
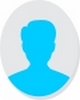
- Open Access
- Authors : Rania Gomaa
- Paper ID : IJERTV9IS080344
- Volume & Issue : Volume 09, Issue 08 (August 2020)
- Published (First Online): 19-09-2020
- ISSN (Online) : 2278-0181
- Publisher Name : IJERT
- License:
This work is licensed under a Creative Commons Attribution 4.0 International License
Coexistence Study of 2.4 GHZ Wireless Technologies for Nuclear and Radiological Applications
(Coexistence Study of 2.4 GHZ for Nucl.& Rad. App.)
Rania Gomaa, QA/QC Department ENRRA, Cairo, Egypt
AbstractWireless technologies continue rapidly to be adopted by nuclear and radiological applications, remote maintenance, radiation dose monitoring, equipment condition monitoring telecommunications, and unintended monitoring for safeguard are the most common uses. It provides reliable and cost-effective approaches for design, development, qualification, implementation, and operation, maintenance, and monitor hazardous and inaccessible areas. But wireless sensors still face common challenges to be licensed especially in the instrument and control systems nuclear facilities as complexity, reliability, cybersecurity, and interference. This work presents the result of an experimental study on the interference and the performance of wireless networks and coexistence among the technologies operate in the 2.4 GHz ISM band that has a higher potential for the nuclear industry. The coexistence test methodology is introduced to evaluate the interference and support the planning for the safe exclusive zone to deploy the wireless network without introducing any vulnerability to the nuclear or radiological facility
Keywordscomponent; Wireless sensor networks; 2.4 GHz ISM band; Electromagnetic Interference; Radio Frequency Interference; and Coexistenc.
-
INTRODUCTION
The Wireless Personal Area Network (WPAN) technology based on the IEEE 802.11, IEEE 802.15.1 IEEE 802.15.4, and other standards are very promising for the nuclear industry due to several characteristics as mobility, reliability, security, scalability, and compatibility with other communication networks technologies. It provides good low- cost solutions to the problem of industrial wiring to prevent the electrical surges around heavy equipment due to field wiring. Digital, wireless Instrument and Control (I&C) devices use specific, standardized wireless communication protocols for transmission of the field signals via radio waves. The expected cost reduction of cables during installation and maintenance is the main trigger for wireless applications in the nuclear industry [1]. The nuclear industry continually finds new areas for industrial measurements and controls that leverage wireless devices. However, wireless technology may exhibit vulnerability to the operating nuclear power plant Electromagnetic Interference/ Radio Frequency Interference (EMI/RFI) environment [2]. Nuclear and radiological facilities as nuclear power plant, cyclotron and radioisotope production facilities have complex systems
composed of large steel tanks, enclosures, containment, piping, valves, motors, pumps, ventilation, etc. All of the materials for these types of structures, systems, and components are characterized by high reverberant radio frequency (RF) environments, which cause multi-path interference in RF signals. Also, RF wireless devices are subjected to electromagnetic interference in the NPPs due to different sources. Electric devices, motor controllers, digital equipment, and radio communication devices are obvious sources of electromagnetic interference, which is a negative environment against wireless communication. The use of wireless technology also brings problems of security and privacy to industrial networks. Encryption standards and network architectural design can provide for secure and reliable transmission of data. Such approaches are accepted in other industries for the communication of highly sensitive information. Methods similar to those used by military communication systems may be required to satisfy security requirements. IAEA is supporting the use of wireless technology for safeguard equipment and accident monitoring, but with consideration to raised challenges (e.g. failure of communications, corruption of data, and security of transmitted data) that need to be taken into account [3] [4].The existing coexistence studies provide limited analysis of the effect of interference in the frequencies of the ISM band. Furthermore, the results obtained are rather inconclusive for the nuclear and radiological applications. So, we try and bridge this gap, we set out to perform a systematic methodology and experimental study of the coexistence considering the specific requirements of a hazardous environment. The coexistence between IEEE 802.15.4 and IEEE 802.11, and BLE (Bluetooth Low Energy) is still under investigation. It was reported that the Packet Error Rate (PER) of IEEE 802.15.4 drops due to IEEE 802.11 interference aggressively if compared with the drops due to Bluetooth interference [6]. Moreover, IEEE 802.15.4 is more affected by the distance to the IEEE 802.11 interferer than Bluetooth, which directly affects the communication reliability in the form of the Received Signal Strength Indicator (RSSI). Remote maintenance and online predictive maintenance programs based on wireless data collection technologies have on-going efforts in the nuclear and radiological facilities. It supports the preventive maintenance gaols in form of schedule-based or event-based type, to
enhance the machine lifetime using failure statistics from similar or exact components. Preventive maintenance could only be effective if the statistical data on worst-case failures is available early enough which not the case with wired monitoring devices. But using commercial wireless devices with vast communication technology, especially, for vibration monitoring equipment and preventive maintenance purposes makes it possible [5].
