
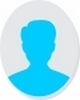
- Open Access
- Authors : Nicolas Titahelu , Samy Junus Litiloly , Mesak Frits Noya
- Paper ID : IJERTV10IS110012
- Volume & Issue : Volume 10, Issue 11 (November 2021)
- Published (First Online): 11-11-2021
- ISSN (Online) : 2278-0181
- Publisher Name : IJERT
- License:
This work is licensed under a Creative Commons Attribution 4.0 International License
CFD Assessment of Utilizing Flettner on 6500 DWT Container Ship
Nicolas Titahelu
Department of Mechanical Engineering, Universitas Pattimura
Maluku, Indonesia
Mesak Frits Noya
Samy Junus Litiloly
Department of Mechanical Engineering, Universitas Pattimura
Maluku, Indonesia
Department of Mechanical Engineering, Universitas Pattimura
Maluku, Indonesia
AbstractThe concept of Flettner rotor, a rotating cylinder immersed in a fluid current, with a top-mounted disk, has been analyzed by means of unsteady Reynolds averaged Navier- Stokes simulations, with the aim of creating a suitable tool for the preliminary design of the Flettner rotor as a ships auxiliary propulsion system. The simulation has been executed to evaluate the performance sensitivity of the Flettner rotor with respect to systematic variations of several parameters, that is, the spin ratio, the rotor aspect ratio, the effect of the end plates, and their dimensions. The Flettner rotor device has been characterized in terms of lift and drag coefficients, and these data were compared with experimental trends available in literature. A verification study has been conducted in order to evaluate the accuracy of the simulation results and the main sources of numerical uncertainty. All the simulation results were used to achieve a surrogate model of lift and drag coefficients. This model is an effective mathematical tool for the preliminary design of Flettner rotor. Finally, an example of assessment of the Flettner rotor performance as an auxiliary propulsion device on a real tanker ship is reported.
KeywordsComponent; formatting; style; styling; insert (key words)
technical report [1], the owner declares that thanks to this device a 30% reduction of fuel consumption is achieved. E- Ship 1 is in current use and has been recounted to cover more than 17000 sea miles with no mention of particular problems about the FRs [1]
The capability of infinite length rotating cylinders to produce aerodynamic forces was studied for the first time at the Langley NACA Laboratory by Reid [2]; he found that in particular conditions such simple devices are capable of developing very high values of the lift coefficient and of the aerodynamic efficiency (i.e., the lift-to-drag ratio). Thom [3] presented an experimental work on rotating cylinders, with emphasis on the effects of the Reynolds number (Re), surface conditions, aspect ratio, and end plates disks, describing the device in terms of lift, drag, and torque coefficients. Successively, Seifert [4] clarified the physics underpinning the FR, thus highlighting the nature of the circulation around the rotating cylinder. More recently, a comprehensive study on the functioning of FR was conducted by Da-Qing et al.,[5].
-
INTRODUCTION
In the era in which most of the worlds attention is focused on improving energy savings, the use of spinning cylinders as an auxiliary naval propulsion system has become a reality. Flettner rotors are rotating cylinders that, when immersed in a fluid stream, are able to produce fluid dynamic lift using the Magnus effect. This idea is due to the German engineer Anton Flettner who studied in the 1920s the effectiveness of spinning cylinders as a ships propulsion system. This kind of propulsion system was enrolled for the first time in 1925- 1926 on the Buckau ship, shown in Figure 1(a). This ship used two rotors to augment the propulsion power of its former conventional sailing rigs. However, further commercial development of the FRs did not take place before the twenty- first century. Nowadays, with increasing fuel prices and a general growing sensibility about green and environmental- protection policies, the FRs are being seriously reconsidered as viable green ship propulsion devices. In 2010, Enercon, a wind energy company, launched a Flettner-powered cargo ship named E-Ship 1, Figure 1(b). On E-Ship 1 the FRs are used to assist the diesel engine: as reported in the Enercon
Figure 1 E-Ship 1 by Enercon wind company
The magnus effect itself can be found in everyday life as in some sports, where the movement of the ball that is thrown by rotating on its axis will produce a different movement when compared with ordinary throwing ball. Today some parties have revived the concept of the rotor Flettner, and in the opinion of some, offer the most effective way to utilize wind as an additional thrust energy.
Figure 2 Magnus effect (a)and schematic of the resulting lift and drag forces onboard a ship (b)
The magnus effect is a force acting on a rotating object in a moving air stream, which is perpendicular to the airflow. The Magnus effect is generated when the rotating cylinder produces a fluid pressure difference. The Magnus effect was put forward by the German physicist Heinrich Gustav Magnus in 1852 [6].
