concerned; while surface water samples failed for magnesium in all locations but are acceptable for calcium in all locations (Tables 4 and 5). These values are consistent with those reported by Yada et al. (2017) for calcium and Yonnana, Yamta, Kaigama, & Bedeson (2017) for magnesium. Calcium rich water is recommendable for drinking several times a day because it has the capacity to provide supplemental calcium and aids adequate hydration, however, it has an inhibitory effect on parathyroid hormone secretion and bone resorption causing stunted growth (Cotruvo J, Bartram J, 2009). Guillemant et al. (2000) and Sengupta (2013) also reported on the ability of excess calcium concentrations to retard growth. The presence of calcium and magnesium in groundwater is linked to the leaching of geologic materials such as limestone, dolomites, gypsum and anhydrites; although calcium ions are also derived from cation exchange process (Alaya, Saidi, Zemni, & Zargouni, 2014).
Nitrates
The mean nitrate values for all samples were observed to meet the permissible limits of 50 mg/L set by WHO and NIS (Tables 4 and 5). However, open wells samples recorded higher concentrations of nitrates (11.13 mg/L and 12.95 mg/L for Barama/Gipalma and Lokuwa respectively). Such high concentration of nitrates in water is a cause for concern as a nitrate concentrations of 10 mg/L and above poses a health threat to infants (Sharma & Kaur, 2017). Ward et al. (2018) reported that the risk of specific cancers (colorectal cancer, bladder and breast cancers) and birth defects is increased when nitrate is ingested under conditions that increase formation of N-nitroso compounds. Conversely however, some recent scientific literatures indicate that high nitrates concentrations do actually have some beneficial effects on human health even though it remains an undesirable component because it is often accompanied by more dangerous constituents such as microorganisms and
pesticides (DAlessandro et al., 2012; LHirondel & LHirondel Jean-Louis, 2001). High concentrations of nitrate in water may indicate a possibility of anthropogenic impacts such as usage of nitrogen based fertilizers, human- animal bodily wastes, and disposal of wastes in proximity to the sampling locations (DAlessandro et al., 2012). The presence of nitrates in water samples is an indication of some bacterial and other biological activities (Elahcene, El-Azim, & Aidoud, 2019). This is the reason why World Health Organization (2017) advices sampling and investigation into nitrates and bacteria as suspects for turbidity higher than 1 NTU in groundwater.
Sulphates
High values of sulphates concentrations above the standard of 100 mg/L (NIS) were observed for all locations and for all sources of domestic supplies sampled except the boreholes in Lokuwa. Sulphates are naturally occurring in water due to leaching of gypsum, barite, epsom and the dissolution of salts of sulfuric acid (Elahcene et al., 2019; Sharma & Kaur, 2017). High concentrations of sulphate may result from the oxidation of pyrite and mine drainage and from human influences such as discharge of industrial wastes and domestic sewage (Gulumbe, Aliyu, & Manga, 2016). High concentrations of sulphate has been linked to some minor health concerns such as catharsis, dehydration and gastrointestinal irritation (Gulumbe et al., 2016). Other health concerns raised by high concentrations of sulphate as reportedly found in literature by Sharma & Kaur (2017) include: renal failure and hyperthyroidism.
Phosphates
Surface waters and groundwaters become contaminated from both natural and anthropogenic sources of phosphates. Natural sources of phosphorus in both surface and groundwater include atmospheric deposition, natural decomposition of rocks and minerals, weathering of soluble inorganic materials, decaying biomass, runoff, and sedimentation. Anthropogenic sources include; fertilizers, wastewater and septic system effluent, animal wastes, detergents, industrial discharge, phosphate mining, drinking water treatment, forest fires, synthetic material development surface (Fadiran, Dlamini, & Mavuso, 2008; Nemati Varnosfaderany, Mirghaffary, Ebrahimi, & Soffianian, 2009). Phosphate is sorbed strongly onto solid phases, including Fe and Al oxides in soils, and P concentrations in recharging groundwater generally do not reflect the large amouns of P applied to agricultural fields (Böhlke, 2002). Borehole and open wells samples from Barama/Gipalma analyzed for phosphate recorded concentrations of 0.18 mg/L and 0.82 mg/L respectively. Both values are far below the maximum permissible concentration of 5 mg/L prescribed by the WHO standard. On the other hand, the mean value obtained for the River Mudzira was 6.25 mg/L, exceeding the maximum permissible limit for phosphate in drinking water as stipulated by the WHO standard. All samples analyzed for Lokuwa locations meet the standard of less than 5 mg/L concentration of phosphate. The mean values are 0.20 mg/L, 0.22 mg/L and 3.37 mg/L for borehole, open wells and River Yedzaram respectively.
Phosphates do not have any significant impact on human health when they are naturally occurring in water however, digestive problems, muscle damage, problem with breathing and kidney failure have been reported when high level of phosphates are consumed in drinking water (Fadiran et al., 2008; Gupta et al., 2017; Nyamangara, Jeke, & Rurinda, 2013).
