
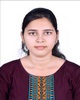
- Open Access
- Authors : Shruti Atkore , Padmaja Sankala , Dr. Parag Chaudhari
- Paper ID : IJERTV11IS080053
- Volume & Issue : Volume 11, Issue 08 (August 2022)
- Published (First Online): 19-08-2022
- ISSN (Online) : 2278-0181
- Publisher Name : IJERT
- License:
This work is licensed under a Creative Commons Attribution 4.0 International License
Design of Supercapacitor Storage Bank for Fast Charging using Single Phase Matrix Converter
Shruti Atkore Department of Electrical Engineering AISSMS COE Pune,India
Prof. Padmaja Sankala Department of Electrical Engineering AISSMS COE Pune,India
Dr. Parag Chaudhari
Scientist F DRDO Pune, India
Abstract This study considers a top converter based single- phase matrix converter (SPMC) topologies for the supercapacitor charging process. Pulse Width Modulation (PWM) is used to synthesize the output from an AC source in a single topology that can perform all the functions of a generalised single-phase converter. The circuit requires four antiparallel common emitters integrated bipolar transistors for bidirectional switching (IGBTs). The switching sequence employed to avoid voltage peaks at the switches enables a safe commutation procedure. Simulations were done in the MATLAB/Simulink environment to examine the SPMC behaviour.
Keywords: AC-AC Conversion, Ideal switches, SPMC, SPWM.
-
INTRODUCTION
Supercapacitors, sometimes known as ultracapacitors, are being employed increasingly often in a variety of applications due to their advantages over batteries. Since supercapacitors function on electrostatic principles and don't include any chemical reactions, they avoid the longevity issues related to chemical storage in batteries. They can withstand millions of charge/discharge cycles and have life spans of up to 20 years, making them orders of magnitude more resilient than batteries. They can charge and discharge quicklyin the range of secondsthanks to their low impedance. Because of this and their typical ability to hold a charge for a long time, and discharge cycles. They are also used in conjunction with batteries in situations where rapid surges in power output are needed during load changes. In terms of power density, cycle life, and other factors, supercapacitors could be more beneficial. To reach their full potential, they also require more difficult answers. A few of the designs that must be taken into consideration include managing super capacitor-discharge, optimising supercapacitor charging, and, in the case of series arrangements of super capacitor modules, maintaining effective voltage balancing between cells. Because of its failure modes, supercapacitor systems might be challenging to size for end-of-life. Understanding how voltage and temperature effect failure is never a "one size fits all" calculation since supercapacitors are significantly more sophisticated than normal board-level capacitors.
For example, the amount of energy that may be used in a particular class of applications may be constrained by the voltage discharge characteristics of supercapacitors. The internal equivalent series resistance (ESR) of a supercapacitor result in a nonlinear voltage decrease upon discharge in a constant power condition. As a supercapacitor is depleted under constant current circumstances, its voltage declines essentially linearly over time. The voltage
decreases more quickly for discharges with increasing power. In high-current pulse applications, the resistive component may result in internal device heating and reduce the useful life of the super capacitor. The lifespan of supercapacitors is significantly impacted by the evaporation of the electrolyte. Operating at a high voltage or temperature might speed up electrolyte evaporation.
The necessary literature review is carried out as under: This study presents a unified Hamiltonian formulation for DER control in ac single-phase microgrids (SP-MG) using the proportional-integral passivity-based control (PI-PBC) and interconnection and damping assignment passivity- based control (PI-PBC) passivity-based control approaches (IDA-PBC). We may take into account both pulse-width modulated voltage source converters (PWM-VSC) and pulse-width modulated current source converters using the provided Hamiltonian framework (PWM-CSC).[1]
Self-charging supercapacitor power cells (SCSPC), which can generate, transform, and store energy in a single integrated energy device, are of tremendous research interest. The use of liquid electrolytes in SCSPC reduces the efficiency of mechanical to electrical energy conversion, prompting the creation of alternative electrolyte systems.[2]
For harmonic transient simulation, this study recommends a model of a stand-alone PV system with a hybrid energy storage system made of batteries and supercapacitors. This paper work model is based on a recently developed harmonic domain modelling method known as flexible extended harmonic domain modelling (FEHD) [3].
