
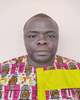
- Open Access
- Authors : Rodrigue Djakou Yopo , Mathias Fonteh Fru , Boris M. Djousse Kanouo , Jean Paul Ii Nsoga Nsoga
- Paper ID : IJERTV10IS070150
- Volume & Issue : Volume 10, Issue 07 (July 2021)
- Published (First Online): 19-07-2021
- ISSN (Online) : 2278-0181
- Publisher Name : IJERT
- License:
This work is licensed under a Creative Commons Attribution 4.0 International License
Assessment of the Level of Drinking Water Supply Service Provision: Case study of Mayo-Tsanaga River Sub-Basin in Cameroon
Rodrigue Djakou Yopo1*, Mathias Fonteh Fru1, Boris M. Djousse Kanouo1, Jean Paul Ii Nsoga Nsoga2
1*Department of Agricultural Engineering, FAAS, University of Dschang, Dschang, Cameroon.
2Department of Hydraulics and Water Management, NPSM, University of Maroua, Maroua, Cameroon.
Abstract – Drinking water supply systems are essential for life and development; this justifies the special attention given to services they provide under various conditions. Indicators of access to drinking water are most often based on technical assumptions and the use of drinking water infrastructures which do not reflect the actual service delivered or the actual percentage access to water by the population. This study highlights the weaknesses of the indicators currently used to define access to drinking water and proposes a more accurate set of indicators which should be used in determining drinking water services. Data for the study was obtained from secondary sources and primary data from a case study. The primary data were collected using direct observations and semi-structured interviews in the Mayo-Tsanaga river sub-basin, in the Sudano- sahelian zone of Cameroon where the concept of drinking water service is to be implemented in municipalities, which are key stakeholders for local development. The data were analysed using the Excel and XLStat software. The WASHCost service scales were used, to obtain more realistic indicators of the state of drinking water access and service. Results shows that the population had access to 15 litres of water per capita per day and the average number of users per day at a water point was 83. These values represent 60% and 30% respectively of values determined using the conventional approach. Drinking water supply service was estimated to be 63% reliable, which is from the water security viewpoint. These results indicate the need for a radical change of approach/indicators used in assessing drinking water projects and programs. The WASHCost service scales indicators should henceforth be used to determine drinking water supply service.
Keywords: WASHCost, access to water, drinking water infrastructure, indicators, Mayo-Tsanaga
-
INTRODUCTION
Drinking water supply systems are essential for life and development and hence, have always received special attention. Access to drinking water is usually defined in relation to the system that supplies the water which delivers a level of service to a given population. A water supply system is defined as the combination of natural, technological and socio-economic elements coordinated to provide an adequate level of service to users for consumption or domestic use [F. Cubillo, and L. Garotte, Drought risk and vulnerability in water systems, Options Méditerranéennes, vol. A80, p. 7[1]] [[1]]. According to [[2]] a drinking water supply system includes the water resource, the infrastructure and the demand / access interface linked to users (referred to as the RIDA grid). This definition takes into account the service offered by technology. This
service includes a set of indicators such as the quantity of water supplied, the water quality, its accessibility, its reliability, the traffic at the water point. A conceptual difference is made between the service itself and the system (tangible and intangible) used to deliver it [[3]]. In developing countries, water supply is achieved using many different technologies. This complicates the analysis of service actually delivered. Furthermore, it is often overlooked that over time, a system or infrastructure deteriorates and is no longer able to provide the initial level of service. Likewise, users can use more than one type of system for drinking water supply, depending on the season, access conditions, traffic, or the limitation of the productive capacities of the systems.
A level of service is a term used to describe and differentiate the qualities of a service. It is a concept that can be understood using a scale where each level or degree represents an increase from the previous level. The concept of "service scale" was first used in the sanitation sub-sector. More recently, it has been extended by the Joint Monitoring Program (JMP) to equally cover the water sector [[4]]. According to [[5]], the most common indicators for assessing the quality or water service level include; the quantity measured in litres per capita per day (l h-1 d-1 ); the quality, typically composed of one or more indicators related to the chemical and biological qualities; and the distance (accessibility) between a household or community centre and a water point. Some countries use other indicators, such as the number of people sharing a water point or the traffic at the water point, and service reliability, defined as the duration the infrastructure functions without a breakdown or the proportion of improved systems in a locality that can provide water year-round.
