
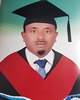
- Open Access
- Authors : Dereje Girma
- Paper ID : IJERTV9IS050602
- Volume & Issue : Volume 09, Issue 05 (May 2020)
- Published (First Online): 27-05-2020
- ISSN (Online) : 2278-0181
- Publisher Name : IJERT
- License:
This work is licensed under a Creative Commons Attribution 4.0 International License
Effect of Land use Types on Selected Soil Physical and Chemical Properties at Sire Morose Sub Watershed, Central Highland of Ethiopia
1* Dereje Girma
Oromia Agricultural Research Institute, Fitche Agricultural Research Center
Abstract:- Information about effects of different land use types on soil physical and chemical properties is crucial for best land management practices. Therefore, this study was conducted to investigate the effect of land use type on selected soil physical and chemical properties at sire morose sub watershed Hidbu abote district Ethiopia. Three land use types were selected from the sub watershed (Forest, grazing and cultivated land). Undisturbed core and disturbed composite soil samples were collected randomly from three sites with three replications from each land use type at two varying depths (0-20 cm and 20-40 cm) and subjected to laboratory soil analysis. Accordingly, the highest mean value sand and clay were recorded in the surface soil of grazing and cultivated land respectively. The mean bulk density of the soils ranged from 0.94 to1.4g cm-3 and the mean total porosity ranged from 52.95 to 64.53%, which indicate good soil structure. The pH ranged from 5.9 to 6.19 while the mean value of OM range from 1.03 to 5.2%. However, the mean value of total N range from 0.09 to 0.25%. The mean value of available P ranged from 17.67 to 24.8 mg/kg, which implies high available P in the study area. The exchangeable basic cations and CEC values were within medium to high ranges in all land use types. Most of the physical and chemical properties of the soils of the study area were distinctly influenced by the different land use types.
Keywords: Cultivated , forest , grazing land , Soil physicochemical properties
INTRODUCTION
Rapid increase in population demands more production of food, fodder, fiber and fuel from the land. To meet these needs, vast tracts of land are being put under intensive cultivation and large areas of grazing lands are being overgrazed and degraded in Ethiopia. The highlands of Ethiopia, with altitude above 1500 meters above sea level (m.a.s.l), are the dominant sources of water, crop production, and fodder and hence are densely populated and hold about 90% human and about two-thirds of the countrys livestock population. The highlands cover about 50% of the land area and 95% of the cropping land accounting for over 90% of the countrys economy [20]. Additionally new and often marginal lands are being brought into production. Land must be carefully managed, if its productivity is to be maintained or increased and, it appears to be imperative to establish land use system that allow for the demands of increasing population while conserving the soil fertility in the long term.
Soil resources are finite, non-renewable, and prone to degradation through misuse and mismanagement. In
addition, intensive agriculture, combined with dynamic population pressure and long-term exploitative farm practices, have led to the continuous depletion of the natural vegetation cover and over-utilization of land resources [30]. Moreover, due to an increasing demand for firewood, timber, pasture, food, and residential construction, the hardwood forests are being degraded or converted to cropland at an alarming rate in the study area. Under such situations, soil degradation is inevitable [42].
Land degradation encompasses the whole environment including individual factors such as soils, water resources (surface and ground), forests (woodlands), grasslands (rangelands), croplands (rain fed and irrigated) and biodiversity (animals, vegetative cover ) [15] Different studies have examined the effects of land use/ cover change on soil physicochemical properties, and most concur that despite its varying consequences land use change frequently leads to nutrient losses and reduction of organic matter inputs in the soil [21]. Conversion of natural forest to other forms of land uses such as farmlands and pasturelands can provoke soil erosion and lead to a reduction in soil nutrients and modification of soil structure [32]. [40] also concur that change in land use types negatively affects soil productivity characteristics such as soil bulk density and hydraulic conductivity. Cultivation of forests for instance can diminish soil carbon (C) within a few years of initial conversion [35] and substantially lower mineralizable nitrogen (N) [41]. [25] reported an increase in bulk density and a reduction in porosity and aggregate stability following the conversion of forest land to crop land, with consequent degradation of adjacent aquatic system
In Ethiopia, rapid population growth and environmental factors lead to the conversion of natural forestland and grassland into cultivated farmland [48]. Such land use changes have contributed to soil degradation and soil loss by deteriorating the soil physical and chemical properties [27]. Soil compaction, the loss of soil structure, soil organic matter (SOM) degradation, undulating terrain, highly erosive rainfall, and inappropriate farming practices make soil highly vulnerable to erosion. Soil erosion from cropland (42 Mt ha-1 average annual rate) is higher compared with 5 Mt ha-1 from grazing land [6]. Soil degradation causes the loss of fertile topsoil and reduces the productivity of the land. The country loses an estimated
1 billion USD per year from both on-site and off-site changes.