Chlorides
Chloride is one of the major constituents of natural water and one of the most important parameter in assessing the water quality (Yogendra & Puttaiah, 2008). Very high concentrations of chlorides detected in water are commonly considered as important index of pollution and are therefore employed as tracers for groundwater contamination (DAlessandro et al., 2012; Mahato, Mahato, Karna, & Balmiki, 2018). This study recorded very low values for chloride concentrations that are far below the maximum permissible concentration allowed by the WHO standard of 250 mg/L. with reference to Table 4 and Fig 1, 2 and 3, it can be seen that the mean chloride concentrations recorded for boreholes, open wells and river points on River Mudzira are 7.62 mg/L, 12.04 mg/L and 6.80 mg/L respectively. Similarly for Lokuwa locations, boreholes, open wells and river points on Yedzeram recorded chloride concentrations of 9.19 mg/L, 12.86 mg/L and 6.96 mg/L respectively. Chloride concentrations in groundwater may result from saline water intrusion, application of deicers, agricultural activities, domestic wastewater, industrial chemical, landfill leachates etc. (Batabyal & Chakraborty, 2015; Ishaku, 2011). Considering results presented in Table 4 and 5, for both locations, the concentrations of chloride was higher in the open wells than those in the rivers. These values may be as a result of pollution from anthropogenic sources like agricultural chemicals, or leachates from pit latrines situated approximately to the open wells (Mahato et al., 2018). The relatively lower concentrations of chloride in the river samples may be due to dilution effect (Nyamangara et al., 2013). Yusuf & Alkali (2018) carried out a study on groundwater variation across a space in Mubi and obtained chloride concentrations in the groundwater in the range of 2 mg/L to 27 mg/L.
Dissolved Oxygen and Biochemical Oxygen Demand Results obtained for DO for all samples and from all sources for all locations were above the minimum required DO concentrations in drinking water as set by WHO. Similarly, the results obtained for BOD for all samples analyzed fall below the maximum permissible limit stipulated by WHO. However, considering the results presented in Tables 4 and 5 and illustrated in Fig 2 and 3, it can be seen that the mean concentrations of DO in open wells from both Barama/Gipalma and Lokuwa (7.89 mg/L and 7.85 mg/L respectively) are higher than the DO concentrations in Rivers Mudzira and Yedzaram (6.90 mg/L and 6.88 mg/L) even though surface waters are prone to more oxygenation. BOD is a function of the amount of organic matter present in a particular water environment. Hence the value of BOD is an indication of the amount of oxygen required by microorganisms present in the water environment to
decompose the organic matter in it. Consequently, a higher BOD value would indicate a higher amount of polluting biodegradables in water which invariably would require higher amounts of DO for its stabilization. On the other hand, low levels of BOD will indicate low levels of biodegradable materials and hence higher amounts of DO may be available in the water environment. This may explain the inverse relationship exhibited in the values for DO and BOD (Fig 2 and 3) for the open wells sources and the river sources. The mean BOD values recorded were 1.45 mg/L for open wells in Barama/Gipalma and 0.88 mg/L for open wells in Lokuwa. DO values were 7.89 mg/L and 7.85 mg/L for open wells in Barama/Gipalma and Lokuwa respectively. Similarly, the results obtained for the surface waters are: BOD: 2.67 and 2.65 mg/L for river samples in Barama/Gipalma and Lokuwa respectively; DO: 6.90 and
-
mg/L for the respective locations.
WATER QUALITY INDEX
This study considered 13 important water quality parameters to establish the water quality index for the sources and locations sampled within Mubi. These parameters were selected to reflect the common activities around the study area and the possible pollution indicators from such. Parameters include: Turbidity, pH, TDS, EC, TH, Mg, Ca, Nitrates, Sulphates, Phosphate, Chlorides, DO and BOD.
The results obtained for the WQI from the different sources are as presented in Tables 6 to 11 and summarized in Fig. 4. Similarly, the water quality and the grades for all locations are summarized in Table 12.
The results of WQIs computed for the borehole sources showed that on average, boreholes waters from the two locations (Barama/Gipalma and Lokuwa) are of good quality. The values obtained fall within the range of WQI level of 26-50 and grade B (Table 2); describing drinking water of good quality. However, boreholes from Lokuwa have a better quality rating (WQI=31) than Barama (WQI=45) with reference to Figure 4. This result may be attributed to the higher values recorded in samples from Barama/Gipalma for Turbidity, TH, 2+, pH, 2+ and
4
4
2 over Lokuwa samples as illustrated in Fig. 1.
For the open wells samples, results obtained from the computation of the WQIs were 70 and 78 for Barama/Gipalma and Lokuwa respectively.