Different scales have been used to evaluate the level/scale of water service offered by water supply systems. The Joint Monitoring Program (JMP) [[4]] uses a simple scale based on three criteria: unimproved or open water points; improved or protected water sources, and domestic tap or running water. Less emphasis is put on water quality and quantity. It gives little details, making cost evaluation difficult. The Multiple Usage Service MUS scale [[6]] is based on five criteria and considers water used for subsistence agriculture and domestic purposes. These levels are known as: High MUS, middle MUS, basic MUS, basic domestic and no domestic service. Unlike the JPM approach, it correlates to some extent the quantity of water to the level of service. WASHCost has developed an improved model for assessing drinking water service level that uses a set of core indicators associated with
different types of technologies [[5]]. The WASHCost approach is based on five criteria depicting service level as high, intermediate, basic, reduced and zero/absence. The main indicators used are: water quality, quantity, access and reliability. This approach is more accurate as it links technologies to the standards of drinking water services to be delivered. The level of water service is assessed based on the actual water supplied by water systems.
In Cameroon like in many other African countries, access to water supply and thus, the level of water service is still based on assumptions, i.e. coverage is calculated by counting the number and types of existing and functioning water supply systems for a given population without considering whether they are actually providing the expected level of service [[5]] and user satisfaction [[3]]. The objectives of this study were to: highlight the weaknesses of current indicators used to determine the level of drinking water supply service and to propose the adoption of a more accurate approach in the study area.
-
MATERIALS AND METHODS
-
Characteristics of the study area
The Mayo-Tsanaga river sub-basin lies between latitude 10°28 and 10°54 North and longitude 13°40 and 14°40 East (Fig. 1). It has an ephemeral river that drains an area of 2064 km² [[7]].
Fig. 1: Location of the Mayo-Tsanaga river sub-basin.
The upstream area of the sub-basin, from Mokolo to Minglia (45% of the area) is characterized by a mountainous landform, with peaks reaching 1392 m and steep slopes ranging from 5% to 10%. These mountains are overlaid at the Cameroonian-Nigerian border by the Mandara mountains chain on which most of the tmporary rivers called mayos draining the sub-basin originate. The area at the foot hills (between Minglia and Maroua, 35% of the area) is characterised by gentle slopes ranging between 2% and 5% and by a tabular landform associating terraces with alluvial plains from which some "Inselbergs" emerge. The downstream zone (to the East), between Maroua and Bogo is a vast alluvial plain, characterized by flat and monotonous landforms and very light slopes (<2%) [[8]].
The Mayo-Tsanaga river sub-basin is located in the Soudano-Sahelian zone with a dry tropical climate and a Sahelian regime comprising two very distinct seasons: a dry
season of 08 to 09 months (October – May) harsher as you move north and away from the Mandara mountains and a rainy season of 3-4 months. The insolation is high and temperatures are generally lower from December to February (28°C daily average) and increase considerably, reaching peaks of 45°C between March and May. The thermal amplitudes sometimes reach 20°C. A dry wind called Harmattan crosses the region like most Sahelian zones and is the dominant wind in the dry season. The relative humidity is very low in March and April [[9]]. Floods are often violent with high runoff coefficients. An instantaneous maximum flood of 315 m3 s-1 was recorded on July 15, 1994 according to [[8]]. Rains are irregular in their spatio-temporal distribution. The onset date of rains and therefore of the runoff is also extremely variable. The hydrographic network is made up of ephemeral rivers [[10]], the main one is the Mayo-Tsanaga and its main tributary the Mayo-Kalliao. Most of the Mayo-Tsanaga river sub-basin is found in areas where the aquifer is discontinuous with an estimate of 40% to 60% of drilling success rate for water boreholes [[11]].
The human environment is characterized by an ethnic diversity made up of the Moundang, the Guiziga and the Peuhls in the plains and, the Mafa and the Mandara in the Mandara mountains [[12]]. Mokolo and Maroua are the largest districts in terms of surface area and population (1,650 km² and 4665 km²; and, 242,274 inhabitants and 330,410 inhabitants respectively in 2005). Furthermore, Mokolo, which now host refugees, is approaching the size of the population of Maroua [[13]]. The internally displaced persons (IDP) in the Mayo-Tsanaga Division are 76,815 while 5,662 inhabitants are refugees living outside the Minawaos camp [[14]].