The conversion of native forest and native rangeland into cultivated land is known to deteriorate soil properties [34]. These authors reported increment of bulk density, organic matter deterioration, and reduction in cation exchange capacity (CEC), which in turn reduce the fertility status of the given soils, as the main impacts. Similarly, conversion of natural forest land into grazing and cultivated lands caused losses of soil cation exchange capacity (CEC) in the magnitude of 38 and 50%; respectively, in the surface (0- 20 cm) [37]. Success in soil management to maintain the soil quality, therefore, depends on the understanding of the effect of land use change and types on soil properties over time.
Land use changes have altered the characteristics of the Earths surface; leading to changes in soil physicochemical properties [37]. Recent interest in evaluating the quality of soil resources has been stimulated by increasing awareness that soil is critically important component of the earths biosphere, functioning not only in the production of food and fiber, but also in the maintenance of local, regional and worldwide environmental quality [51]. Thus, successful agriculture requires the sustainable use of soil resource, because soils can easily lose their quality and quantity within a short period of time and consequently impose negative impacts on environmental quality.
Despite the tremendous land use changes from forest to grazing and cultivated lands in Ethiopia, particularly during the last five to six decades, the impacts of these changes on soil properties are not well studied and documented. It is also stated that information on the effect of land use and the associated management practices on soil physicochemical properties in the country is generally very little [24]. In the Ethiopian highlands, population pressure, which accounts for 85% of the countrys total population as well as 67% of its livestock population, has pushed
cultivation and livestock grazing to steep slopes and fragile lands causing serious overgrazing and soil erosion. However, the efforts to maintain the fertility of the soil and locally available data of soil fertility status are insufficient and also not quantified. As a cnsequence of continuous cultivation and intensive grazing of land without proper management in the study area resulted in declining soil physical, chemical and biological properties which aggravate crop yield reduction and food shortage. Due to population pressure and shortage of improved technology regarding land use management, inappropriate cultivation and free grazing were being carried out for many years in the study area, which in turn led to declining soil productivity and shortage of fodder. In order to put the proper recommendations for sustainable utilizations of soil resources and improve crop productivity, the information about effects of land use types on soil physical and chemical properties are essential. Therefore, the objective of this study was to investigate the effects of land use types on selected soil physical and chemical properties at Sire morose subwatershed, hidebu Abote district.
-
MATERIALS AND METHODS
-
Description of the study area
The study was conducted at Sire morose sub watershed, located in Hidebu Abote district of North Showa Zone, Oromia Regional State. The sub watershed is located at about 43 km north of zone town, Fitche and 142 km northwest of Addis Ababa. The geographical location of the watershed is in 9.810 47' to 10.08o11'N and 38o27' to 38.67043E with an average altitude of 2149 meter above sea level (masl).
Figure 1. Location of the study area
The main rainy season in the area is from mid-June to mid- September and the short rainy season (Belg) is from March to May. The average annual rainfall and mean annual
minimum and maximum temperatures of the area based on the last 10 years records are 971 mm, and 9.7oC and 21.oC, respectively.
mean rainfall Maximum Temprature Minmum Temprature
max.and min temprature (0c)
max.and min temprature (0c)
400 25
350
20
mean rainfall (mm)
mean rainfall (mm)
300
250 15
200
150 10
100
5
50
0 0
Figure 2. Mean monthly rainfall and mean maximum and minimum temperatures of the study area (Gundo-meskel Meteorology Station)
-
Soil , vegetation and farming system
The dominant soil of the study area is Nitisols and Vertisols [22]. The vegetation type of the district is characterized by tree, bushes and shrubs. The most dominant tree species found in the area Croton macrostachyus, Cordia africana, Olea europaea, Vernonia amygdalina and Erythrina abyssinica.
The agricultural activities in the district are mainly characterized by the presence of subsistence mixed farming system of both crop and livestock production. There are also households engaging in off-farm activities. On-farm trees are the main source of fuel wood demand for the whole communities. The major cereal crops are being produced in the district are tef, wheat, sorghum, and barley and vegetables (onion, potato, redroot, tomato and cabbage). Based on climatic classification, the farming systems vary in such a way that in low land areas, the farmers practice crop production and as in the midland and highland areas both crop and livestock productions are the common practice. Generally, the main sources of income for the local communities are crops and livestock.
-
Site selection and soil sampling
Assumption was made in this research approach that soil conditions and parameters under the three land uses were similar before changes in the land use have been introduced. Differences due to lateral movement of soil material in the watershed slopes before land use changes were assumed to be very small. The observed differences in present soil conditions and other parameters are assumed as being caused by the present land use types and management practices.