Table 6: computed WQI for borehole from Barama/Gipalma
BARAMA/GIPALMA BOREHOLE WATER WQI
Parameters
Observed Vn
Standard Sn
Ideal value Vio
Quality rating Qn
Unit weight Wn
QnWn
Turb.(NTU)
3.36
5
0
67.2
0.1931124
12.97715
pH
7.76
8
7
76
0.12069525
9.172839
TDS (mg/l)
210.62
500
0
42.124
0.00193112
0.081347
EC (µS/ml)
396.45
500
0
79.29
0.00193112
0.153119
TH (mg/l)
101.55
500
0
20.31
0.00193112
0.039221
Mg (mg/l)
9.59
20
0
47.95
0.0482781
2.314935
Ca (mg/l)
15.14
75
0
20.1866667
0.01287416
0.259886
NO3 (mg/l)
5.37
50
0
10.74
0.01931124
0.207403
SO4 (mg/l)
100.21
100
0
100.21
0.00965562
0.96759
PO4 (mg/L)
0.18
5
<>0 3.6
0.1931124
0.695205
Cl- (mg/l)
7.62
250
0
3.048
0.00386225
0.011772
DO (mg/l)
6.15
5
14.6
88.0208333
0.1931124
16.99791
BOD (mg/l)
0.15
5
0
3
0.1931124
0.579337
Wn=0.99291959
QnWn=44.45772
WQI = QnWn/ Wn = 44.45772/0.99291959 = 44.77474
Table 7: computed WQI for open wells from Barama/Gipalma
BARAMA/GIPALMA OPEN-WELL WATER WQI
Parameters
Observed Vn
Standard Sn
Ideal value Vio
Quality rating Qn
Unit weight Wn
QnWn
Turb.(NTU)
6.72
5
0
134.4
0.1931124
25.95431
pH
8.2
8
7
120
0.12069525
14.48343
TDS (mg/l)
371.15
500
0
74.23
0.00193112
0.143347
EC (µS/ml)
575.54
500
0
115.108
0.00193112
0.222288
TH (mg/l)
159.9
500
0
31.98
0.00193112
0.061757
Mg (mg/l)
14.72
20
0
73.6
0.0482781
3.553268
Ca (mg/l)
27.22
75
0
36.29333
0.01287416
0.467246
NO3 (mg/l)
11.13
50
0
22.26
0.01931124
0.429868
SO4 (mg/l)
240.8
100
0
240.8
0.00965562
2.325073
PO4 (mg/L)
0.82
5
0
16.4
0.1931124
3.167043
Cl (mg/l)
12.04
250
0
4.816
0.00386225
0.018601
DO (mg/l)
7.89
5
14.6
69.8958333
0.1931124
13.49775
BOD (mg/l)
1.45
5
0
29
0.1931124
5.60026
Wn=0.99291959
QnWn=69.9242
WQI = QnWn/ Wn = 69.92424/0.99291959 = 70.42286
Table 8: computed WQI for river Mudzira passing adjacent Barama/Gipalma
RIVER MUDZIRA WATER WQI
Parameters
Observed Vn
Standard Sn
Ideal value Vio
Quality rating Qn
Unit weight Wn
QnWn
Turb.(NTU)
13.75
5
0
275
0.1931124
53.10591
pH
7.88
8
7
88
0.1206953
10.62118
TDS (mg/l)
579.82
500
0
115.964
0.0019311
0.223941
EC (µS/ml)
604.86
500
0
120.972
0.0019311
0.233612
TH (mg/l)
159.26
500
0
31.852
0.0019311
0.06151
Mg (mg/l)
23.46
20
0
117.3
0.0482781
5.663021
Ca (mg/l)
20.32
75
0
27.09333
0.0128742
0.348804
NO3 (mg/l)
9.24
50
0
18.48
0.0193112
0.356872
SO4 (mg/l)
191.48
100
0
191.48
0.0096556
1.848858
PO4 (mg/L)
6.25
5
0
125
0.1931124
24.13905
Cl (mg/l)
6.8
250
0
2.72
0.0038622
0.010505
DO (mg/l)
6.9
5
14.6
80.20833
0.1931124
15.48922
BOD (mg/l)
2.67
5
0
53.4
0.1931124
10.3122
Wn=0.9929196
QnWn=122.4147
WQI = QnWn/ Wn = 122.4147/0.99291959 = 123.2876
Table 9: computed WQI for borehole from Lokuwa
LOKUWA BOREHOLE WATER WQI
Parameters
Observed Vn
Standard Sn
Ideal value Vio
Quality rating Qn
Unit weight W
QnWn
Turb.(NTU)
1.82
5
0
36.4
0.193112
7.029291
pH
7.23
8
7
23
0.120695
2.775991
TDS (mg/l)
242.66
500
0
48.532
0.001931
0.093721
EC (µS/ml)
432.22
500
0
86.444
0.001931
0.166934
TH (mg/l)
96.82
500
0
19.364
0.001931
0.037394
Mg (mg/l)
8.23
20
0
41.15
0.048278
1.986644
Ca (mg/l)
9.84
75
0
13.12
0.012874
0.168909
NO3 (mg/l)
6.59
50
0
13.18
0.019311
0.254522
SO4 (mg/l)
91.03
100
0
91.03
0.009656
0.878951
PO4 (mg/L)
0.2
5
0
4
0.193112
0.77245
Cl (mg/l)
9.19
250
0
3.676
0.003862
0.014198
DO (m/l)
6.76
5
14.6
81.66667
0.193112
15.77085
BOD (mg/l)
0.16
5
0
3.2
0.193112
0.61796
Wn=0.992920
QnWn=30.5678
WQI = QnWn/ Wn = 30.56781/0.99291959 = 30.78579
Table 10: computed WQI for open wells from Lokuwa
LOKUWA OPEN-WELL WATER WQI
Parameters
Observed Vn
Standard Sn
Ideal value Vio
Quality rating Qn
Unit weight Wn
QnWn
Turb.(NTU)
8.52
5
0
170.4
0.1931124
32.90635
pH
8.7
8
7
170
0.12069525
20.51819
TDS (mg/l)