-
Analysis of indicators used to assess the level of drinking water service in most sub-Saharan Africa countries
An analysis of the literature was used to come up with assumptions commonly applied in most countries in sub- Saharan Africa as concerns access to potable water. The aim was to determine whether these assumptions were realistic or not. A critical analysis of national standards for access to drinking water in Cameroon and Burkina Faso was made to identify their limits in relation to the services delivered to users. The analysis focused on two indicators: the flow rate and the number of people using the water point (traffic). In order to assess the limitations, these indicators were calculated using samples from 96 water points in the study area. The flow rate was estimated from normal pumping using a 10 litres bucket and a stopwatch. The traffic was obtained from interviews with the managers in charge of water points. The data collected was analysed using Microsoft Excel spreadsheet.
-
Implementation of the WASHCost model in evaluating the level of drinking water service in Mayo Tsanaga
A more accurate approach has been suggested by Moriarty using WASHCost Approach [[5]]. Drinking water supply systems must deliver a quantity of water above the expressed needs; this amount of water should be monitored regularly to ensure that, over time, it covers the expressed
needs. Otherwise, alternatives must exist to supply drinking water to the community. It is important to assess the quality of the water supplied on a regular basis. As part of the implementation of the Moriartys approach, data was collected from samples of water points coupled with semi- structured interviews with users and managers in charge of water points in the five administrative units of the study area. The population of the study area was estimated from the growth rate and the average size per household [[13]] carried out in the year 2017. The sample size was determined using
the following formula.
p is the effective average population of users / households with access to the water point.
q1 is the average quantity (in litre) of water collected per user
/ household
2 = 2
h is the agreed time for the water point to be in service in 24 hours.
q2 is the average production flow of the considered structure (in litres per hour).
NB: Average production flow rates of water infrastructure
Where n = sample size;
= 2(1 )2
were the flow rates obtained on the basis of the average of water supplied by manual, hand pump, solar powered pump, and submerged electric pump. These flow rates are always
Z = confidence level according to the reduced centred normal
distribution (for a confidence level of 95%, z = 1.96);
p = estimated proportion of the population exhibiting the characteristic (unknown p = 0.5);
d = tolerated error (for example we want to know the real proportion to within 5%).
The actual level of drinking water service was obtained by answering Moriartys questions [[5]]: Do the designed systems provide the desired amount of water? Do they do so every day? Does every member of the community have access to it? Do they meet national quality standards? The questions were administered to 600 households using semi-structured interviews. A great variety of people in the study area were concerned. These include the host community (Host), refugees (OoCR), Returnees (Ret.) and internally displaced persons (IDPs). The characteristics of respondents are shown in Fig. 2.
200
lower than the theoretical recharge flow rate obtained during pumping tests.
The questionnaire of drinking water infrastructure was administered in the administrative units on the basis of the proportion of refugees, returnees and internally displaced persons that it has. This information was provided through reports from the International Organization for Migration [[14]]. The sample is presented in the Table 1. The survey was carried based on 96 drinking water infrastructures.
Type of WP \ Situation
Rural area
Urban area
Borehole with HMP
36
24
Borehole with electric pump
0
1
MiniWSP with standpipe
4
0
MiniWSP with connection
1
0
Unimproved source
1
0
Developed well with HMP
0
1
Manual well
3
2
Traditional well
14
9
Total
59
37
Type of WP \ Situation
Rural area
Urban area
Borehole with HMP
36
24
Borehole with electric pump
0
1
MiniWSP with standpipe
4
0
MiniWSP with connection
1
0
Unimproved source
1
0
Developed well with HMP
0
1
Manual well
3
2
Traditional well
/td>
14
9
Total
59
37
TABLE 1. DWSS identified in the Mayo-Tsanaga river sub-basin
150
100
157
55 59
116
91
79
Hôte OoCR PDI
Water systems intended for human consumption consist mainly of boreholes with HMP (62.5%) and traditional wells (24%). These are the improved and unimproved autonomous
50 37 29
0
F H
Ret
water points respectively. The boreholes exploit the groundwater table while the wells exploit the surface water table with drying up and pollution observed in the dry season [[18]]. The water quality was assessed using five parameters:
chlorine, pH, turbidity, odour and bacteriological analysis.