Primarily, a general visual field survey of the area was carried out to have a general view of the land use/land cover and management practice in the study area. Then three land uses were selected based on the similarity of topography, aspects and soil and replicated three times. Following this, three land uses (cultivated, forest and grazing lands) were selected and Global Positioning System (GPS) and clinometers were used to identify the geographical locations and slopes of the sampling points, respectively. Composite soil samples were collected from the three land use types (cultivated,grazing and forest ) by two soil depths (0-20 cm and 20-40 cm) with three replications. The whole factors were situated on the same slope and topography. Both undisturbed and disturbed soil samples were taken from two soil depths from towelve sampling point based on the heterogeneity of land unit in a zigzag manner. Undisturbed soil samples were taken by core sampler to measure the soil bulk density, whereas the disturbed soil samples were taken by using an auger to measure the rest selected soil physical and chemical properties.
During the collection of soil samples, gravel materials, dead plants, old manures, areas near trees and compost pits were excluded. This is to minimize the differences variation, which may arise because of the dilution of soil OM due to mixing through cultivation and other factors. After these materials and areas were separated, 18 composite soil samples were collected from representative land use types. Then after, about one kilogram of the soil samples from 18 composite soil samples were sub-sampled and packed by plastic bags with tightly and labeled carefully with the location, representative field and depth of soil and transport to Laboratory. The soil samples
collected from the study area were air dried, ground and passed through a 0.5 sieve for total nitrogen (TN) and soil organic carbon (OC) and 2 mm sieve for the analysis of other soil physicochemical properties. Analyses were done
-
Analysis of soil physical and chemical properties
at Fitche Soil Research Center & Haramaya Universitys Soil Laboratory following general standard laboratory soil analysis procedures for the selected soil physicochemical properties.
Selected soil Physical and chemical properties
Methods of analysis
Texture
Bouyoucos hydrometer by using sodium hexametaphosphateas described by [13]
Bulk density
Core sampling method [43]
pH
pH- meter in the supernatant suspension of 1:2.5 soil to water ratio [26]
Organic carbon (OC)
by the wet oxidation method as described by [52]
Total Nitrogen(TN)
Measured titrimetrically following the Kjeldhal method as described by [26]
Available phosphorous (av.P)
Olsen extraction method [38]
Cation exchange capacity (CEC)
Distillation and titration [11]
The exchangeable basic cations (Ca, Mg, K and Na)
Atomic absorption spectrophotometer (AAS) and flame photometer (FP) respectively [11]
Percent base saturation (PBS)
Computed as the ratio of the sum of exchangeable bases to the CEC multiplied by 100.
Total porosity (%TP)
1 BD) *100
Organic matter ( %OM)
%OC *1.724
-
-
STATISTICAL ANALYSIS
Descriptive statistics was used to reveal the relationships between the three land use types. simple linear correlation analysis was used to detect the relationships between different soil physicochemical properties by using GenStat software.
-
RESULTS AND DISCUSSION
-
Selected soil physical properties under different land use types
-
Texture
The particle size distribution analysis indicated the existence of difference in the proportion of the three separates and their distribution with soil dpth under the three land use types (Table 1). Results revealed that the textural class of soil under all the land use types was sandy clay loam (Table 1). This suggests land use types have no much impact on soil textural class and also, indicats the similarity in parent material of the soil. Considering the three land use types, highest mean sand , silt and clay contents were recorded for surface soil under grazing, forest and cultivated lands respectively (Table 1), whereas the lowest mean silt and clay contents were recorded for subsurface soil under grazing land, while the lowest mean sand (47.67%) content was for the cultivated land. As indicated in (Table 1), the clay percentage increased while the sand and silt decreased from the surface to the subsurface in all land uses. [18] also reported highest clay content in subsurface soil than in the surface soil and
associated with clay migration and leaching processes from the surface soil. The highest sand content at the surface soil of the three land uses might be due to the removal of finer particles by selective erosion or translocation of the finer particle into the subsurface soil. This results are in agreement with the result of [28]. The highest sand content was recorded for soil of the grazing land and the lowest for soil of the forestland followed by the cultivated land (Table 1). This results are in agreement with the result of [4]. Relatively the highest silt content was recorded in soil of forestland and the lowest was for cultivated land followed by grazing land (Table 1). The clay contents in both depths of the cultivated land were higher than its contents in soil under grazing and forest land. This could be due to the intensive and continues cultivation, which might have caused transformation of silt particles into clay size. Similarly, the clay content of subsurface soil of the cultivated land is higher than its content in surface soil. This also shows the enhancement of clay translocation from surface soil due to intensive and continuous cultivation. In agreement with this, [49] suggests the reason for low clay in surface soil of cultivated lands as it might be due to selective removal of clay from the surface by erosion. [2] also reported that soils of different land use systems, but of same area with same soil type and textural class, differed in some other soil physical conditions mainly due to the fact that soil physical properties change with the change in land use systems and its management practices.