In general, the Electromagnetic Compatibility (EC) is an important element in the qualification program of the Instrument and Control Systems (I&C) of nuclear facilities. The number of regulatory requirements and industry standards in this area [6] [7]. EPRI publication Guidelines for Electromagnetic Interference Testing of Power Plant Equipment is the recognized guide for electromagnetic compatibility (EMC) in NPP, as it helps establish the safe location of the transmitter relative to its power and distance from sensitive equipment. By using the mathematical approach, a safe exclusive zone can be determined for the WSN. It specifies where the transmitter can be located at a safe distance were the electromagnetic field falls off per the equation:
Pt= effective radiated power of the EMI/RFI transmitter (watt)
Gt= the gain of the EMI/RFI transmitter
E=the allowable radiated electric field strength of the EMI/RFI transmitter at a point (volt/meter)
-
PHYSICAL LAYER PROTOCOLS
A Personal Area Network (PAN) is defined as the immediate space surrounding a device, usually confined to a single way or point to point connection with a maximum range of 10 m. A Local Area Network (LAN) is an expansion of a PAN that delivers service to several devices. To apply WSNs successfully, it is necessary to select appropriate wireless protocols for the various layers of the network protocol stack; PHYsical (PHY), Medium Access Control (MAC), and NetWorK (NWK) layers. Although several protocols have been developed, only a few have been successful and have become the standards for the WSNs. The popular and commercially available wireless protocols for WSN include IEEE 802.11, IEEE 802.15.1, and IEEE
802.15.4 [8].
The standards support the number of frequency within the
2.4 GHz ISM band. Fig. 1 shows the channel classifications of the IEEE 802.11 and IEEE 802.15.4 standards in the 2.4 GHz frequency spectrum. The 802.11b/g standards use a 20 MHz transmission bandwidth, whileBLE uses a narrow 2MHz transmission band. The figure shows that the 1, 6, and
13 WLAN channels significantly interfere with 802.15.4 channels, and each WLAN channel interferes with BLE channels.
IEEE 802.11 (WiFi) networking technology used for WiFi wireless local area networking, it has three channels in the
ISM band and data rate of 54Kb/s. The IEEE 802.11 protocols require high bit rates and energy more than the acceptable in the low bit rate sensor network application [8]. Another drawback of IEEE 802.11 is the relatively high cost and limited scalability features.
Fig. 1 2.4 GHz ISM band: IEEE 802.15.4, IEEE802.15.1 and IEEE
802.11 channels2.4 GHz ISM band: IEEE 802.15.4, BLE and IEEE
802.11 channels
IEEE 802.15.1 (Bluetooth) radio uses a low-powered transceiver that uses 79 channels operating in the 2.4GHz ISM band. It uses the spread spectrum, frequency hopping, and full-duplex signal at a nominal rate of 1600 hops/sec. Adaptive frequency hopping (AFH) capability and data rate supported is up to 3 Mbps It is a major application, the connection of devices to a personal computer or mobile phone. As soon the data transmission started it continuously changes the frequencies until the data reaches its destination. Hence it has fewer effects of interference from WiFi. But its disadvantage is the higher energy consumed with less coverage area when compared to ZigBee nodes.
IEEE 802.15.4 (ZigBee) suitable for WSNs that need low cost, low power consumption, low to medium data rate and scalability, which is suitable for the industrial and monitoring system They are all based on the IEEE 802.15.4 physical layer. IEEE 802.15.4 PHY model is based 2.4 GHz PHY employs O-QPSK modulation and Direct Sequence Spread Spectrum (DSSS) with 2 MHz, chip rate 2000 kc/s, bit rate 250 kb/s and a codebook of 16 symbols [9].