Fig. 2: Distribution of respondents by gender and status
Accessibility of drinking water was determined as the distance users cover from home to the water point and back, as well as the time taken to collect water. Drinking water supply was assessed using the following indicators: traffic; the frequency of breakdowns; water quality; reliability determined by how long water was delivered in a 24-hour day and in a year; the average quantity (litres) of water collected by a user per day; the volume (litres) actually consumed by users of a water point (V1); and the volume (litres) of water actually supplied by type of infrastructure to users (V2),
1 = 1
The data was analysed using Excel and XLStat Softwares.
-
-
RESULTS AND DISCUSSION
-
Weaknesses of indicators used to assess the level of drinking water service in most sub-Saharan Africa countries
In Sub-Saharan Africa most often, access to safe drinking water refers to the percentage of the population with access to an adequate supply of safe drinking water at home or within a reasonable distance from home. According to [[15]], access to drinking water is defined under satisfactory conditions as: "availability of 20 litres per inhabitant and per day at a distance of less than 1000 m". This definition of access to water commonly used in sub-Saharan Africa is the baseline
hypothesis for drinking water access. In Cameroon and Burkina Faso for example, the ministries in charge of water defined an Equivalent Water Point (EWP) representing "any drinking water mobilization work, designed to supply the population according to the standards adopted: namely 250 to 500 people approximately, with a daily allowance set at 25 to 40 litres per inhabitant, the water point having to supply approximately 7.5 to 8 m3 of water for 12 hours per day [[3]] [[17]]. According to this standard, the following weaknesses were observed:
-
According to these norms, a water point is referred to as adequate if it supplies 0.6 m3 h-1 minimum flow using motorized pumping. However, human power pumping is less efficient than motorized pumping. This means, it will take more than 12 hours pumping time at the water point to deliver the target volume. Moreover, in these countries most often in the year, high temperatures do not allow manual pumping of water during a significant part of the day. Moreover, normative values do not take into account breakdowns occurring on the pumping systems and which reduce the quantity of water delivered. So, the assumption that a water point (borehole, well, etc.) with a flow of 0.6 m3 h-1 can supply 25 to 40 litres per capita per day to 250-500 inhabitants is an unrealistic basis of calculation. Fig. 3 illustrates the difference between reference flows measured with motorized pumps and the current flow obtained by pumping water with a hand pump in the Mayo-Tsanaga river sub-basin.
Fig. 2. Difference between the reference flow measured with the motorized pump and the actual flow measured with the hand pump.
Based on Figure 2, the actual value obtained varies between
Number of users
Number of users
-
and 0.4 m3 h-1. Taking the maximum value of 0.4 m3 h-1, we can expect, based on the same standard, to cover a maximum of 192 inhabitants per water point. This analysis was corroborated by the actual number of people frequenting the water point (traffic) as presented in Fig. 3 which shows an average of 83 users per water point.
300
200
100
0
0
20
40
60
80
100
300
200
100
0
0
20
40
60
80
100
Water points
Reference numbre of users Number of users per water point
Water points
Reference numbre of users Number of users per water point
Fig. 3. Number of users per water point compared to the reference
-
For the calculation of the rate of access to drinking water, using the same approach, the volumes of water indicated for the equipment (hang pump, electric pump, solar
-
pump, etc.) correspond to theoretical volumes and not to the volumes actually consumed by users.
These analyses show that current indicators used to appreciate the level of drinking water service are inappropriate. As a result, the quality of the calculated indicators (drinking water access rate) is highly questionable. A number of authors have clearly highlighted these difficulties [[3]] and [[16]].
-
-
-
WASHCost model of evaluating the level of drinking water service in Mayo-Tsanaga
-
Quantities of water collected each day by households
Table 2 shows statistics of the amount of water collected each day and the traffic around water points.
TABLE 2. Statistics of the average water consumption per household per day during the rainy and the dry season, and the average traffic around the
water points in rural and urban areas.
