
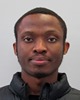
- Open Access
- Authors : Franklin Nkado, Fredrick Nkado
- Paper ID : IJERTV10IS090065
- Volume & Issue : Volume 10, Issue 09 (September 2021)
- Published (First Online): 13-10-2021
- ISSN (Online) : 2278-0181
- Publisher Name : IJERT
- License:
This work is licensed under a Creative Commons Attribution 4.0 International License
Design of an off-Grid Residential Photovoltaic System
Franklin Nkado
Department of Electrical and Electronic Engineering Auckland University of Technology
Auckland, New Zealand
Fredrick Nkado
School of Electrical Engineering Xian Jiaotong University Xian, China
AbstractMost of the households in the Nigerian rural communities are not closely located to the grid, and it is capital intensive to connect them to the grid. Solar energy is considered the best option for providing electricity for these households in rural areas. Solar energy in Nigeria is regarded as one of the most abundant renewable energy resources available due to the country's climatic condition and location. Supplying electricity to remote areas is easier when considering solar energy. This paper presents the needed components and guidelines for designing the least-cost and efficient off-grid photovoltaic (PV) system for a low-energy consumption level residential household in Sokoto state, Nigeria, which has average radiation of 4 7 kWh/m2/day.
Keywords off-grid; photovoltaic system; standard testing condition (STC); solar irradiation.
-
INTRODUCTION
In most developing countries, electricity is not reliable due to the increasing demand for the available supply. In some developing countries, the electricity generation capacity is unable to meet the growing demand of the ever-increasing population. Also, the people living in rural areas have been negatively affected because of inaccessible electricity in their area. The problems mentioned above causes the migration of the rural dwellers to the urban areas. This contributes to factors that make the rural areas remain undeveloped. This rural to urban migration problem can be solved by emphasizing the electricity challenges within their communities [1, 2]. One form of renewable energy which uses solar irradiation to produce electricity is solar energy. It is renewable because the sun can never stop shinning.
Sokoto is a large city in the Northern part of Nigeria located at Longitude 13.1246° N and Latitude 5.1994° E. Due to the towns location, it enjoys higher solar irradiation throughout the year than other parts of the country (see Figure 1). Application of photovoltaics (PVs) has been made cost-effective due to the advanced technology in renewable energy resources. Nigeria power system has been unable to meet the energy demand of the teeming population, yet the solar irradiation potentials in Nigeria is underutilized. This is due to not understanding that this resource can help to improve the Nigerian generation capacity. Energy from fossil fuel has remained the chief source of energy from both the generating companies and individuals and this has negative effect on the atmosphere.
Fig. 1. Global horizontal irradiation in Nigeria [3].
Solar energy is reliable, clean and does not emit carbon dioxide (CO2), which contributes to the reduction of atmospheric pollution. Solar energy has developed rapidly over the years and it is a recognized excellent fossil fuel alternative; however, developing countries have continued to over depend on fossil fuels. They need immediate redirection to solar technologies, which will create alternative energy sources and reduce the price of energy purchase by increasing competition. The high efficiency and capability make solar technology to be tremendously promising, especially in remote areas. Designing and installing an off-grid or grid-tied solar system for rural communities is of great importance due to the optimal access to sunshine [4-6] which can effectively meet the energy demand of the large populace in the area.
The intermittent electricity in developing countries can be improved by implementing photovoltaic (PV) technologies in the generating system. However, for rapid economic and social development, an expert in renewable energy technology is needed to choose the right type of technology. The use of renewable energy technology in rural communities have some setbacks. Some factors that contribute to the challenges, according to [7-10] includes components repairs complication and frequent components breaking down due to lack of acclimatization. The study in [11] divided the challenges into primary and secondary factors. The primary factor is the weather effect on the technology, and the
secondary factor is the technical, economic and socio- political sustainability. These factors can be overcome by training and educating the communities on how renewable energy technologies work before implementing the technology in the communities. Therefore, successfully adopting and deploying photovoltaic technologies in rural communities also needs government support and awareness [3].