In the case of cooperative coexistence, all the ISM band technologies especially WiFi, Bluetooth, and ZigBee coordinate spectrum usage between multiple networks that employ different wireless technologies. Fig. 1 show the overlapping of 2.4 GHz ISM band, the channel disposition is presented comparing the IEEE 802 PHY channels, as we can see some is overlapping between the IEEE standards. In the 2,4GHz band, the spectrum usage is more efficient, it is separate in 16 channels of 2 MHz, and it includes a channel separation of 5MHz to avoid co-channel interference. Also, the PHY layer is responsible for certain tasks: in the first place the activation or deactivation of the radio transceiver. Secondly, the Energy Detection (ED) within the current channel, as well as the Link Quality (LQI) for received
packets, the Clear Channel Assessment (CCA), the low duty cycle, the channel frequency selection, and finally the data transmission and reception. The legacy of the 802.15.4 slandered coding scheme uses pseudo-orthogonal codes with symbols of 4 bits each and encoded as a 32-chip signal. The raw signaling is carried out using Offset Quadrature Phase Shift Keying with Half Sine Shaping at a rate of 2Mchip/s. Consequently, one eightieth rate coding results in a throughput of 250kb/s. The standard specifies that the receiver sensitivity is measured at a PER of 1% of the sent packets [ 9].
-
COEXISTENCE TEST METHOD FOR NUCLEAR AND RADIOLOGICAL FACILITIES
Coexistence between different wireless technologies can be classified into three domains: space, time, and frequency. Coexistence can thus be achieved by meeting identified conditions; as the adequate spacing between the networks, controlled time-sharing of the channel, and adequate frequency separation between the networks. Coexistence mechanisms can be classified into two categories: non- cooperative and cooperative, depending on whether the involved networks operate independently of one another or coordinate their use of the spectrum. In no cooperative coexistence, each network treats the other networks present as interference and performs interference mitigation. In cooperative coexistence, all the networks collaborate and coordinate their use of the spectrum reasonably [10] [11]. For a given application, to propose a coexistence solution first we need to study the effect of interference due to the cross- technology on the performance of the used technologies. And those technologies must co-operate with each other to minimize the generated interference from devices utilizing the ISM band. The nuclear environment is very complex, noisy with limited space and a large number of rotating equipment, at the same time it needs to operate with a high level of safety, security, and reliability which needs a high degree of protection from electromagnetic frequency interference [6]. We introduce a five-step methodology that reflects a systematic mechanism to test the interference and coexistence in the nuclear and radiological facilities, each step results fed into the next one as shown in Figure 2 to achieve the predictive deployment process.
-
Survey the Test Environment
The noise levels of a plant are very important data to verify or estimate the possibilities of EMI/RFI hazards to digital equipment in nuclear power plants. Furthermore, it is a technical basis to ensure that the safety class equipment will be operating properly in its location of the plant. However, in the case of new plant construction, it is impossible to map electromagnetic noise in the power station before an operation. Therefore, it is recommended that similar plants shall be chosen to undergo site survey and the measured data will be used as a reference for EMI qualification for I&C equipment until it is superseded by the site survey data taken after the plants start-up and operation. Although the EMI/RFI test standards address the applicable guidelines and acceptance criteria, tailoring is the best option given to an application. The application criteria
may be derived from operational and engineering analysis on equipment or the environment it is to be installed in. The concept of tailoring is appropriate for application in safety- related systems for nuclear power plants. So, the first step of activity regarding EMI/RFI qualification is to review and analyze the historical electromagnetic ambient data of domestic and other nuclear power plants to justify the applicable criteria for the scheduled new plants.
Fig. 2 Coexistence test method for nuclear and radiological facilities
-
Wireless Link Analysis
Recommended baseline for the wireless functions of the device to investigate worst-case scenario in a reasonable way for coexistence testing, the wireless device transmitter and receiver are separated by maximum operational distance. To increase test repeatability and reproducibility, the PER is to be recorded at the receiver. The Device Under Test (DUT) should be placed on a nonconductive table at a height of 1 m. A more precise method of calculating the maximum distance of operation is determined to produce a 0% PER during operation.
-
Interfering Network Introduction
Introduce the Wireless Interfering Network after the DUT is installed and the baseline is recorded, an interfering wireless network is introduced into the test setup. The primary source of interference for DUT will be Bluetooth, Wifi, or Zigbee as a single network operating with fixed transmits power and throughput. The initial separation distance between the DUT test setup and the wireless interfering network is started by 1
m. A receiver surrounded by a wireless interfering network can experience increased packet collision, i.e., the hidden terminal effect. In contrast, when a transmitter is near an interfering network, the utilization of the available channel is decreased, i.e., the exposed terminal effect [12].