Statistics
Average water consumption per household per day (litres)
Average traffic around the water points
Dry season
Rainy season
Rural area
Urban area
Nbr. of observations
96.0
96.0
59
37
Minimum
10.0
7.0
10.0
50.0
Maximum
300.0
200.0
200.0
200.0
1st Quartile
60.0
40.0
50.0
60.0
Median
80.0
60.0
80.0
80.0
3rd Quartile
100.0
80.0
95.0
90.0
Mean
90.1
68.0
81.7
83.3
Variance (n-1)
2947.9
1901.9
2078.0
1051.4
Standard deviation
(n-1)
54.3
43.6
45.6
32.4
From Table 2, average water consumption of 67.9 l d-1 per household during rainy season compared to 90.1 l d-1 per household during dry season is determined. This difference is statistically different at 5% degree of freedom (p-value two- tailed = 0.002). Also, the average traffic calculated around the water points, respectively 81.7 in rural areas and 83.3 in semi- urban areas, were not statistically different at 5% degree of freedom (p-value two-tailed 0.848). However, they were below Cameroonian standards of 250 people per water point in semi-urban areas and 300 people per water point in rural areas. The average daily water collection per household was less than 100 litres. Based on the Cameroonian standard, this will be fine for a household of 4 people. However, a survey conducted on more than 600 households in the study aea indicates an average of 7 persons per family. That said, the actual average daily household water collection was estimated to be 15 litres per person per day. This average value contrasts with the low traffic around the water points (< 100 users per water point).
-
Water quantity and access to it by the population
Table 3 shows the perception of users of the access to potable water sufficient for basic needs.
TABLE 3. Statistics of water users responses to the variable Do you have
access to water for drinking and cooking?
Variable
Categories
Frequency
Relative
frequency (%)
Do you have access to potable water for drinking and cooking?
No
105
16.9
Yes, in sufficient quantity
253
40.6
Yes, but in insufficient quantity
265
42.5
Total
623
100
More than 75% of respondents indicated that they had access to drinking water for basic needs; of these, half considered this water sufficient while the other half considered it insufficient. More than 95% of the time, users go to fetch water on foot. The average time taken to fetch the water (< 30 min) and the means of transport (foot) are dependent (p-value
= 0.0001) with an error of 5%. The distance to water points is generally less than a kilometre. Girls under 18 (22.15%) and women aged between 18 and 59 (42.22%) are mainly in charge of fetching drinking water. Women over 60, boys under 18 and men aged 18 to 59 represent less than 20% of those involved in fetching drinking water. The majority of women involved, carry water on their heads, walked on foot and may not always have the time and energy to lift a heavy container or make several trips per day. This would explain why the household is satisfied with modest water quantities.
-
Water supply systems and national quality standards
The water quality of all improved drinking water points in the Mayo-Tsanaga river sub-basin representing 98% of water points was analyzed immediately after construction and all were of good quality. After these initial tests, less than 10% of improved drinking water systems have carried out water quality test at least once. The results of the water quality analysis carried out as part of this study revealed that 79% of water points were contaminated with faecal coliforms, of which 58% are boreholes and 100% are wells. Disinfection of water points with chlorine has not been done during the last six months for all water points. Boreholes have better water quality than wells because they use groundwater that is less prone to contamination [[18]]. Exposures to external hazards are associated to the sensitivity of water production systems and to other factors [[2]], [[19]], [[20]], [[21]] and, [[22]].
-
Daily water supply capacities of recognized adequate systems
Table 4 presents the average daily volumes of water theoretically supplied by different types of infrastructure and the average daily volumes of water actually consumed by users in the dry and rainy seasons.
TABLE 4. Average daily volumes theoretically supplied and actually consumed by type of recognized adequate drinking water production infrastructure.
Area
Infrastructure
Average daily volumes theoretically supplied (litre)
Average daily volumes actually consumed (litre)
Type
Numbe r
Dry Season
Rainy season
Rural
Borehole with hand pump
36
14143
8415
6732
MiniWSP with standpipe
4
11137
13875
10125
Urban
Borehole with hand pump
24
13901
8269
6319
Borehole with electric pump
2
21600
36000
24000
Developed well with HMP
1
17280
5000
3000
In Table 4, the average daily volumes theoretically supplied were evaluated based on the average water production flow rate of the considered water point and refer to its average daily production at the agreed service hours.
The trends highlighted in Table 4 indicate that drinking water supply structures are used more in the dry season. However, whatever the season, only half the theoretical daily water production of the water point is consumed. Hence there is a possibility of improving the drinking water service actually provided. These conclusions are consistent with the information of the low traffic around the water points (< 100 users per water point) and the low rate of access to drinking water (15 litres per user per day) obtained.