448.48
500
0
89.696
0.001931124
0.173214
EC (µS/ml)
591.02
500
0
118.204
0.001931124
0.228267
TH (mg/l)
182.03
500
0
36.406
0.001931124
0.070305
Mg (mg/l)
13.24
20
0
66.2
0.0482781
3.19601
Ca (mg/l)
21.76
75
0
29.01333333
0.01287416
0.373522
NO3 (mg/l)
12.95
50
0
25.9
0.01931124
0.500161
SO4 (mg/l)
198.6
100
0
198.6
0.00965562
1.917606
PO4 (mg/L)
0.22
5
0
4.4
0.1931124
0.849695
Cl (mg/l)
12.86
250
0
5.144
0.003862248
0.019867
DO (mg/l)
7.85
5
14.6
70.3125
0.1931124
13.57822
BOD (mg/l)
0.88
5
0
17.6
0.1931124
3.398778
Wn=0.99291959
QnWn=77.73019
WQI = QnWn/ Wn = 77.73019/0.99291959= 78.28447
Table 11: computed WQI for river Yedzaram passing by Lokuwa
RIVER YEDZERAM WATER WQI
Parameters
Observed Vn
Standard Sn
Ideal value Vio
Quality rating Qn
Unit weight Wn
QnWn
Turb.(NTU)
13.89
5
0
277.8
0.1931124
53.64662
pH
8.15
8
7
115
0.12069525
13.87995
TDS (mg/l)
548.91
500
0
109.782
0.001931124
0.212003
EC (µS/ml)
620.3
500
0
124.06
0.001931124
0.239575
TH (mg/l)
177.69
500
0
35.538
0.001931124
0.068628
Mg (mg/l)
24.17
20
0
120.85
0.0482781
5.834408
Ca (mg/l)
21.65
75
0
28.86666667
0.01287416
0.371634
NO3 (mg/l)
11.44
50
0
22.88
0.01931124
0.441841
SO4 (mg/l)
150.94
100
0
150.94
0.00965562
1.457419
PO4 (mg/L)
3.37
5
0
67.4
0.1931124
13.01578
Cl (mg/l)
6.96
250
0
2.784
0.003862248
0.010752
DO (mg/l)
6.88
5
14.6
80.41666667
0.1931124
15.52946
BOD (mg/l)
2.65
5
0
53
0.1931124
10.23496
Wn=0.99291959
QnWn=114.943
WQI = QnWn/ Wn = 114.943/0.99291959= 115.7627
45
45
70
70
123
123
31
31
78
78
116
116
BBH B O W BRP L B H L O W L R P B A R A M A / G L O K U W A
Fig. 4: Summary of WQI for all sources and locations
Table 12: Summary of computed WQIs for Barama/Gipalma and Lokuwa
Location
Sample Type
WQI
Water Quality Status
Grade
Barama/ Gipalma
BBH
45
Good water Quality
B
BOW
70
Poor water Quality
C
BRP
123
Unsuitable for Drinking
E
Lokuwa
LBH
31
Good water Quality
B
LOW
78
Very poor water Quality
D
LRP
116
Unsuitable for Drinking
E
3
3
These results fall within the ranges of 51-75 and 76-100 for Barama/Gipalma and Lokuwa respectively. These therefore describe that the water quality statuses for Barama/Gipalma and Lokuwa are: Poor water quality (grade C) and very poor water quality (grade D) respectively. The high concentrations for , , pH, Turbidity, TDS, EC and TH could be responsible for the the higher WQI obtained for the open wells in Lokuwa as seen from Fig. 2.
WQIs results obtained for the river points sampled from the river Yedzaram along its reach within Lokuwa (WQI=123) and River Mudzira within its reach along Barama/Gipalma (WQI=116) indicate that the river reaches considered are having waters unsuitable for drinking purpose. The river points for both Barama/Gipalma and Lokuwa have a grading of E, the least possible for WAWQI method.
CONCLUSION
The quality and suitability of some domestic water supply sources in Mubi North were determined through physicochemical analysis and WQI computations. Physicochemical analysis showed that the pH, TH, Calcium, Nitrates, chloride, DO, and BOD fo all samples from all sources in both Barama/Gipalma and Lokuwa met the limits of acceptability by WHO and NIS. Turbidity and EC failed for open wells and river points in all locations, while TDS and magnesium met the standards in boreholes and open wells but failed in all river samples. Phosphate values as analyzed were all within permissible limit for all samples except river Mudzira which recorded a mean of 6.25 mg/L as against the 5 mg/L stipulated by WHO. The concentrations of sulphates in all samples failed the maximum permissible value stipulated by NIS except for the borehole in Barama/Gipalma.