LU types
Soil depth
particle size distribution (%)
STC
BD
TP
( cm)
Sand Silt Clay
g/cm3
%
LU types
Soil depth
particle size distribution (%)
STC
BD
TP
( cm)
Sand Silt Clay
g/cm3
%
Table 1. Characteristics of the selected physical properties of soil under different land use types at Sire Morose sub watershed
Forest land
0-20
58.67
15.67
25.66
SCL
0.94
64.53
20-40
54.2
15.47
30.33
SCL
1.11
57.99
Grazing land
0-20
70
10
20
SCL
1.2
54.72
20-40
66.67
8.33
25
SCL
1.4
47.04
Cultivated land
0-20
47.67
18
34.33
SCL
1.25
52.95
20-40
46.34
14
39.66
SCL
1.29
51.45
LU = Land use, STC = Soil textural class, SCL = Sandy clay loam, BD = Bulk density, TP = Total porosity
-
Bulk density
Similar to particle size distribution, soil bulk density also showed some degree of variation with land use types and soil depth in a given land use (Table 1). Under all the three land uses, it showed increasing trend with soil depth. Across land use types, the relatively high bulk density was recorded for surface soil of cultivated land (1.25g/cm3) and the lowest under forest land (0.94 g/cm3).The relatively higher bulk density value recorded for surface soil of cultivated land might be associated with the effect of SOM content as the bulk density values are negatively correlated with SOM (Table 4). The higher bulk density of soil in cultivated land might be due to the practice of ploughing in cultivated soil, which tends to lower the quantity of OM of that soil through animal trafficking and expose the soil surface to direct strike by rain drops. This finding is in agreement with [54] who found the highest bulk density under cultivated land compared to the adjacent grazing and forest lands at a soil depth of 0-20 cm.. Furthermore, the continuous exposure of the soil surface to the direct impact of raindrops under fields with long period of continuous cultivation might have also contributed to the increment of bulk density as raindrop impacts cause soil compaction through disintegration of the soil structure [8]. Relatively bulk density values of soil of grazing land were lower than that of cultivated land soil (Table 1). This might be due to difference in SOM contents among different of land use types. The lowest bulk density value recorded for forestland soil might be due to the highest OM content of soil under this land use, as a result it holds high proportion of pore space to solids, which might have lowered the bulk density. Moreover, in uncultivated land, there was relatively higher organic matter (OM) making the soil loose, porous and well-aggregated that might have reduced the bulk density. The bulk density was negatively correlated with OM and increased with soil depth for all land use systems. Similar results were reported by [10].
Therefore, the bulk density values of soil under three land use types were found within this range; i.e. 0.94 to 1.4g cm-
3. Bulk density is one of the major physical parameters used to evaluate the physical fertility status of soil. According to the range of [7], bulk density values of the soil in the study area were not too compact to limit root penetration and restrict movement of water and air. This indicates the existence of loose soil conditions in all land use types and, hence, good structure.
-
Total porosity
-
Total porosity followed an opposite trend with that of bulk density consistently decreased with an increase in soil depth (Table 1). Similar to bulk density, the changes in land use and also depth have affected porosity differently. Accordingly, in the surface soil the highest (64.53%) mean value of total porosity was recorded for soil of the forest land, while the lowest (52.95% ) was recorded for soil of the cultivated land (Table 1). Also in the subsurface soil the highest total porosity was recorded for soil of forest land followed by cultivated and grazing lands. Within a soil depth, the mean total porosity of the surface soil was greater than that of the subsurface soil (Table 1). The total
porosity increased from the cultivated land followed by the grazing land and forestland. This trend could be attributed to the low OM content of the cultivated land and the increase in bulk density with soil depth, respectively. [51] indicates lower total porosity of cultivated soil at surface and subsoil depths as a result of low OM in the Dystric Nitosols of Bako area. Hence, decreased total porosity of the cultivated land might not allow for easy movement of air and water through soils. This in turn, might reduce plant growth and development. In general, this study clearly showed that total porosity of soil of the study area has been affected by the land use types.
-
Selected soil chemical properties under different land use types
-
Soil reaction (pH)
The pH (H2O) values of the soil were slightly affected by all land use types (Table 2). Under all the three land uses, it showed increasing trend with soil depth. Based on the mean values of the surface soil of land uses, the highest mean soil pH (H2O) ( 6.19) was recorded for soil of forest land followed by grazing land ( 6.15) and the lowest ( 5.9) was recorded for soil of the cultivated land (Table 2). The relatively higher soil pH (H2O) recorded for forestland followed by grazing land could be partly due to the presence of higher total exchangeable bases and percent base saturation (Table 3) than that of the cultivated land. And also the highest mean value of subsurface soil pH(H2O) was recorded for soil of forest land followed by grazing and cultivated lands. This is supported accordingly, basic cations, CEC and pH have positive correlations with each other ( Table 4). The lowest pH value of soil of cultivated land might be due to two major reasons, removal of basic cations with crop harvest, runoff generated from accelerated erosions and leaching. Microbial oxidation that produces organic acids, which provide H ions to the soil solution and there by may lowers soil pH. Moreover, the land use practices such as cultivation and continuous use of inorganic fertilizers might have contributed to the decline in soil pH at the surface soil of the cultivated land. In line with this [24] reported that the use of acidifying mineral fertilizers and intensive cultivation enhanced leaching of basic cat ions and oxidation of organic matter that eventually reduced soil pH. In general, the pH values observed in the study area are within the ranges of moderately acidic to slightly acidic as indicated by [17].