By applying the WASHCost drinking water service scale from [[5]], the drinking water service in the Mayo-Tsanaga river sub-basin could take the form of Table 5.
TABLE 5. Drinking water service in the Mayo-Tsanaga river sub-basin
according to WASHCost as compared to conventional service delivery.
Service delivery approach
Quantity (litres per capita per
day)
Quality
Accessibility (minute per capita par day)
Reliability
WASHCost
< 15 litres
Wells (100%) and boreholes (58%)
contaminated No disinfection done during the last six months
Less than 01 km
Less than 100 users per EWP
63%
improved sources
Conventional
25-30
litres
WHO norms, Water analyses performed at
least once a year
500 meters
250-300 users per EWP
100%
improved sources
With the WASHCost approach, people have access to 15 litres of water per person per day. This quantity is delivered through autonomous drinking water systems for which 63% are improved. Less than 100 person use the water point on regular basis and 79% of water points have their water contaminated with coliforms. Having access to 15 litres of water per person per day for the average traffic at the water points (83 persons per day) constitutes less than half of the infrastructure's daily production capacity. Coupled with the level of contaminated water points, the level of water supply service provision in the Mayo-Tsanaga river sub-basin is basic or reduced. The conventional approach would have shown it much higher, which would not reflect the reality.
-
-
CONCLUSION
This study revealed that common indicators used in most sub- Saharan Africa countries to determine the level of drinking water service do not give the actual service provided. These indicators, which give the thresholds for accessibility to infrastructure, usually 250 to 300 inhabitants per water point, are clumsily used to assess water supply projects and the drinking water service delivery. The normative values applied in most countries in sub-Saharan Africa to assess water service provision do not take into account the fact that water points are pumped manually and that, climatic conditions and failures in the pumping system contribute to reducing the amount of water supplied.
The case study carried out in the Mayo-Tsanaga river sub- basin has revealed that users have access to a reduced dinking water service, i.e. less than 15 litres of water per person per day although the water point can deliver more water. Likewise, there is little traffic around the water points compared to the normative standards. Improved water points are contaminated because disinfection has not been carried out. The situation thus determined would be assessed differently with the conventional indicators.
The drinking water service delivery can be improved in the Mayo-Tsanaga river sub-basin by a good organization of the collection and management of the water point. However, the quality of the water is a factor to be considered as its effects on human health could be harmful. From this case study, improved drinking water systems should be disinfected at least once each semester and unimproved ones should not be allowed for consumption uses. From this perspective, the main recommendation of the study is to adopt the WASHCost approach in assessing the drinking water service provided in order to better understand the disparities in this service.
REFERENCES
-
F. Cubillo, and L. Garotte, Drought risk and vulnerability in water systems, Options Méditerranéennes, vol. A80, p. 7, 2008.
-
M F. Mohammad, S. Saadati, and, A. Ahmadi, Vulnerability and risk reduction of water supply systems, ASCE In: World Environmental and Water Resources Congress 2010: Challenges of Change, pp. 4414 4426, 2010. http://www.ascelibrary.org.
-
C. Batchelor, S. Smits, et A.J. James, Adaptation de la fourniture des services dAEPHA au changement climatique et autres risques et incertitudes, Cahier Thématique (TOP) vol. 24, La Haye, Pays- Bas : IRC, 2011, p.105.
-
J. Nansi, C. Pezon, J. Boulenouar, A. Dubé, et R. Bassono, Accès aux ouvrages vs Accès aux services deau potable étude de cas de 24 villages dans la région du Sahel, Centre International de lEau et lAssainissement (IRC), 2013, p.68.
-
JMP, Progress on Drinking Water and Sanitation: Special Focus on Sanitation, Génève, Swizerland: UNICEF and WHO, pp.77, 2008. http://whqlibdoc.who.int/publications/2008/9789241563673_eng.pdf
-
P. Moriarty, C. Batchelor, C. Fonseca, A. Klutse, A. Naafs, et al., Echelle dévaluation du coût et de la qualité des services deau potable WASHCost, deuxième édition, Hague, Netherlands: IRC, 2011, p. 24.