Based on the WQI computations, the water quality and suitability of sources based on the WAWQI are: good water quality for BBH and LBH both having grade B; poor water quality for BOW grade C; very poor water quality for LOW grade D; and unsuitable for drinking grade E for both river points. Even though most of the parameters tested were found to fall within permissible limits of concentrations based on the standards agencies
adopted, however, the Weighted Arithmetic Water Quality Index computations as summarized on Table 12 and illustrated on Fig. 4 indicate on average a poor water quality status for the study area. This outcome is understandably possible since the combined effects of the tested parameters were taken into consideration by the WQI model employed.
REFERENCES
-
A. H. Fathy, S., F. Abdel Hamid, F., A. Shreadah, M., A. Mohamed, L., & G. El-Gazar, M. (2012). Application of Principal Component Analysis for Developing Water Quality Index for Selected Coastal Areas of Alexandria Egypt. Resources and Environment, 2(6), 297305.
https://doi.org/10.5923/j. re. 20120206.08
-
Abba, M. U., Abubakar, M. S., & Bwade, E. K. (2016). Water Quality Assessment of Hand Dug-Wells and Treatment with (Moringa Oleifera) Powder in Mubi, Adamawa State, Nigeria. International Journal of Innovative Research in Technology, Basic and Applied Sciences, 3(1), 920.
-
Abbasnia, A., Yousefi, N., Mahvi, A. H., Nabizadeh, R., Radfard, M., Yousefi, M., & Alimohammadi, M. (2019). Evaluation of groundwater quality using water quality index and its suitability for assessing water for drinking and irrigation purposes: Case study of Sistan and Baluchistan province (Iran). Human and Ecological Risk Assessment, 25(4), 9881005. https://doi.org/10.1080/10807039.2018.1458596
-
Adebayo, A.A. (2004), Soil and vegetation, In: Adebayo, A. A (ed). Mubi Region: A Geographical Synthesis. A Division of Paraclete and Sons, Yola-Nigeria, pp. 38-43.
-
Ahmad, A. B. (2014). Evaluation of Groundwater Quality Index for drinking purpose from some villages around Darbandikhan district, Kurdistan Region -Iraq. IOSR Journal of Agriculture and Veterinary Science, 7(9), 3441.
https://doi.org/10.9790/2380-07913441
-
Alaya, M. Ben, Saidi, S., Zemni, T., & Zargouni, F. (2014). Suitability assessment of deep groundwater for drinking and irrigation use in the Djeffara aquifers (Northern Gabes, south- eastern Tunisia). Environmental Earth Sciences, 71(8), 3387 3421. https://doi.org/10.1007/s12665-013-2729-9
-
Alexander, P. (2008). Evaluation of ground water quality of Mubi town in Adamawa State, Nigeria. African Journal of Biotechnology, 7(11), 17121715. https://doi.org/10.5897/ajb08.227
-
APHA, AWWA, & WEF. (2017). Standard Methods for the Examination of Water and Wastewater. (R. B. Baird;, Andrew
D. Eaton;, & Eugene W. Rice, Eds.), American Public Health Association, American Water Works Association, Water Environment Federation.
-
Bairu, A., Tadesse, N., & Amare, S. (2013). Use of geographic information system and water quality index to assess suitability of groundwater quality for drinking purposes in Hewane areas, Tigray, Northern Ethiopia. Ethiopian Journal of Environmental Studies and Management, 6(2), 110123.
https://doi.org/10.4314/ejesm.v6i2.1
-
Barcelona, M. J. (2014). A Practical Guide for Ground-Water Sampling Practical Guide For Ground-Water Sampling, (January 1985).
-
Barron, A. J. J., & Ashton, C. (2007). The Effect of Temperature on Conductivity Measurement.
-
Batabyal, A. K., & Chakraborty, S. (2015). Hydrogeochemistry and Water Quality Index in the Assessment of Groundwater Quality for Drinking Uses. Water Environment Research, 87(7), 607617. https://doi.org/10.2175/106143015×14212658613956
-
Begum, A., Mondal, S., Ferdous, Z., Zafar, M. A., & Ali, M. M. (2014). Impact of water quality parameters on monosex tilapia
(. Int. j. Anim. Fish. Sci, 2(1), 1421.
-
Böhlke, J. (2002). Groundwater recharge and agricultural contamination. Hydrogeology Journal, 10, 153179. https://doi.org/10.1007/s10040-001-0183-3
-
Bonte, M., van Breukelen, B. M., & Stuyfzand, P. J. (2013). Temperature-induced impacts on groundwater quality and arsenic mobility in anoxic aquifer sediments used for both drinking water and shallow geothermal energy production. Water Research, 47(14), 50885100. https://doi.org/10.1016/j.watres.2013.05.049
-
Brown, R. M., N. I. McClelland, R. A. Deininger and M. F. OConnor, (1972) Water Quality Index-Crashing, the Psychological Barrier, Proc. 6th Annual Conference, Advances in Water Pollution Research, , pp 787-794.
-
Chatterjee, P. R., & Raziuddin, M. (2007). Studies on the water quality of a water body at asansol. Nature Environment and Pollution Technology ©, 6(2), 289292.