-
Organic matter
Organic matter, being one of the dynamic soil properties revealed variation among land uses and in its distribution with soil depth in a given land use type. Accordingly in soil of all the three major land use types, OM consistently decreased with increase in soil depth (Table 2). Furthermore, across land uses it followed the order : forest land > grazing land > cultivated land , which clearly indicates the deleterious effect of cultivation and intensive grazing on this attribute of soil. The surface soil OM content was the highest in soil of forest land (5.2% ) followed by in soil of grazing land (4.33%) and cultivated land (2.74%) (Table 2). The same trends was observed also for the subsurface soil of the respective land uses, the
highest was in soil of forest land followed by grazing and cultivated lands. [14] reported that SOM content of soil under grazing and cultivation land uses were lower than those under natural forest. The mean values of soil organic matter (SOM) content among land use types, were lower in cultivated land use types as compared to that of forest and grazing land (Table 2). The lowest OM in soil of cultivated land could be due to low organic matter in put coupled by reduced physical protection for SOM as a result of tillage and increased oxidation of soil organic matter. Soil of forest sites were well protected with little disturbance but these of the grazing land were poorly managed; heavily overgrazed and mostly susceptible to surface erosion. In line with this, previous finding also reported decline in soil organic matter due to deforestation and subsequent cultivation [24]. Most of cultivated soils of Ethiopia are poor in organic matter content due to low amount of organic matter applied to the soil and complete removal of biomass from the field [46], severe deforestation and intensive cultivation [29], which aggravates oxidation of organic carbon. According to the rating of soil organic matter as per the ranges suggested by [47] the soils of the study area are medium (2.59 – 5.17%) in soil of cultivated and grazing lands and high ( > 5.17) in that forest land.
-
Total nitrogen
Total nitrogen followed the trend of organic matter and decreased consistently with soil depth in all the three land uses (Table 2). The highest mean value of the total nitrogen was recorded for surface soil of the forest land (0.25%) followed by that of grazing land ( 0.18%), while the lowest value was recorded for the subsurface soil of the cultivated land (0.09%) (Table 2). In subsurface soil the highest
mean value of total nitrogen was recorded in soil of grazing land followed by forest and cultivated land (Table 2). The variation paralleled with that of the change in organic matter content, which was also expressed by the positive correlation ( Table 4), indicating that higher nitrogen in forest and grazing land was due to high organic matter content in respective lands. [9] reported that the distribution of soil nitrogen paralleled that of soil OM due to the fact that nitrogen along with other nutrients, are present in organic combination and are slowly released by the process of mineralization. This was also supported by
[33] who reported that about 90% of TN in soil derives from organic sources.The relatively low TN content recorded in the soils of the cultivated land could be attributed to rapid mineralization of soil organic matter following frequent tillage operations, which increase aeration and microbial accessibility to organic matter. Furthermore, reduced input of plant residues in such cereal-based farming systems into the soils is also expected to have contributed to the depletion of OM and there by also of TN in these cultivated soil. On the other hand, nitrate ions which are not absorbed by the negatively charged colloids that dominate most soils, may move downward with drainage water and be leached from the soil. The findings of this study are in consent with the findings of similar works reported by [50] that the low input of plant residues results in low TN.
Following the rating suggested by [31] total nitrogen content of the surface soil of the study area was low (0.1- 0.2%) in soil of the cultivated and grazing lands and medium (0.2 – 0.5%) for forestland.
Table 2. Chemical properties of soil as affected by land use types at Sire Morose Sub watershed
LU types
Soildepth (cm)
pH (H2O)
SOM (%)
TN (%)
C:N ratio
Av.P (mg/kg)
Forest land
0 – 20
6.19
5.2
0.25
12.08
19.67
20 – 40
6.33
2.62
0.13
11.69
11.67
Grazing land
0 – 20
6.15
4.33
0.18
13.94
17.67
20 – 40
6.25
2.43
0.14
10.07
9.13
Cultivated land
0 – 20
5.9
2.74
0.16
9.94
24.8
20 – 40
6.1
1.03
0.09
6.67
14.47
LU = Land use, SOM = Soil organic matter, TN = Total nitrogen, C:N ratio = Carbon to nitrogen ratio, Av.P = Available phosphorous
-
Carbon to nitrogen ratio
Carbon to nitrogen ratio(C:N) of the soil showed slight variations for land use types and soil depth in the study area (Table 2).The overall C:N ratio was higher in surface soil than in the subsurface. The mean values showed variation of C:N rations of soil of the cultivated land from that of forest and grazing land use types (Table 2). Lower C:N ratio of soil of cultivated land compared to grazing and forest land uses could be attributed to lower level of OM content. In line with this, correlation analysis show positive correlation of C:N with OM ( Table 4). Relative to forest land soils, the recorded narrow C:N ratio for soil of the cultivated land could be probably due to aeration during tillage and increased temperature that enhance higher microbial activity and more CO2 evolution and its loss to the atmosphere from the top (0-20 cm) soil. The overall C:N ratios of soil of the study area were within the range of
8:115:1,which is commonly cited as the general C:N ratio of mineral soil [39].