SCSPC are receiving more attention in design and development due to its ability to convert and store energy in a single integrated device. Here, we demonstrate how to make a self-charging supercapacitor using siloxane sheet electrodes and a polymeric siloxane separator that is immobilised with an ion gel electrolyte.[4]
Recent advancements in power electronics and the growing adoption of renewable producing and energy storage technologies have largely contributed to the conventional passive operation of electric distribution networks beginning to give way to an active operation. [5,6].
Wide-scale power networks to microgrids using alternating- current (ac) or direct-current (dc) paradigms are only a few examples of scenarios where this shift can be observed.
Power electronic converters are crucial for the effective operation and control of renewable energy producing and energy storage systems, regardless of the design of the power grid. The paradigm shift in power systems includes single-phase microgrids (SP-MG). [7-9].
Pulse-width modulated voltage-source converter (PWM- VSC) and pulse-width modulated current source converter are two types of power electronic converters that are often employed in the integration of distributed energy resources (DER) (PWM-CSC). For the integration of energy storage systems (like batteries and
supercapacitors) and renewable generating systems (like photovoltaic (PV) and wind generation), the former is by far the most popular type of converter, whereas the latter is used in applications that require control of the dc current, like some wind energy systems and superconducting magnetic energy storage systems.[10].
-
SYSTEM REQUIREMENT
The necessity of charging Supercapacitor:
Because of their intricate design, which includes numerous holes of varied sizes in activated carbon particles and an equally intricate circuit architecture, supercapacitor electrodes are not always easy to charge. A supercapacitor charger frequently has to generate huge currents and operate over a wider voltage range in comparison to a "equivalent" battery charger. Various billing methods are available, depending on the requirements of the application.
-
Continuous current
-
Stable voltage
-
Continuous voltage/Continuous current (CICV)
-
Continuous power
-
Connection to a power source in parallel
One common control approach is constant current/constant voltage. At the beginning of the charge cycle, the supercapacitor receives a constant current from the charging apparatus, which causes the capacitor's voltage to grow linearly. The constant voltage loop engages once the supercapacitor reaches the correct voltage and accurately controls the supercapacitor charge level to maintain stability and avoid overcharging.
Fig1. Control of CICV supercapacitor charging.
Fig 2. Collaborative UPS Line System
They are bidirectional convrters. If there is AC voltage, the transistor full-bridge converter functions as a rectifier and the half-bridge inverter functions as a step-down regulator for charging of the battery. Topology is the existence of a dc bus voltage, which needs to be at least 325 V in amplitude in order to generate an output Voltage level of 230 V. The capacitor must therefore be substantial. Another issue is the energy dual conversion. These problems can be solved by using matrix converters. For AC-AC conversion, matrix converters are a well-known "fully – featured" substitute for reactive components.
It was the first to commercially realize and introduce the SPMC. The only application of this method was for AC-AC conversion, though. In the future, single-phase matrix converters will gain popularity. They served as buck and boost converters, voltage inverters, rectifiers, and rectifiers PFC.
Fig 3. Single Phase Matrix Converter
Figure 3 displays the SPMC topology. Bi-directional switches make up the whole bridge inverter that it symbolizes. Figure 4 shows a bidirectional switch. Typically, two transistors connected in series and facing opposing directions make up this device.
Figure 3 presents the SPMC topology. It is a full bridge inverter made up of switches that can move both ways. Figure 4 shows a switch that is bidirectional. Typically, two transistors are connected in series with each other in the opposite direction.
Fig 4. Bidirectional Switch
Although SPMC offers numerous benefits, it also has one big drawback: Over the energy storage voltage, it is impossible to raise the output AC voltage amplitude.
-
-
MATHEMATICAL MODELLING
The SPMC consist four ideal bidirectional switches. In that switches are Sw1, Sw2, Sw3, Sw4 and source input is coupled to the load. Where p is the forward direction and q is the reverse direction of each switch . For positive half cycle switches Sw1p, Sw4p, Sw2q, Sw3q conduct as shown
in the fig 8 and fig 10. For the negative half cycle Sw1q, Sw4q, Sw2p, and Sw3p conducts as shown in fig 9 and fig 11.