-
LITERATURE REVIEW
-
Description of System
One of the most used renewable energy technologies used is Photovoltaic (PV) system. It uses the solar insolation from sun rays that incident on the PV module to generate electricity for the consumers. There are numerous PV technologies which include:
-
Multi-crystalline (or Polycrystalline) silicon contains multiple plates and silicon's crystals grains that form the polycrystalline cells when arranged together. The lifespan is (>25 years), it is durable and cheap to manufacture. They are about 12% less efficient than the monocrystalline silicon cell. It is a mechanical brittle and has low conversion efficiency [12, 13]
-
Monocrystalline silicon: they are produced from pure crystal silicon. It has a conversion efficiency of around 15%, and it is durable (>25%). It is, however, expensive due to the thickness reduction of the cell [12, 13]
-
Thin-film (Amorphous silicon): This type of solar cell is produced using less silicon film on a substrate glass. The lesser silicon film is used than in polycrystalline silicon and monocrystalline silicon. It has a low efficiency of about 6%. The advantages of thin-film silicon include; it can be produced in diverse shapes, flexible, has cell efficiency of 5- 7%, and does not experience overheating that decreases solar cell performance [12].
-
Thin-film (Cadmium Telluride CdTe): it has an efficiency of around 165%. It is well-known because it has a cheaper cost per kW/hr. However, it does not absorb the longer wavelength of light like the silicon cells. It also has a toxic effect on the environment when disposed of [12].
-
Copper Indium Gallium Selenide (CIGS): The efficiency of CIGS is around 20%, which makes it a better option than the thin-film PV cell. Also, it does not contain the toxic (Cd), and it has good resistance to heating [12]. Table 1 shows a summary of the available PV technologies.
Manufacturers
Canadian Solar
UniSolar
Canadian Solar
Model
CS6K280
PVL-136
CS6P-275P
Types of Technology
Monocrystalline
Amorphous Silicon
Polycrystalline
Power
280W
136W
265W
Price
US $215
US $190
US $200
Short-Circuit Voltage
9.42
5.2
9.24
Open Circuit Voltage
38.7
46.3
37.8
Efficiency
17.11%
8.10%
416.47%
NOCT
45± 20C
460C
450C
Manufacturers
Canadian Solar
UniSolar
Canadian Solar
Model
CS6K280
PVL-136
CS6P-275P
Types of Technology
Monocrystalline
Amorphous Silicon
Polycrystalline
Power
280W
136W
265W
Price
US $215
US $190
US $200
Short-Circuit Voltage
9.42
5.2
9.24
Open Circuit Voltage
38.7
46.3
37.8
Efficiency
17.11%
8.10%
416.47%
NOCT
45± 20C
460C
450C
TABLE 1. EVALUATION OF THE EXISTING PV
-
-
Inverter
An inverter is a device that can convert a direct current (DC) to an alternating current (AC) that most home appliances use. For example, the output of solar PV panels is direct current, and an inverter converts this direct current to alternating current, which most household appliances run on. Therefore, there is a need to consider the inverters ability to handle surge electric demand from the load when choosing an inverter for an off-grid design.
-
Battery energy storage system
The battery stores electrical energy as chemical energy and converts the chemical energy to electrical energy when supplying the load [14, 15]. Batteries are rated according to their power and energy capacity. The needed current and voltage required by a specific photovoltaic system design is obtained when batteries are connected in series and parallel. Deep cycle batteries are mostly used for renewable energy systems, and they usually range from 18 to 40 MWh. Some of the batteries used for PV systems are:
-
Lithium-ion (Li-ion): the positive electrode (cathode) consists of lithiated metal oxides (phosphates) and contains the lithium titanate (carbon) in the negative electrode. It has a high energy density and has a cell voltage of around 3.6V to 3.7V [16].
-
Lead-acid battery: A porous material that is dipped in a sulfuric acid electrolyte separates the positive electrode and negative electrode of the lead-acid battery. The positive electrode is made of lead dioxide, and the negative electrode is made of sponge lead [16].
-
Nickel Cadmium (Ni-Cd) battery: Ni-Cd provides reliable services; they have a long life span and are easy to manage [16].
-
Sodium sulphur (NaS) battery: each cell has a positive electrode of molten sulphur and a negative electrode of molten sodium. It has an efficiency of 90% [16].