-
Available phosphorus
Similar to the other soil attributes, available phosphorus also varied among the three land use types and with soil depth in every land use type. Ingeneral, relatively higher available phosphorus was recorded for soil of cultivated land followed by forest and grazing land (Table 2). Furthermore, it decreased with soil depth under all the three land use types Accordingly, in the subsurface soil the highest mean value of phosphorus was recorded for soil of culitivated land followed by forest and grazing lands (Table 2). The highest available phosphorus content recorded for the surface soil of the cultivated land could be ascribed to residual effects from the continuous application of phosphorus fertilizers. In support of this study, [45] reported higher available phosphorus for the surface soils
than in the subsurface soils of cultivated land and attributed this to the addition of fertilizers and manures or easily mineralized organic phosphorus compounds. On the other hand, [53] reported that the low concentrations of available phosphorus in the uncultivated lands could be due to the inherent phosphorus deficiency of the soils since little or no phosphorus fertilizers are applied. According to [5], the inorganic sources of phosphorus from mineral weathering have considerable importance in the contribution of soil available phosphorus as intensified human practices increase the rate of weathering and encourage the decomposition.
Furthermore, the results obtained from this study indicate that the contribution of organic matter to available phosphorus in soil of grazing and forestlands was not conspicuous. This was revealed by the negative correlation between OM and available phosphorus (Table 4). Following the rating suggested by [12], the available phosphorus contents of the study area was medium (10 17 mg/kg) in soil of the grazing land and high (18 25 mg/kg) in the soil of forest and cultivated land use types. This showed that available phosphorus content ranges from medium fertility status in the grazing land to high in the forest and cultivated lands.
-
Exchangeable basic cations
The Exchangeable bases showed difference among land uses and between soil depth in the land use types (Table 3). Except exchangeable K+ in the soil of forest land, all the others followed a consistent increase with soil depth in the three land use types (Table 3). Exchangeable calcium (Ca) and magnesium (Mg) contents of soils of the study area showed differences in response to variations in land uses and soil depths. On the basis of the mean value of land uses by soil depths the highest exchangeable Ca (14.07cmol(+)kg1) was recorded for surface soil of the forest land, whereas the lowest exchangeable Ca (8.49 and
10.56 cmol(+)kg1 was recorded for surface and subsurface soil of the cultivated land respectively (Table 3). In the subsurface soil the highest exchangble Ca was recorded for soil of forest land and followed by grazing and cultivated lands (Table 3). The highest exchangeable Ca observed in the surface soil of the forest land might be due to the higher OM content of this soil. On the other hand, the lowest exchangeable Ca recorded for soil of the cultivated land could be due to its continuous removal through crop harvest with no or little organic matter input into the soil. The present studies are in line with [46] who reported highest and lowest exchangeable Ca in forest and cultivated lands, respectively at Ababo area in western Ethiopia.
On the other hand, based on the mean values, the highest exchangeable Mg (6.4cmol(+) kg1) was recorded for surface soil of forest land and the lowest exchangeable Mg (5.33 and (5.01cmol(+) kg1) was recorded for surface soil of grazing and cultivated lands respectively (Table 3). The relatively low exchangeable Mg observed in the soils of the cultivated land might be due to its continuous removal with crop harvest. [24] reported that continuous cultivation enhances the depletion of Ca2+ and Mg2+, especially in acidic tropical soils. The contents of both exchangeable Ca
and Mg increased with soil depths for all the three land use types (Table 3). This might be due to down ward leaching of basic cations. [3] reported that downward translocation of soluble bases from the surface soil and subsequent accumulation in the deeper layers.
According to the ratings of exchangeable Ca and Mg [16], the observed mean exchangeable Ca was of medium fertility status in the soil of the cultivated land and high in the soils of the forest and grazing land. On the other hand, the mean exchangeable Mg recorded was high fertility status in the soils of all the three land use types.