-
Chaurasia, A. K., Pandey, H. K., Tiwari, S. K., Prakash, R., Pandey, P., & Ram, A. (2018). Groundwater Quality assessment using Water Quality Index ( WQI ) in parts of Varanasi District
, Uttar Pradesh , India. Journal Geological Society Of India, 92(July), 7682. https://doi.org/10.1007/s12594-018-0955-1
-
Colombo, A. F., & Karney, B. W. (2002). Energy and Costs of Leaky Pipes: Toward Comprehensive Picture. Journal Of Water Resources Planning And Management, 128(December), 441450.
-
Colombo, A. F., Karney, B. W., & Asce, M. (2014). Energy and Costs of Leaky Pipes: Toward Comprehensive Picture Energy and Costs of Leaky Pipes: Toward Comprehensive Picture. Journal of Water Resources Planning and Management, 9496(July), 303306. https://doi.org/10.1061/ (ASCE) 0733- 949 6(2002)128
-
Cotruvo J, Bartram J, E. (2009). Calcium and Magnesium in Drinking-water – Public Health Significance. Geneva, World Health Organization. Retrieved from http://apps.who.int/iris/bitstream/handle/10665/43836/9789241 563550_eng.pdf;jsessionid=d351abbe791c3a84aaf8c2bd7dd12 c2d?sequence=1
-
DAlessandro, W., Bellomo, S., Parello, F., Bonfanti, P., Brusca, L., Longo, M., & Maugeri, R. (2012). Nitrate, sulphate and chloride contents in public drinking water supplies in Sicily, Italy. Environmental Monitoring and Assessment (Vol. 184). https://doi.org/10.1007/s10661-011-2155-y
-
David K., E., Senu, J., Fianko, J. R., Nyarko, B. K., Adokoh, C. K., & Boamponsem, L. (2011). Groundwater Quality Assessment: A Physicochemical Properties of Drinking Water in Rural Setting of Developing Countirs. Canadian Journal on Scientific and Industrial Research, 2(4), 171180.
-
Driscol, D. G. (2002). Hydrology of the Black Hills Area, South Dakota: Ground-Water Characteristics, 4661. Retrieved from https://pubs.usgs.gov/wri/wri024094/pdf/mainbodyofreport- 3.pdf
-
Elahcene, O., El-Azim, H. A., & Aidoud, A. (2019). Physico- chemical and bacteriological analysis of water quality in different types of water from the Ain Zada Dam of Bordj Bou Arreridj (Algeria). Egyptian Journal of Aquatic Biology and Fisheries, 23(3), 423439.
https://doi.org/10.21608/ejabf.2019.49062
-
Fadiran, A. O., Dlamini, S. C., & Mavuso, A. (2008). A comparative study of the phosphate levels in some surface and ground water bodies of Swaziland. Bulletin o the Chemical Society of Ethiopia, 22(2), 197206.
https://doi.org/10.4314/bcse.v22i2.61286
-
Guillemant, J., Le, H. T., Accarie, C., Du Montcel, S. T., Delabroise, A. M., Arnaud, M. J., & Guillemant, S. (2000). Mineral water as a source of dietary calcium: Acute effects on
parathyroid function and bone resorption in young men. American Journal of Clinical Nutrition, 71(4), 9991002. https://doi.org/10.1093/ajcn/71.4.999
-
Gulumbe, B. H., Aliyu, B., & Manga, S. S. (2016). Bacteriological and Physicochemical Analyses of Aliero Dam Water. International Journal of Innovative Studies in Sciences and Engineering Technology, 4863(April), 3034.
-
Gupta, N., Pandey, P., & Hussain, J. (2017). Effect of physicochemical and biological parameters on the quality of river water of Narmada, Madhya Pradesh, India. Water Science, 31(1), 1123. https://doi.org/10.1016/j.wsj.2017.03.002
-
Howladar, M. F., Numanbakth, A. Al, & Faruque, M. O. (2017). An application of Water Quality Index ( WQI ) and multivariate statistics to evaluate the water quality around Maddhapara Granite Mining Industrial Area , Dinajpur , Bangladesh. Environmental Systems Research, 6(13). https://doi.org/10.1186/s40068-017-0090-9
-
Idiata, D. J. (2015). Investigation of the Relationship between Electrical Conductivity and Total Dissolved Solids for Mono- Valent, Di-Valent and Tri-Valent Metal Compounds. International Journal of Engineering Research and Reviews, 3(1), 4048. Retrieved from www.researchpublish.com
-
Imneisi, I. B., & Aydin, M. (2016). Water Quality Index (WQI) for Main Source of Drinking Water (Karaçomak Dam) in Kastamonu City, Turkey. Journal of Environmental & Analytical Toxicology, 6(5). https://doi.org/10.4172/2161- 0525.1000407
-
Ingale, A. M., & Jadhal, S. M. (2017). Determination of Water Quality Index of Three Different Water Bodies in Amravati City. International Journal of Recent Engineering Research and Development, 02(08), 5458.
-
Ishaku, J. M. (2011). Assessment of groundwater quality index for Jimeta- Yola area, Northeastern Nigeria. Journal of Geology and Mining Research, 3(9), 219231.
-
Ishaku, J. M., Ahmed, A. S., & Abubakar, M. A. (2012). Assessment of groundwater quality using water quality index and GIS in Jada , northeastern Nigeria. Journal of Earth Sciences and Geotechnical Engineering, 1(1), 3560.