-
-
System Configuration:
Fig. 2 shows the system configuration of an off-grid system which comprises of solar panel that produces DC electrical power from direct sunlight. Batteries stores the excess DC power produced by the PV panels and supply to the load when there is no sunshine. A charge controller regulates, monitors and controls the energy flowing from the PV array to the inverter and the charge flowing from the battery to the load. An off-grid PV system supplies electricity directly for domestic usage; this system is designed to provide alternating current or direct current to power the household appliances.
Fig 2. Charge controller configuration
-
-
METHOLOGY
The methodology presented in this paper studies the household's energy demand in the rural area of Sokoto state, which does not require a large electrical load. Firstly, the power rating of the electrical devices and the hours of use per day are listed. Solar irradiation data of the study area is obtained from NASA website using the longitude and latitude of the study area.
-
Estimation of Load
Estimation of the load of a typical household in Sokoto state is determined. The detailed analysis of the appliances used in a typical household is shown in table III, the total ampere-hour load (Ah/day), the total AC load power (VA), hours of use per day (duty cycle), efficiency of the battery, wire, and nominal voltage of 48V.
-
Solar Irradiation Data
The study area is located on latitude and longitude of 13.1246° N, 5.1994° E, the solar irradiation data is obtained from NASA website. The summary of the monthly average solar irradiation data in 3 different latitudes is shown in table
II. The peak sun hour (PSH) refers to the solar insolation a location would receive if the sun were shining at its maximum value for a certain number of hours.
-
System Voltage Selection
It is required that the system voltage is adequate to supply the load demand. The system voltage usually increases with the increase in load. The system voltage of a standalone PV system is selected based on the characteristics of the inverter
available. The total AC load power in this design is less than 4000W, 48V system voltage is selected for this design. The peak current when all loads are operational is shown in Table III.
-
Sizing of the Solar Array:
The essential parameters considered in the solar array sizing of the off-grid PV design are the system's voltage, total daily energy in W/hr, and the average daily sun hours. To improve the efficiency of the system design, the total daily average energy consumption will be divided by the product of the component's efficiency, as shown in equation (1). When sizing a system, the efficiency of the battery, the derate factor and temperature losses of the PV must be regarded, and this can be obtained from the product's datasheet.
Er = (1)
In this system's design, the peak sun hour (PSH) of the case study were obtained using the latitude and longitude. The PV temperature loss is considered in this design. A battery efficiency of 100% is difficult to obtain, so the battery efficiency of 90% is usually used in designing the system [11]. The needed voltage and current required by the load is obtained by connecting the PV module in parallel and series. Equations (2) and (3) are used to calculate the needed number of series and parallel panel, considering 48V as the system's voltage.
Np = (2)
NS = (3)
Equation (3) is used to determine the required modules to be connected in series.
Equation (4) is used to obtain the overall number of the module (NT), this is obtained by simply multiplying the number of modules in series (NS) by the total number of modules in parallel (NP).
NT = NS x NP (4)
TABLE II THE DESIGN CURRENT AND THE ARRAY TILT ANGLE
Tilt at latitude -15
Tilt at Latitude
Tilt at latitude +15
Month
Corrected Load (Ah/day)
Peak Sun (Hrs/day)
Design Current (A)
Corrected Load (Ah/day)
Peak Sun (Hrs/day)
Design Current (A)
Corrected Load (Ah/day)
Peak Sun (Hrs/day)
Design Current (A)
J
814.77
4.19
194.46
814.77
4.26
191.26
814.77
4.36
186.87
F
814.77
4.23
192.62
814.77
4.27
190.81
814.77
4.33
188.17
M
814.77
4.22
193.07
814.77
4.21
193.53
814.77
4.23
192.62
A
814.77
4.23
192.62
814.77
4.19
194.46
814.77
4.13
197.28
M