Exchangeable potassium (K) and sodium (Na) contents varied in response to variation in land uses and soil depths. Based on the mean values, the highest mean of exchangeable K was recorded for soil of the forest land and the lowest (1.01cmol(+)kg1) was recorded for soil of the cultivated land (Table 3). Along soil depth, exchangeable K content decreases in the forest land, while it increased in the grazing and cultivated land. [3] also reported similar results for soil of forest land. The highest exchangeable K content in the soil of the forest land than that of the cultivated land might be attributed to the high organic matter content. As reported by [44], high intensity of weathering, intensive cultivation, and use of acid forming inorganic fertilizers affect the distribution of K in soils and enhance its depletion. This might be the possible reason for the relatively lower exchangeable K in soil of the cultivated land. According to rating suggested by [16], the exchangeable K content of soil of the study area was high (0.6 – 1.2(cmol(+)kg-1) in the soil of cultivated land and very high ( >1.2 cmol(+)kg-1) in the soil of grazing and forest land use types. These results confirm the common argument that Ethiopian soils are rich in K. However [51] reported K deficiency in Eutric Vertisols of Melbe (Tigray) and Dystric Nitisols of Bako area, respectively. Higher content of exchangeable K in the soil suggests the existence of potassium-bearing minerals such as feldspars and micas in the studied area. Therefore, this suggests that potassium fertilizer application my not be required in the area where the soil samples were collected and conducive for optimum crop production. Considering the mean values, the highest exchangeable Na content (0.35cmol(+)kg-1) was recorded for soil of grazing land and the lowest (0.24cmol(+)kg-1) for that of the cultivated land (Table 3). According to rating suggested by [16] exchangeable Na was medium (0.3 – 0.7 cmol(+)kg-1) in soil of the three land use types of the study area.
In general variations in the distribution of exchangeable bases depend on the mineral present, particle size distribution, degree of weathering, soil management practices, climatic conditions, degree of soil development, the intensity of cultivation and the parent material from which the soil is formed [21]. Generally, the exchangeable base contents were well maintained in the forest ecosystem due to nutrient recycling when compared to grazing and cultivated lands, where basic nutrients loss upon grazing and harvesting prevailed. Results revaled that the exchange complex was dominated by Ca followed by Mg, K and Na, considering exchangble bases, soil of the study area . Cation Exchange Capacity
The cation exchange capacity ( CEC) values of the soil of the study area were influenced by land use types and soil depth (Table 3). Accordingly, the highest mean CEC value (35.27 cmol(+) kg1) was recorded for soil of the forest land and the lowest (24.67cmol(+) kg1) was for soil of the cultivated land. Along the soil depths, the CEC of the surface soil was higher than the CEC of the subsurface soil of the respective land uses (Table 3). In soils of all the three land uses, CEC was highest in the forestland followed by the grazing land to the cultivated land and from the surface to subsurface soil in accordance with OM contents (Tables 2, 3). The decreases in the CEC values of soil of this study area with depth might be due to the positive correlation between organic matter and CEC ( Table 4), as it is also evident from the fact that the higher CEC was recorded for the surface soil which also contained the highest organic matter (Tables 2, 3). The depletion OM because of continuous cultivation might have reduced the CEC of the soil of the cultivated land of the study area. In line with this [19] reported that continuous cultivation decreases soil OM and resulted in CEC reduction in the
cultivated land than that of uncultivated land. Moreover
[36] reported that soil CEC recorded values in cultivated land uses decreased mainly due to the reduction in organic matter content. Basically, CEC of a soil depends on the relative amounts and type of colloidal substances (organic matter and clay) as both provide negatively charged surfaces that play important role in exchange process. Particularly, organic matter plays an important role in exchange process, because it provides more negatively charged surfaces than clay particles do [19]. As a result, the CEC records of soils of the study area were positively correlated with OM than with clay, which was negatively correlated to CEC recorded both across the land uses and within the soil depths (Table 4)According to the rating of cation exchange capacity by [23], surface soil of the forest and the grazing lands qualify for high and that of the cultivated land for moderate status of CEC, respectively. Therefore, deforestation, overgrazing and changing of land from forest to crop land without proper management aggravates relatively soil fertility reduction.
Table 3. Exchangeable bases, CEC and PBS under different land use types in the study area
LU types
Soil depth
Exchangeable bases(cmol(+)kg-1)
CEC
PBS
(cm)
Ca
Mg
K
Na
(cmol(+)kg-1
)
(%)
Forest land
0-20
14.07
6.4
1.62
0.32
35.27
63.64
20-40
16.03
6.42
1.58
0.35
29.73
81.92
Grazing land
0-20
10.7
5.33
1.23
0.35
28.87
60.99
20-40
13.38
6.02
1.55
0.37
24.47
87.28
Cultivated land
0-20
8.49
5.01
1.01
0.24
24.67
59.84
20-40
10.56
5.83
1.29
0.31
22.67
79.63
LU = Land use, CEC = Cation exchange capacity, PBS = Percent base saturation
cations than the cultivated land of Vertisols at the central
-
Percent base saturation
-
Percentage base saturation (PBS) was influenced by land use and soil depth (Table 3), considering the mean values of surface soil of the land uses, the highest (63.64%) and the lowest (59.84%) PBS were recorded for soil under forest and the cultivation, respectively (Table 3). Fore subsurface soil the highest mean values was recorded for soil of grazing land followed by forest and cultivated land (Table 3). [1] indicates that virgin lands retain more basic
highlands of Ethiopia. On the other hand, among the two soil depths, higher PBS was observed in the subsoil than in the surface soil. In general, processes that affect the extent of basic cations also affect percent base saturation. According to the ratings of base saturation by [23],
The observed PBS of soil of the study area falls in the range of medium fertility status for cultivated and high for grazing and forestland use types.