-
Ishaku, J. M., Kaigama, U., & Onyeka, N. R. (2011). Assessment of groundwater quality using factor analysis in Mararaba-mubi area , Northeastern Nigeria. Journal of Earth Sciences and Geotechnical Engineering, 1(1), 933.
-
Kachroud, M., Trolard, F., Kefi, M., & Jebari, S. (2019). Water Quality Indices: Challenges and Application Limits in the Literature. Water (Switzerland), 11(361), 126. https://doi.org/10.3390/w11020361
-
Kale, V. S. (2016). Consequence of Temperature , pH , Turbidity and Dissolved Oxygen Water Quality Parameters. International Advanced Research Journal in Science, Engineering and Technology, 3(8), 186190.
https://doi.org/10.17148/IARJSET.2016.3834
-
Khatri, N., & Tyagi, S. (2015). Influences of natural and anthropogenic factors on surface and groundwater quality in rural and urban areas. Frontiers in Life Science, 8(1), 2339. https://doi.org/10.1080/21553769.2014.933716
-
Kottek, M., Grieser, J., Beck, C., Rudolf, B., & Rubel, F. (2006). World map of the Köppen-Geiger climate classification updated. Meteorologische Zeitschrift, 15(3), 259263.
https://doi.org/10.1127/0941-2948/2006/0130
-
Kumar, Y. R., Reddy, M. D., Jayaveera, K. N., & Pradesh, A. (2016). Studies on groundwater quality in anantapur district. International Journal of Pllant, Animal and Evironmental Science, 6(2), 219225.
-
LHirondel, J., & LHirondel Jean-Louis. (2001). Nitrate and man: toxic, harmless or beneficial? Nitrate and man: toxic, harmless or beneficial?
https://doi.org/10.1079/9780851995663.0000
-
Leo M. L. Nollet. (2007). Handbook of Water Analysis. (Leo M.
L. Nollet, Ed.) (Second Edi). Boca Raton, FL: CRC Press,
Taylor & Francis Group. https://doi.org/10.1038/006104a0
-
Mahato, S., Mahato, A., Karna, P. K., & Balmiki, N. (2018). Investigating aquifer contamination and groundwater quality in eastern Terai region of Nepal. BMC Research Notes, 11(1), 1 7. https://doi.org/10.1186/s13104-018-3445-z
-
Martins, A. K., & Gadiga, B. L. (2015). Hydrological and Morphometric Analysis of Upper Yedzaram Catchment of Mubi in Adamawa State , Nigeria . Using Geographic Information System ( GIS ). World Environment, 5(2), 6369. https://doi.org/10.5923/j.env.20150502.03
-
Meride, Y., & Ayenew, B. (2016). Drinking water quality assessment and its effects on residents health in Wondo genet campus, Ethiopia. Environmental Systems Research, 5(1), 17. https://doi.org/10.1186/s40068-016-0053-6
-
Nemati Varnosfaderany, M., Mirghaffary, N., Ebrahimi, E., & Soffianian, A. (2009). Water quality assessment in an arid region using a water quality index. Water Science and Technology, 60(9), 23192327. https://doi.org/10.2166/wst.2009.669
-
Nyamangara, J., Jeke, N., & Rurinda, J. (2013). Long-term nitrate and phosphate loading of river water in the Upper Manyame Catchment, Zimbabwe. Water SA, 39(5), 637642. https://doi.org/10.4314/wsa.v39i5.7
-
Oni, O., & Fasakin, O. (2016). The Use of Water Quality Index Method to Determine the Potability of Surface Water and Groundwater in the Vicinity of a Municipal Solid Waste Dumpsite in Nigeria. American Journal of Engineering Research (AJER), 5(10), 96101. Retrieved from www.ajer.org
-
Oyem, H. H., Oyem, I. M., & Ezeweali, D. (2014). Temperature, pH, Electrical Conductivity, Total Dissolved Solids and Chemical Oxygen Demand of Groundwater in Boji-Boji Agbor/Owa Area and Immediate Suburbs. Research Journal of Environmental Sciences, 8(8), 444450.
https://doi.org/10.1111/ijfs.12122
-
Prathumratana, L., Sthiannopkao, S., & Kim, K. W. (2008). The relationship of climatic and hydrological parameters to surface water quality in the lower Mekong River. Environment International, 34(6), 860866.
https://doi.org/10.1016/j.envint.2007.10.011
-
Ranawat, K., Singh, N., Chourasiya, R., & Bhatnagar, P. (2017). Investigation of water quality index ( wqi ) of different water sources in ratlam town , madhya pradesh , india. Life Sciences International Research Journal, 4(1), 224228.
-
Rusydi, A. F. (2018). Correlation between conductivity and total dissolved solid in various type of water: A review. IOP Conference Series: Earth and Environmental Science, 118(1). https://doi.org/10.1088/1755-1315/118/1/012019
-
Saito, T., Hamamoto, S., Ueki, T., Ohkubo, S., & Moldrup, P. (2017). Temperature change affected groundwater quality in a con fi ned marine aquifer during long-term heating and cooling. Water Research, 94(2016), 120127.
https://doi.org/10.1016/j.watres.2016.01.043
-
Seli, A. B., Ankidawa, B. A., Ishaku, J. M., & Aminu, M. D. (2019). Geochemical assessment of groundwater quality: A case study from Adamawa in Northeastern Nigeria. International Journal of Water, 13(3), 269292.
https://doi.org/10.1504/IJW.2019.101339
-
Sengupta, P. (2013). Potential health impacts of hard water.