Every switch is capable of allowing current to flow bi- directionally while blocking forward and reverse voltages.
Given equations (1) and (2) gives the input and output voltage of SPMC and respectively and with loads represented in equation (3)
() = 2 (1)
() = 2 (2)
() = () + (3)
The essential output voltage component is determined by,
= (4)
Where, output frequency , the modulation frequency and is the input frequency.
-
ARCHITECTURE OF SYSTEM
Fig 5. Plan for employing a SPMC to quickly charge a bank of supercapacitors
Single Phase Matrix Converter topology:
This topology connects the supply input to the load at the intersections of a matrix of input and output lines using four bi-directional switches, as shown in Figure 6. The SPMC consists of four bidirectional switches, Sw1 to Sw4, with a current flow direction of "p" or "q" (ahead direction for "p" and opposite direction for "q").
Fig 6. Circuit configuration
Each switch can conduct current in both directions in addition to being able to block voltage. Figure 6 substitutes a common emitter anti-parallel IGBT with two diodes for a bidirectional switch module.
The diodes allow for reverse blocking of the switch module. IGBTs were selected because they have a high switching and
current carrying capability, making them perfect for high power applications.
Fig 7. Bidirectional switch
The four bidirectional switches Sw1 to Sw4's switching angle, "p" or "q," corresponds to drivers one and two, respectively, based on the following criteria:
At any time t, any two of the switches below will be turned ON:
-
Sw1p and Sw4p will control the current flow during the input supply's positive cycle. (mode 1)
-
Sw1q and Sw4q will conduct the current flow during the input supplys negative cycle. (mode 2)
-
Sw2q and Sw3q will control the current flow during the input supply's positive cycle. (mode 3)
-
Sw2p and Sw3p will control the current flow during the input supply's negative cycle. (mode 4) Figures 8 to 11 show the same:
Fig 8. Mode 1- +ve Cycle
Fig 9. Mode 2 – ve Cycle
Figure 10. Mode 3- +ve Cycle
Fig 11. Mode 4- -ve Cycle
Sinusoidal Pulse Width Modulation:
Figure 12. shows the popular SPWM used in power electronics. In order to achieve, with a sinusoidal reference signal (Vref ) a high frequency triangular carrier signal of the desired frequency (Vc) is contrasted. The crossover points are used to determine the switching instants. [9-10]
Fig 12. SPWM (Single phase pulse width modulation)
The carrier signal (Vc) and reference signal's difference in magnitude is what determines the modulation index (Vref). Mi has an inverse relationship with the size of the fundamental component of the output voltage. The Vc amplitude of the triangle signal is normally held constant. The modulation index can be changed to control the output voltage, though. [10-12]
-
-
SIMULATION RESULT AND DISCUSSION
Fig 13. (a) SIMULINK model of SPMC
(b) Output Waveform
The suggested control paradigm is verified through simulation using MATLAB/SIMULINK, as shown in Figure 13 (a). MATLAB/Sim SIMULINK's Power System Block set (PSB) is used to model and simulate the circuit. Fig 13 (b) results are obtained using simulation techniques associated with SPMC at 50Hz frequency.
-
CONCLUSION
In this study, a matrix converter-based supercapacitor charger is discussed. The topology of the converters is explained. The ability of appropriate switching techniques, where IGBTs are utilised as the primary power switching device, to imitate and identify the SPMC has been demonstrated. This work illustrates how the frequency of the input supply can be changed using an SPMC with bidirectional switches. By using Single-Phase Matrix Converter time required to charge the super-capacitor bank (24 V) is 400 sec to attain 90% SOC and the % voltage ripple with filter is ± 0.65.