The produced lift and drag forces by the Magnus effect are a supporting mean for ship propulsion if a rotating cylinder is properly mounted onboard the ship. The rotation of the cylinder is controlled by an electric motor mounted onboard the ship. The power consumed by the motor and the thrust produced from the Flettner rotor will regulate the amount of the main engine power that can be replaced. The produced thrust can be calculated as the summation of the lift and drag forces in the ship direction
-
LITERATURE STUDY
In the early 1920s, as an alternative to classical sails, Anton Flettner invented the Flettner rotor, an upright-mounted cylinder rotated by a motor [7]. In sideways wind the Magnus force, observed in many sports as it makes a ball with spin curve, creates a lift force that propels the ship forward (Fig. 1). A few ships were equipped with Flettner rotors and tested but the technology could not compete with steam and diesel ships at the time. Flettner rotors were reconsidered in response to the shipping crisis in the 1980s [8], and again in recent times, as focus is turning towards alternatives to fossil fuels, as indicated by a number of Computational Fluid Dynamics (CFD) studies [9]. In 2010, the10,000 dwt cargo vessel E-Ship 1 was completed, equipped with four Flettner rotors of 27 m height and 4 m in diameter, demonstrating the design feasibility of the technology.
Flettner rotors are controlled via a single parameter, their rotational speed. It is assumed that the rotors are incorporated into the ship structurally as by design large forces are at work, and to ensure the hydrostatic and dynamic stability of the vessel.
Flettner rotors take up deck space and very likely increase the overall height of the ship, presenting potential barriers to their installation, depending on the ships type and intended operational prole. It is noted that these assumptions and issues need to be assessed further when considering implementing Flettner rotors on a specic vessel. All results are calculated for a single rotor. Choosing an optimum number of Flettner rotors would depend on many fac- tors, such as vessel specics, which are outside the scope of this study, but assumptions about the number of rotors need to be ta- ken into account when putting results into context.
For a moving vessel with a speed of (Vs), the true wind speed and direction (Vt, ) will affect the Flettner rotor performance. This is because the changes in true wind direction to a moving vessel will result in a change in apparent wind speed (Va). Consequently, the apparent wind speed will affect Flettner rotor to generate the thrust in ship direction. Figure 2 illustrates the angles between ship and wind velocities as well as two coordinate systems. The (Xh, Yh, Zh) cordinate system is used for the vessel hull, while (Xf, Yf, Zf) is introduced for the course through the ocean.
Figure 3 Ship and wind velocity angles
-
METHOD
-
GOVERNING EQUATION
The Reynolds-averaged Navier-Stokes (RANS) method is a three-dimensional equation developed and used in the CFD model. One of the equations developed by ANSYS-CFX software is unsteady viscous incompressible flow to solve flow problems in the walls of ships. The averaged continuity and momentum equations for incompressible flows may be given as in the following two equations [10]. The equations of mass and momentum can be written as follows in Equations (1) and (2):
(1)
(2)
Furthermore, introduced Reynolds Averaged Navier-Stokes (RANS) in which configurated from modification of unsteady Navier-Stokes by involving averaged and fluctuating quantities. The turbulence model based on the RANS equation [11], as a statistical turbulence model caused by procedure of statistical average used to obtain the equations. Reynolds' average equations are given in Equations (3) and (4):
(3)
(4)
where is the stress tensor molecular consisting of normal and shear stress components
-
FLETTNER ROTOR DESIGN
The Flettner Rotor is conducted to computational fluid dynamics (CFD) simulation. The three-dimensional (3D) model used in this research is a full-scale replica. According to the following table 1, the Flettner Rotor is of the following dimensions:
TABLE 1 Size of the Flettner rotor Parameter Unit value Rotor Diameter (D) m 3
Rotor Heigh (H) m 15
Number of Flettner 1
Flettner Rotor
Figure 3 Container Ship with Flettner
-
BOUNDARY CONDITION AND MESHING
The domain dimensions and boundary conditions are specified in Figure 4. The boundary conditions are specified as follows: model is a no-slip condition is imposed on the wall surface; opening condition is applied to the top, side and
bottom walls; the flow velocity at inlet is defined as the current speed of wind (5-10m/s); at the outlet, the velocity set as same with inlet section.
Figure 4 Numerical Domain of Model
A good mesh is essential for accurate and dependable results. CFD Simulation, formally known as CFX, has a long tradition of innovative meshing technologies including Mesh Enhancement, Automatic Mesh Sizing, and Automatic Refinement. The mesh generation in this study is accomplished using Design Modeler. The calculation domain is discretized using unstructured meshes. Considering the complex geometrical characteristics of the Flettner Rotor a mesh with triangular elements is generated on the Flettner Rotor surface and the boundary layer is refined with prism elements created through extending the surface mesh node. The region around the Flettner Rotor is filled with tetrahedral
elements with interface domain, while in the far field, unstructured mesh with grid generation is generated for reducing the number of elements as shown Figure 5.
The mesh size plays an important role in the calculation procedure, a fine mesh can always bring credible results in ANSYS CFX but at the same time increases the computational cost and time consumption due to the large element number. Therefore, to determine the mesh size with acceptable numerical accuracy and element number, mesh convergence studies are carried out for the ship with Flettner. Grid model of Flettner Rotor according to Chung, [12] the number of used elements about 1,235,236.
Figure 5 Meshing mode
-
-
RESULT AND DISCUSSION
In this section, the technical, environmental, and economic results for using the Flettner rotor onboard the selected case study are presented. The results imply the effect of wind speed variation, ships speed, lift coefficient, motor speed, and rotation coefficient on the net output power of Flettner rotors.