International Journal of Preventive Medicine, 4(8), 866875.
-
Sharma, Y., & Kaur, K. (2017). Determination of Nitrates and Sulphates in Water of Barnala ( Punjab , India ) Region and Their Harmful Effects on Human Lives. International Journal of Advanced Research in Education & Technology, 3(February),
7982.
-
SON. (2007). Nigeria Standards for Drining Water Quality. Nigerian Industrial Standards (Vol. 203).
-
Tian, Y., Jang, Y., Liu, Q., Dong, M., Xu, D., Liu, Y., & Xu,
X. (2019). Using a water quality index to assess the water quality of the upper and middle streams of the Luanhe River, northern China. Science of the Total Environment, 667(February), 142151.
https://doi.org/10.1016/j.scitotenv.2019.02.356
-
TirkeyPoonam, BhattacharyaTanushree, & ChakrabortySukalyan. (2015). Water Quality Indices-Important Toolsfor Water Quality Assessment: a Review. International Journal of Advances in Chemistry (IJAC), 1(1), 1529.
https://doi.org/10.5121/ijac.2015.1102
-
Tripathi, B., & Shukla, D. N. (2017). Determination of water quality index and physico- chemical parameter value of the river ganga at phaphamau , allahabad. Global Journal of Bio-Science and Biotechnology, 6(2), 224228.
-
Triplett, Laura D., Burford, Patricia, Sielaff, Brad, Clark, R. C. (2006). Sampling Procedures for Ground Water Monitoring Wells, (September), 15.
https://doi.org/10.1017/CBO9781107415324.004
-
Tyagi, S., & Sharma, B. (2013). Water Quality Assessment in Terms of Water Quality Index. American Journal of Water Resources, 1(3), 3438. https://doi.org/10.12691/ajwr-1-3-3
-
Tyagi, S., Sharma, B., Singh, P., & Dobhal, R. (2013). Water Quality Assessment in Terms of Water Quality Index. American Journal of Water Resources, 1(3), 3438.
https://doi.org/10.12691/ajwr-1-3-3
-
United Nations. (2019). World Population Prospects.
-
van Vliet, M. T. H., & Zwolsman, J. J. G. (2008). Impact of summer droughts on the water quality of the Meuse river. Journal of Hydrology, 353(12), 117.
https://doi.org/10.1016/j.jhydrol.2008.01.001
-
Wante, S. P., & Anoliefo, G. O. (2014). Impact of developmental needs of the people of Mubi-North local government area of Adamawa state , Nigeria on environmental sustainability, 6(6), 13031316.
-
Ward, M. H., Jones, R. R., Brender, J. D., de Kok, T. M., Weyer,
P. J., Nolan, B. T., van Breda, S. G. (2018). Drinking water nitrate and human health: An updated review. International Journal of Environmental Research and Public Health, 15(7), 131. https://doi.org/10.3390/ijerpp5071557
-
WHO. (2017). Guidelines for Drinkingwater Quality (Fourth (In). Geneva: World Health Organization.
-
World Health Organization. (2017). Water Quality And Health
– Review Of Turbidity: Information for regulators and water suppliers. Retrieved from https://www.who.int/water_sanitation_health/publications/turbi dity-information- 200217.pdf%0Ahttp://www.who.int/water_sanitation_health/p ublications/turbidity-information-200217.pdf
-
Yada, S., Lami, J., Usman, A., Bulama, G., & Zira, Z. (2017). An Assessment of Water Quality of Some Dug Wells and Boreholes in Gella, Mubi South Local Government Area of Adamawa State. Journal of Scientific Research and Reports, 15(6), 111. https://doi.org/10.9734/jsrr/2017/34360
-
Yisa, J., Jimoh, T. O., & Oyibo, O. M. (2012). Underground Water Assessment using Water Quality Index. Leonardo Journal of Science, (21), 3342.
-
Yogendra, K., & Puttaiah, E. T. (2008). Determination of Water Quality Index and Suitability of an Urban Waterbody in Shimoga Town, Karnataka. In Proceedings of Taal 2007: The 12th World Lake Conference: 342-346 Determination (pp. 342 346).
-
Yonnana, E., Yamta, S., Kaigama, I., & Bedeson, A. (2017). Assessment of Water Quality for Goro Dong (Lake) and Its Suitability for Consumption and Domestic Use by the Immediate Lake Communities in Numan, Adamawa State Nigeria. Journal of Geography, Environment and Earth Science International, 12(4), 18.
https://doi.org/10.9734/jgeesi/2017/37517
-
Yusuf, A. K., & Alkali, S. C. (2018). Variation of Groundwater Parameters through West to East Central Profile Section in Mubi Town , Northeastern Nigeria, 6(3), 112122.
-
https://doi.org/10.12691/ajwr-6-3-2