-
Interference and Coexistence Test
Test the wireless function of the DUT, where interference and coexistence testing consists of three primary variables. Changing any of the three variables (power, distance, and
frequency) will affect coexistnce performance. EMI/RFI testing on emissions and susceptibilities is done under the EMI qualification specification identified by the system design documents.
-
Real Deployment.
Performing real deployment on the new plant will be the last step to verify the electromagnetic noise of the newly- built plant and to justify the applied EMI testing criteria based on the review of ambient noise data of the existing power plants in the first step. Periodic monitoring of EMI levels shall be conducted. Generally, to achieve robust deployment of the wireless network with lower cost and free of unreliable behavior, we suggest this deployment procedure for critical application especially; the nuclear facilities. The goal of the method is to trace most of the interference problems by a good allocation of the base station for optimal performance and the nodes to maintain strong radio signals.
-
-
EXPERIMENTAL STUDY
-
Experimental Setup
The sink and end device nodes use the TI CC2530 chips, it supports ZigBee protocol through Z-Stack open source tool provided by TI, ZigBee protocols provide mesh networking features and support IEEE 802.15.4 for wireless communication [10]. CC2530 is supported by the SmartStudio Platform to control the building process of the ZigBee network, It operates in the 2.4 GHz unlicensed radio frequency band with 16 channels. The chip is connected to an antenna with 5 dBi gain (dipole omnidirectional antenna) and is powered by 2 AA batteries. Fig. 1 shows a block diagram of the experimental setup with the sink node and end device node. The DUT has tested indoor scenarios witp00 packets, the Sink, and end nodes placed 2 m apart (LOS) at a height of 120 cm from the ground. The CC2531 is a USB-enabled SOC the ZigBee communication frequencies, it sniffs the transmitted packets over the air and supported through the Smart software from TI to calculate RSSI/LQI (Receive Signal Strength Indicator/ Link Quality Indicator) [10].
Fig. 3 The experimental setup
The setup of the experiment represented in Fig. 3 is installed at Nuclear Regulatory Authority (NRRA) and Science Life Scientific Services (SLSS) where a survey on the environment was collected to represent the background scenario.
The setup of the experiment represented in Fig. 3 is installed at Nuclear Regulatory Authority (NRRA) and Science Life Scientific Services (SLSS) where a survey on the environment was collected to represent the background scenario.
Fig.4 represents a sample result from the peer to peer communication where the received signal strength is measured per packet and averaged for the transmit signal. First, the background experiment is implemented and the readings were collected for three frequency 2205, 2240, and 2475 MHz. for each frequency the test was repeated at different transmitted power (-12, -8,-4, 1,4.5 dBm) to test the optimum communication parameters. Second, the best- transmitted power was selected to perform the co-existence experiment; the test took place for the transmitted power of 1dBm and 4.5 dBm. The co-existence scenario was repeated with WiFi, Bluetooth, and ZigBee interference source at different distances (1, 3, 6, and 9 m).
Fig. 4 Tested parameters
-
Experimental Results
We start with a survey on the test environment, where the RSSI and PER where recorded for the three selected frequencies at different transmitted power at a fixed distance of
2 m apart (LOS). The test was repeated at the two sites ENRRA, and SLSS, where the results where more reliable at the SLSS site. The PER was not affected, while the RSSI was aggressively changed (-75 to -106 dBm). The results show that the worst-case signal strength that affects the communication is with minimum transmitted power (- 12 dBm) and on channel 11 (2405 MHz), where the link is not reliable and RSSI by edge the accepted limit where PER reaches 20%, as shown in Fig.5. According to the IEEE 802.15.4, the reliable link PER 1.1%
Fig. 6 shows the results of the WiFi interference test, where, at transmitted power of 1dBm the PER is affected where 30-40 packet where lost at short interference distances PER 6 m from the WiFi source at the frequency of 2405MHz. The RSSI was reliable and not affected by the distance from the source as the DUT keep the 2 m apart (LOS).
For IEEE 802.15.1 interference, we set up an Ad hoc wireless Bluetooth network between speaker and laptops and use to generate IEEE 802.15.1 traffic with specified parameters. The results in Fig.7 of the coexistence of the IEEE 802.15.4 show reduced interference performance with the IEEE 802.15.1 network. It is the effect of the hops between the channels with a lack of CSMA features.