814.77
4.22
193.07
814.77
4.13
197.28
814.77
4.04
201.68
J
814.77
3.86
211.08
814.77
3.77
216.12
814.77
3.70
220.21
J
814.77
4.01
203.19
814.77
3.94
206.80
814.77
3.85
211.63
A
814.77
4.2
193.99
814.77
4.15
196.33
814.77
4.08
199.70
S
814.77
4.3
189.48
814.77
4.3
189.48
814.77
4.31
189.04
O
814.77
4.69
173.73
814.77
4.73
172.26
814.77
4.78
170.45
N
814.77
4.74
171.89
814.77
4.82
169.04
814.77
4.89
166.62
D
814.77
4.4
185.18
814.77
4.4
185.18
814.77
4.60
177.12
TABLE III PEAK CURRENT WHEN ALL LOAD ARE OPERATIONAL
Load
Quantity
Load Current (A)
Load Voltage (V)
AC Load power (VA)
Daily Duty Cycle (hrs/day)
Weekly Duty Cycle (days/wk)
Power Conversion Efficiency (Decimal)
Nominal System Voltage (V)
Ampere hour load (Ah/day)
Lighting bulbs
3
0.222
220
146.52
16
1
0.85
48
57.46
Fan
1
0.227
220
49.94
5
1
0.85
48
6.12
Phone charger
1
0.023
220
5.06
2
1
0.85
48
0.25
Heater
1
10.22
220
2248.40
10
1
0.85
48
551.08
Refrigerator
1
0.568
220
14.96
24
1
0.85
48
73.51
Microwave
1
6.534
220
1437.48
0.75
1
0.85
48
26.42
Laptop charger
1
0.295
220
64.90
2
1
0.85
48
3.18
Electric Iron
1
1.59
220
349.80
0.25
0.286
0.85
48
0.61
Total AC load power (VA)
4427.06
Total Ampere-hour load (Ah/day)
718.63
-
PV Module Selection: Cost of the PV module, the characteristics, the efficiency and the ease of replacing the module are the major factors considered when selecting a PV module. The Canadian solar CS3K-280P-AG is used for this design, considering the factors mentioned above.
-
Inverter Selection: The total AC load power is required to determine the exact capacity of the inverter for the off- grid system. This design has a total AC load of 4427.06 VA, therefore, an inverter of 5kVA is selected. Inverter capacity of 5kVA is chosen because it is higher than the total AC load power.
A Sunsys 5kVA, 48V inverter with a built-in 4kW Maximum Power Point Tracking (MPPT) is selected for this design.
-
Charge controller: The function of a charge controller in a standalone PV system is to protect the battery from over-charging by the array, it keeps the battery at the maximum state of charge, it also protects the battery from over-discharging by the load. The charge controller prevents severe discharging from the battery, which reduces its life span.
-
Battery Bank Sizing: Table XII shows the Battery model, HAIYI, 12V, 450Ah deep cycle was used for the design. This battery model is cheap and readily obtainable in Nigeria; it does not always require cost-saving maintenance. Deep cycle batteries have fast charging ability and discharges slowly. It has a reasonable maximum depth-of-discharge (DOD) in relation to other battery design available. Battery manufactures specify the maximum DOD of a battery. The DOD is defined as the discharged capacity from a fully charged divided by battery's nominal capacity. The DOD of HAIYI battery, as seen in Table XV, is 0.8, as stated by the manufacturer. The total number of batteries required in series and parallel is shown in Table XIII, which is obtained considering the battery's voltage and system voltage. Table XIV shows the calculated useable capacity of the battery in Ah, and this is obtained considering the manufacturer's specified maximum DOD. The batteries required in parallel is 7.54, then approximated to 8, which will adequately provide storage for up to 3 days.
I. PV Solar characteristics: Table IX shows the rated current and voltage of the module; the system voltage is 48V. The Canadian model of CS3K-280P-AG was chosen because of its low-cost, high efficiency when considering the installation location. The largest design current with its corresponding lowest peak sun obtained from different tilt angle, as shown in Table IV. This ensures continuous production of enough electricity even on the days with least peak sun such as on the cloudy days. Table V shows the lowest design current with the corresponding lowest peak sun; this is also used in the design because of the reason mentioned above. Table VI shows the AC load, wire efficiency, voltage of the system and battery efficiency. Tables VIII, IX, X shows the required PV in series. The total PV required is calculated as seen in Table VII considering the manufacturers' information about the module provided as presented in Table XI.