REFERENCES
[1] Montoya, O. D., Gil-González, W., & Garces, A. (2019). Distributed energy resources integration in single-phase microgrids: An application of IDA-PBC and PI-PBC approaches. International Journal of Electrical Power & Energy Systems, 112, 221-231. [2] Manoharan, S., Pazhamalai, P., Mariappan, V. K., Murugesan, K., Subramanian, S., Krishnamoorthy, K., & Kim, S. J. (2021). Proton conducting solid electrolyte-piezoelectric PVDF hybrids: Novel bifunctional separator for self-charging supercapacitor power cell. Nano Energy, 83, 105753. [3] Vargas, U., Lazaroiu, G. C., Tironi, E., & Ramirez, A. (2019). Harmonic modeling and simulation of a stand-alone photovoltaic- battery-supercapacitor hybrid system. International Journal of Electrical Power & Energy Systems, 105, 70-78. [4] Krishnamoorthy, K., Pazhamalai, P., Mariappan, V. K., Nardekar,S. S., Sahoo, S., & Kim, S. J. (2020). Probing the energy conversion process in piezoelectric-driven electrochemical self- charging supercapacitor power cell using piezoelectrochemical spectroscopy. Nature communications, 11(1), 1-11.
[5] Krishnamoorthy, K., Pazhamalai, P., Mariappan, V. K., Nardekar,S. S., Sahoo, S., & Kim, S. J. (2020). Probing the energy conversion process in piezoelectric-driven electrochemical self-
charging supercapacitor power cell using piezoelectrochemical spectroscopy. Nature communications, 11(1), 1-11.
[6] Montoya, O. D., Garces, A., & Espinosa-Perez, G. (2018). A generalized passivity-based control approach for power compensation in distribution systems using electrical energy storage systems. Journal of Energy Storage, 16, 259-268. [7 Ortega, A., & Milano, F. (2015). Generalized model of VSC-based energy storage systems for transient stability analysis. IEEE transactions on Power Systems, 31(5), 3369-3380. [8] Avila-Becerril, S., Montoya, O. D., Espinosa-Pérez, G., & Garcés,A. (2018). Control of a detailed model of microgrids from a hamiltonian approach. IFAC-PapersOnLine, 51(3), 187-192.
[9] Montoya, O. D., Ruiz, A. G., Serra, F. M., & Magaldi, G. (2018, February). Apparent power control in single-phase grids using SCES devices: An IDA-PBC approach. In 2018 IEEE 9th Latin American Symposium on Circuits & Systems (LASCAS) (pp. 1-4). IEEE. [10] Montoya, O. D., Gil-González, W., Garcés, A., & Espinosa-Pérez,G. (2018). Indirect IDA-PBC for active and reactive power support in distribution networks using SMES systems with PWM- CSC. Journal of Energy Storage, 17, 261-271.
[11] Jaiswal, J. L., Biswas, A., & Agarwal, V. (2012, December). Implementation of Single-phase matrix converter as generalized single phase converter. In 2012 2nd International Conference on Power, Control and Embedded Systems (pp. 1-6). IEEE. [12] Baharom, R., Rahman, N. F. A., Hidayat, M. N., Muhammad, K. S., Seroji, M. N., & Yahaya, N. Z. (2020). A new gate drive for a single-phase matrix converter. International Journal of Power Electronics and Drive Systems, 11(2), 823. [13] Burhanudin, J., Hasim, A. S. A., Ishak, A. M., Fairuz, S. M., Dardin, S. M., Azid, A. A., & Burhanudin, J. (2022, March). Simulation of AC/AC Converter using Single Phase Matrix Converter for Wave Energy Converter. In 2022 IEEE International Conference in Power Engineering Application (ICPEA) (pp. 1-6). IEEE. [14] Rahimi Baharom, A. M. A. (2019). Development of four quadrant operation of DC-to-DC converter using single phase matrix converter. Indonesian Journal of Electrical Engineering and Computer Science, 16(3), 1249-1256. [15] Boydak, M., Orhan, A., & Çalkan, A. (2020, June). Multimode Operation of Single-Phase Matrix Converter with Safe Commutation Strategy. In 2020 International Conference on Electrical, Communication, and Computer Engineering (ICECCE) (pp. 1-5). IEEE.