-
B. Van Koppen, S. Smits, P. Moriarty, F. Penning de Vries, M. Mikhail, and E. Boelee, The Water Ladder: Multiple-use of water services for poverty reduction, Hague, Netherlands: IRC and IWMI, p.213, 2009.
-
L. Leroux, J. Oszwald, NB. Ngatcha, D. Sebag, M-J. Penven, et E. Servat, Le bassin versant du Mayo-Tsanaga (Nord Cameroun) : un bassin versant expérimental pour une compréhension des relations Homme/Milieu, Revue Francaise de Photogrammetrie et de Teledetection, p.16, 2013. https://www.researchgate.net/publication/235758171
-
G. Lienou, Impact de la variabilité climatique sur les ressources en eau et les transports de matières en suspension de quelques bassins versants représentatifs du Cameroun, Thèse de doctorat, Département des sciences de la terre, Yaoundé, Cameroun: Université de Yaoundé I, 2007, p.486.
-
MINEPAT / DREN, Rapport socio-économique de la région de lExtrême Nord du Cameroun, Maroua, Cameroun: Ministère de lEconomie, de la Planification et de lAménagement du Territoire / Délégation Régionale de lExtrême-Nord (MINEPAT / DREN), 2010, pp.100.
-
N.B. Ngounou, J. Mudy, J.F. Aranyossy, E. Noah, et R.J. Sarrot, Apport de la géologie, de lhydrologie et des isotopes de lenvironnement à la connaissance des nappes en creux du Grand yaéré (Nord Cameroun), Revue des sciences de leau / Journal of water science, vol. 1(20), pp.29-43, 2007.
-
M. Detay, Atlas de la province de lextrême Nord du Cameroun : Hydrogéologie, Ministère de la Recherche Scientifique et Technique, Paris, France : Ministère de la Recherche Scientifique et Technologique (MINREST), 2000, p.7.
-
C. Seignobos, et O. Lyébi-Mandjek, Atlas de la province Extrême- Nord Cameroun : Mise en place du peuplement et répartition ethnique Nouvelle Editions, Marseille, France : IRD, 2000, pp.38-
43. https://book.openéditions.org/irdeditions/11557
-
Recensement Général de la Population Humaine (RGPH), Etat et structure de la population, vol. II, tome I. Yaoundé, Cameroun : BUCREP, 2005, p.190.
-
Organisation Internationale des Migrations (OIM), Rapport sur les Déplacements, Round 13, Région de lExtrême-Nord. Maroua, Cameroun: DTM, 2018, p.45.
-
Joint Monitoring Program (JMP), Global Water Supply and Sanitation Assessment 2000 Report, Genève, Suisse: OMS et UNICEF, 2000, p. 80.
-
C. Pezon, J.Nansi, et R. Bassono, De laccès aux systèmes de distribution deau potable à laccès aux services deau potable : méthode et outils, La Haye, Pays-Bas : IRC, 2012, p.32. www.whascost.info
-
MINMEE, Etude diagnostique (domaine de leau) – Stratégie du Ministère des Mines, de lEau et de lEnergie, Rapport détude, Yaoundé, Cameroun: MINMEE, 2005, p.84.
-
R. Djakou, M. Fonteh, et R. Njila, Vulnérabilité des systèmes dapprovisionnement en eau face aux risques climatiques en zone soudano-sahélienne : Cas de Mogode, Extrême-Nord Cameroun, Journal of the Cameroon Academy of Sciences, vol. 2(12), pp.115- 128, 2015.
-
A. Davinson, G. Howard, M. Stevens, P. Callan, L. Fewtrell, D. Deere, and J. Batran, Water Safety Plans Managing drinking water quality from catchment to consumer, Geneva, Switzerland: WHO, 2005, p.244.
-
Organisation Mondiale de la Santé (OMS), Water Safety Planning for Small Community Water Supplies: Step-by-step risk management guidance for drinking-water supplies in small communities, Genève, Suisse: OMS, 2012, p.66.
-
E. Ludi, R. Calow, and F. Greaves, Environmental assessment and risk screening for rural water supply, Oxford, UK: Consortium for Sustainable Water, Sanitation & Hygiene in fragile Contexts, 2015, p.56.
-
R. Calow, L. Eva, A. McKenzie, and S. Kebede, Climate and environmental risk screening for rural water supply in Ethiopia, London, UK: Overseas Development Institute (ODI), 2015, p.43.