Fig. 5 Test environmental survey results
The effect of CSMA in IEEE 802.15.4 is evident in the case of IEEE 802.11b interference, where the reliability of IEEE 802.15.4 improves at higher transmitted power and PER drops within range within the interference packet interval.
Fig. 6 WiFi interference source Results (a) 4.5dBm (b) 1dBm
Fig.8 (b) shows the worst-case interference as it refers when the interferers are at the same channel (Ch. 25), resulting in collision of useful signal and full packet. In terms of link distance, the PER was found to be 3.3 % (approximately one third of the communication payload). RSSI was dropped to lower level due to collision too.
FIG. 7 Blue tooth interference source Results (a) 4.5dBm (b) 1dBm
FIG. 8 ZigBee interference source Results (a) 4.5dBm (b) 1dBm
Coexistence results show that the communication performance will decrease drastically if ZigBee nodes are at a close distance (< 6 m) at the same frequency channel. In general Zigbee-based Wireless Sensor Network (WSN) has the features of high bandwidth and typical data rate for monitoring application, with low cost, low power consumption, reasonable indoor coverage, and ability to accommodate a large number of nodes seems to be the good candidate for the system that has been proposed. Planned deployment with practical site survey enhances the proper placement of the nodes and set the amount of infrastructure required to meet the network demands to overcome interference problem in complex environments with high dense of Electromagnetic/ Radio Frequency Interference as nuclear and radiological facilities.
CONCLUSION
In this paper, we evaluate the influence of interference on WSN as well as the effectiveness of the Coexistence test method presented. It described an experimental study of coexistence between IEEE 802.15.4, IEEE 802.15.1and IEEE 802.11b in the unlicensed 2.4 GHz ISM band. First, we performed an analysis and quantified the effect of varying the spatial, temporal, and frequency parameters of the interference on the reliability of the network. We compared the resilience of the PHY layers of the aforementioned technologies against cross-technology interference. Then, we set up experiments with real wireless networks and verified our analytical results. A case-by-case study should be conducted to assure reliable network performance, which can be enhanced by adding low-cost relays.
REFERENCES
-
I.S. Jacobs and C.P. Bean, Fine particles, thin films and exchange anisotropy, in Magnetism, vol. III, G.T. Rado and H. Suhl, Eds. New York: Academic, 1963, pp. 271-350. M H. Laikari Arto & al. Wireless in Nuclear, feasibility study, Energiforsk report, 2018.
-
B.J. Kaldenbach, et al Assessment of Wireless Technologies and Their Application at Nuclear Facilities, NUREG/CR-6882, 2006.
-
IAEA safety standard, "Advanced Surveillance, Diagnostic and Prognostic Techniques in Monitoring Structures, Systems, and Components in Nuclear Power Plants", 2003.
-
IAEA technical publication, Safeguards Techniques and Equipment, 2011.
-
Hashemian, H.M., 2009. Wireless Sensors for Predictive Maintenance of Rotating Equipment in DOEs Research Reactors: Phase I Final Reprt. US Department of Energy Small Business Innovation Research Program, Grant No. DE-FG02- 08ER85004.2009.
-
USNRC, Regulatory Guide 1.180 – Guidelines for Evaluating Electromagnetic and Radio-Frequency Interference in Safety-Related Instrumentation and Control System, 2003.
-
EPRI TR-102323-R1, Guidelines for Electromagnetic Interference Testing in Power Plants, Rev1, 1997.
-
R. G. Garroppo, L. Gazzarrini, S. Giordano, and L. Tavanti, Experimental assessment of the coexistence of WiFi, ZigBee, and Bluetooth devices, IEEE International Symposium on a World of Wireless, Mobile and Multimedia Networks (WoWMoM), pp. 19, 2011.
-
ZigBee White ZigBee Alliance, www.zigbee.org, 2011. [online].
-
S. Silva, T. Fernandes, A. Valente, and A. Moreira, Coexistence and Interference Tests on a Bluetooth Low Energy Front-End, IEEE Science and Information Conference (SAI), pp. 1014 1018, 2014.
-
S. J. Shellhammer, Estimation of Packet Error Rate Caused by Interference using Analytic Techniques – A Coexistence Assurance Methodology, IEEE P802.19 Wireless Coexistence, 2005
-
N. La. Sorte, H. Refai, and S. Rajab, "Developing a Reproducible Non- Line-of- Sight Experimental Setup for Testing Wireless Medical Device Coexistence," IEEE Transactions on Biomedical Engineering, vol. 59, pp. 3221 – 3229, 2013.