TABLE IV. LARGEST PEAK SUN AND DESIGN CURRENT FROM EACH LATITUDE
Tilt at latitude -15 |
Tilt at latitude |
Tilt at latitude +15 |
|||
Peak Sun (hrs/day) |
Design Current (A) |
Peak Sun (hrs/day) |
Design Current (A) |
Peak Sun (hrs/day) |
Design Current (A) |
3.77 |
216.12 |
3.86 |
211.08 |
3.70 |
220.21 |
TABLE V. THE SMALLEST DESIGN CURRENT AND CORRESPONDING PEAK SUN
Tilt at latitude |
|
Peak Sun (hrs/day) |
Design Current (A) |
3.86 |
211.08 |
TABLE VI. VALUE OF THE PEAK CURRENT LOAD
Total AC load power (W) |
Nominal System Voltage (V) |
Peak Current draw (A) |
Total Amp- hour Load (Ah/day) |
Wire Efficiency factor (Decimal) |
Corrected Amp-hour Load (Ah/day) |
4427.06 |
48 |
92.23 |
718.63 |
0.98 |
814.77 |
TABLE VII. SYSTEM ARRAY ESTIMATION OF THE TOTAL MODULE
Nominal Battery Voltage (V) |
Battery in Series |
Voltage required for the load (V) |
Highest temp. module volt. (V) |
Module in series |
Module in Parallel |
Total module |
12 |
4 |
57.6 |
15 |
2 |
20 |
40 |
TABLE VIII. SYSTEM ARRAY ESTIMATION OF THE MODULE
Design current (A) |
Module derate factor |
Derated design current (A) |
Rated module current (A) |
Module in Parallel |
169.04 |
0.9 |
187.82 |
8.98 |
20.92 |
TABLE IX. A DETAILED DESCRIPTION OF THE NUMBER OF MODULES IN SERIES
Module in series |
Rated module voltage (V) |
Rated array voltage (V) |
2 |
31.2 |
62.4 |
Module open-circuit voltage (V) |
Array open-circuit voltage (V) |
|
37.9 |
75.8 |
TABLE X. A DETAILED DESCRIPTION OF THE NUMBER OF MODULES IN PARALLEL
Modules in parallel |
Rated module voltage (V) |
Rated array voltage (V) |
20 |
8.98 |
179.7 |
Module open-circuit voltage (V) |
Array open-circuit voltage (V) |
|
9.47 |
189.4 |
TABLE XI. CHARACTERISTICS OF CANADIAN SOLAR PV
Make/Model |
Canadian Solar/ CS3K-280P-AG |
Type |
Poly-crystalline |
Rated voltage (V) |
31.2 |
Rated current (A) |
8.98 |
Short circuit current (A) |
9.47 |
Open circuit voltage (V) |
37.9 |
TABLE XII. HAIYI BATTERY SPECIFICATION
Make/Model |
Haiyi/EH4 |
Battery Type |
Deep cycle |
Nominal Voltage (V) |
12 |
Rated capacity (Ah) |
450 |
TABLE XIII. BATTERY SPECIFICATION IN SERIES AND
PARALLEL
Nominal system voltage (V) |
Nominal battery voltage (V) |
Battery in series |
Battery in parallel |
Total batteries |
48 |
12 |
4 |
7 |
28 |
TABLE XIV. SYSTEM BATTERY CAPACITY
Batteries in parallel |
Capacity of selected battery (Ah) |
System battery capacity (Ah) |
Maximum depth of discharge (decimal) |
Usage battery capacity (Ah) |
7 |
450 |
3150 |
0.8 |
25020 |
TABLE XV. SPECIFICATION OF MAXIMUM DEPTH OF DISCHARGE NUMER OF BATTERIES IN PARALLEL
Corrected amp-hour load (Ah/day) |
Storage days |
Maximum depth of discharge (decimal) |
De rate for temperature (decimal) |
Required battery capacity (Ah) |
Batteries in parallel |
814.77 |
3 |
0.8 |
0.9 |
3394.88813 |
7.54 |
TABLE XVI. COST ANALYSIS OF THE COMPONENTS
Analysis |
PV Module |
Battery |
Inverter |
Capital cost |
$190/panel |
$80/kWh |
$150/kW |
Maintenance |
$60/kW/year |
$15/kWh/year |
$0.6/kW/yr |
CONCLUSION
The quantity of solar radiation received by Sokoto state is high, it is estimated at average monthly radiation of about 4-7 kWh/m2/day. This state has a great potential for the off-grid PV system, especially in the residential houses located in the rural areas which are not situated closely to the conventional power grid. The components and guidelines for designing a cost-beneficial off-grid PV system is presented in this paper. The household electrical devices of a typical household in the state's rural area are used in the design of the off-grid PV system. The optimal sizing of the components is ensured to obtain a cost-efficient and reliable system that will meet the load demand of three days.