It is important to include the aerodynamic interaction effects among different Flettner rotors when studying their performance onboard a ship. The potential part of this interaction is dominating as the apparent wind speeds and directions are changed according to the induced circulation (13). The apparent wind speed and direction at each rotor are highly affected by the position and the circulation of the other rotors. Therefore, the lift and drag coefficients, as well as the optimum rpm, should be calculated according to the characteristics of the experienced wind speed and directions. The wind speeds and angles agree with these practices by sail boats at various locations in a fleet (14). The optimum rpm for
each rotor is calculated according to wind speed, wind direction, and the induced velocity at each rotor, and the preferred spin ratio ranges from 1.0 to 3.0 for marine applications (15). To show the interaction effects of the installed four Flettner rotors, the induced flow field for the case study, at Vs = 13.5 knots and Vt = 6.5 m/s, is illustrated in Fig. 5.
Figure. 6 show polar plots of the power delivered by the Flettner rotor, respectively, which depend on the ship speed and the wind velocity. To be more precise: each graph shows ten different curves of the power as a function of the angle between the ships course and the true wind velocity. As the power is symmetric between positive and negative angles, and hence results identical, the ve curves corresponding to a ship speed of 12 knot m/s (11.7 knots) are only plotted in the range from 0 to 360.
Figure 6 Power delivered from the Flettner rotor as a function of the true wind angle for a ship course of 00as indicated by the arrow
The ve curves differ in wind speed: the innermost is for a wind speed of 5 m/s (9.7 knots) and the outermost is for a wind speed of 15 m/s (29.2 knots). The arrow represents the course of the ship. For a straight tailwind, the distance of the curve from the origin at 0 represents the power delivered to
the ship. At a ship speed of 10 m/s, and a true wind speed of 5 m/s, the apparent wind is a headwind, and in this case, the kite delivers no power to the ship. For this reason, there are only four curves in the bottom of the kite diagram, corresponding to
wind speeds, of 15, 12.5, 10, and 7.5 m/s, from the outside to the inside.
(a)
(b)
Figure 6 Visualization of vector (a) and streamline (b) velocity of fluids
(a)
Thrust
(b) Trust of Flettner
Figure 7 Visualization of contour velocity of fluids (a) low; (b) pressure
thrust
-
CONCLUSION
Numerical performance models of two wind power technologies, Flettner rotors, and kites, are combined with wind velocity data along ve shipping routes in a methodology for assessing the wind power input. The average power contribution from the Flettner rotor on the analyzed routes ranges from 193 kW to 373 kW. On the route between Varberg and Gillingham, tting three Flettner rotors on a typical 6500 DWT, slow-steaming general container could provide more than half of the required main engine power. Such a market could be a natural starting point for more detailed studies to establish the case for the Flettner rotor.
This paper shows that, although the transient power contribution is too low and variable for the industry to consider wind as the sole driver of ships typically serving the selected routes, wind can in some cases provide a major share of required propulsive power. The next steps needed in order to tap into this potential are highlighted, including modeling of the implementation of a kite and a Flettner rotor on a particular vessel with a specic pattern of operations. These studies will have to account for practical barriers and the complex integration of thrust contributions from both the main engine and the wind power technology to estimate fuel savings.
REFERENCES
-
Enercon Wind Company, Enercon E-ship 1: a wind-hybrid commercial cargo ship, in Proceedings of the 4th Conference on Ship Efficiency, Hamburg, Germany, Septeber 2013.
-
E. G. Reid, Tests of rotating cylinders, Techinical Notes NACA 209, 1924.
-
A. Thom, Effects of discs on the air forces on a rotating cylinder, Reports & Memoranda 1623, Aerospace Research Council, 1934.
-
J. Seifert, A review of the Magnus effect in aeronautics, Progress in Aerospace Sciences,vol.55,pp.1745,2012.
-
L. Da-Qing, M. Leer-Andersen, and B. Allenstr, Performance and vortex formation of Flettner rotors at high Reynolds numbers, in Proceedings of 29th Symposium on Naval Hydrodnamics,
Gothenburg, Sweden, August 2012
-
A. da Rosa, Wind Energy, in Fundamentals of Renewable Energy Processes, Elsevier, 2013, pp. 685763.
-
L. Prandtl, The Magnus effect and wind-powered ships, Naturwissenschaften,vol.13,pp.17871806,1925.
-
Tillig F, Ringsberg JW (2020) Design, operation and analysis of wind- assisted cargo ships. Ocean Eng 211:107603
-
J. H. Ferziger and M. Peric, Computational Methods for Fluid Dynamics. 2002.
-
F. R. Menter and T. Esch, Elements of Industrial Heat Transfer Predictions, 16th Braz. Congr. Mech. Eng., 2001.
-
J. E. Bardina, P. G. Huang, and T. J. Coakley, Turbulence Modeling Validation, Testing, and Development, Nasa Tech. Memo., 1997, doi: 10.2514/6.1997-2121.
-
T. J. Chung, Computational Fluid Dynamics Cambridge: Cambridge University Press, 2010.
..