The 5kW array obtained from the result consists of 20 PV array modules, 28 (12V, 450Ah) batteries and 48V, 5kVA inverter, which has a built-in charge controller. The above- listed components will adequately supply the households in the rural areas if the load demand is within the capacity sated in this paper. The guideline stated in this paper can be adopted by the households in the urban areas. The future work will consider rural households with stochastic loads.
REFERENCES
-
K. Reiche, A. Covarrubias, and E. Martinot, "Expanding electricity access to remote areas: off-grid rural electrification in developing countries," Fuel, vol. 1, no. 1.2, pp. 1-4, 2000.
-
B. Zhao, X. Zhang, P. Li, K. Wang, M. Xue, and C. J. A. E. Wang, "Optimal sizing, operating strategy and operational experience of a stand-alone microgrid on Dongfushan Island," vol. 113, pp. 1656-1666, 2014.
-
M.-L. Barry, H. Steyn, and A. Brent, "Selection of renewable energy technologies for Africa: Eight case studies in Rwanda, Tanzania and Malawi," Renewable Energy, vol. 36, no. 11, pp. 2845-2852, 2011.
-
K. Kaygusuz, "Energy for sustainable development: A case of developing countries," Renewable and Sustainable Energy Reviews, vol. 16, no. 2, pp. 1116-1126, 2012.
-
H. Tazvinga, M. Thopil, P. B. Numbi, and T. Adefarati, "Distributed renewable energy technologies," in Handbook of distributed generation: Springer, 2017, pp. 3-67.
-
E. J. T. E. J. Adhekpukoli, "The democratization of electricity in Nigeria," vol. 31, no. 2, pp. 1-6, 2018.
-
S. Teitel, "On the concept of appropriate technology for less industrialized countries," Technological forecasting and social change, vol. 11, no. 4, pp. 349-369, 1978.
-
S. Singh, M. Singh, S. C. J. E. C. Kaushik, and Management, "Feasibility study of an islanded microgrid in rural area consisting of PV, wind, biomass and battery energy storage system," vol. 128, pp. 178-190, 2016.
-
A. K. Shukla, K. Sudhakar, and P. J. S. E. Baredar, "Design, simulation and economic analysis of standalone roof top solar PV system in India," vol. 136, pp. 437-449, 2016.
-
M. Sharafi and T. Y. J. R. e. ELMekkawy, "Multi-objective optimal design of hybrid renewable energy systems using PSO-simulation based approach," vol. 68, pp. 67-79, 2014.
-
I. Dunmade, "Indicators of sustainability: assessing the suitability of a foreign technology for a developing economy," technology in society, vol. 24, no. 4, pp. 461-471, 2002.
-
F. Huide, Z. Xuxin, M. Lei, Z. Tao, W. Qixing, and S. Hongyuan, "A comparative study on three types of solar utilization technologies for buildings: Photovoltaic, solar thermal and hybrid photovoltaic/thermal systems," Energy Conversion and Management, vol. 140, pp. 1-13, 2017.
-
H. Tian, F. Mancilla-David, K. Ellis, E. Muljadi, and P. J. S. e. Jenkins, "A cell-to-module-to-array detailed model for photovoltaic panels," vol. 86, no. 9, pp. 2695-2706, 2012.
-
T. O. Saetre, O.-M. Midtgård, and G. H. J. R. E. Yordanov, "A new analytical solar cell IV curve model," vol. 36, no. 8, pp. 2171-2176, 2011.
-
A. N. Al-Shamani et al., "Design & sizing of stand-alone solar power systems a house Iraq," pp. 145-150, 2015.
-
P. Manimekalai, R. Harikumar, and S. Raghavan, "An overview of batteries for photovoltaic (PV) systems," International Journal of Computer Applications, vol. 82, no. 12, 2013.