Table 4. Linear correlation between selected physicochemical properties of soil of the study Area
Sand
Clay
Silt
BD
pH
OM
N
C/N
av.P
Ca
Mg
K
Na
CEC
sand
1
clay
-0.96
1
Silt
-0.85
0.67
1
BD
-0.07
0.35
-0.46
1
PH
0.75
-0.90
-0.29
-0.72
1
OM
0.58
-0.78
-0.06
-0.86
0.97
1
N
0.14
-0.41
0.40
-1.00
0.76
0.89
1
C/N
0.97
-1.00
-0.69
-0.32
0.89
0.76
0.38
1
av.p
-0.95
1.00
0.64
0.39
-0.92
-0.81
-0.45
-1.0
1
Ca
0.32
-0.57
0.22
-0.97
0.87
0.96
0.98
0.55
-0.61
1
Mg
0.15
-0.41
0.40
-1.00
0.77
0.89
1.00
0.39
-0.45
0.98
1
k
0.28
-0.54
0.26
-0.98
0.85
0.95
0.99
0.51
-0.57
1.00
0.99
1
Na
0.95
-1.00
-0.63
-0.4
0.92
0.81
0.45
1.00
-1.00
0.61
0.46
0.58
1
CEC
0.32
-0.57
0.22
-0.97
0.87
0.96
0.98
0.55
-0.61
1
0.98
1.00
0.61
1
-
-
CONCLUSION
In general, the information generated from the present study on the effect of land use types on selected soil physicochemical properties might be helpful for proper management of land in the area. This study showed that conversion of natural ecosystem into crop/cultivated land ecosystem has resulted in deterioration of the soil physicochemical properties. Most of the physicochemical properties of the study area were considerably influenced by the different land uses. Therefore, further detailed studies that include plant response, soil characterization, and field experiment on crop nutrient requirement should be conducted to examine effect of land use types on soil physic-chemical properties on sustainable use of the land.
ACKNOWLEDGEMENTS
I would like to thank Oromia Agriculture Research institutes provide funds for this study . In addition, I would like to acknowledge fitche Agricultural research center for providing laboratory service.
REFERENCE
[1] Abebe ,M . (1998) Nature and management of Ethiopian soils.Alemaya University,Alemaya, p 272
[2]. Achalu, C., Heluf, G., Kibebew, K. and Abi , T. (2012). Status of selected physicochemical properties of soils under different land use systems of Western Oromia, Ethiopia. Journal of Biodiversity and Environmental Sciences 2(3): 57-71 [3]. Berhanu, H. 2015. Evaluation of selected soil physicochemical properties across land use types and soil depth in sum be sub- watershed of west Showa Zone, central Ethiopia. MSC. Thesis Haramaya University: 31-34. [4]. Berhanu, S. (2016). Assessment of soil fertility status of vertisoil under selected thee land uses in Girar jarso district of north Shoa Zone Oromia National Regional State, Ethiopia,P. 5-6 [5]. Bewket W, Steroosnijder, L. (2003). Effects of agro-ecological land succession on soil properties in the Chemoga watershed, Blue Nile Basin, Ethiopia. Geoderma 111:8598 [6]. Bewket, W. and Teferi, E. (2009). Assessment of soil erosion hazard and prioritization for treatment at the watershed level: case study in the Chemoga watershed, Blue Nile basin, Ethiopia, Land Degrad. Dev., 20: 609622. [7]. Bohn, H.L., McNeal, B.L. and O'Connor, G.A. (2001). Soil Chemistry. 3rd edition. John Willey and Sons, Inc., New York. [8]. Brady N.C., and Weil, RR . (2002). The nature and properties of soils,13th edn. Prentice Hall International Inc, New Jersey: [9] Brady, N.C.;Weil, R.R. (2005). The Nature and Properties of Soil, 12th ed.; Prentice Hall: Upper Saddle River, NJ, USA, [10]. Çelik, I. (2005). Land use effects on organic matter and physical properties of soil in a southern Mediterranean highland of Turkey. Soil and Tillage Res., 83:270-277. [11]. Chapman, H.D. (1965). Cation-exchange capacity. Methods of Soil Analysis. Part 2. Chemical and microbiological properties, (methodsofsoilanb): 891-901 [12]. Cottenie, A. (1980). Soil and plant testing as a basis of fertilizer recommendations FAO soil Bulletin 38/2. Food and Agriculture Organization of the United Nations, Rome [13]. Day, P.R. (1965). Particle fractionation and particle-size analysis. Methods of soil analysis. Part 1. Physical and mineralogical properties, including statistics of measurement and sampling, (methods of soilana): 545-567. [14]. Dawit, S., F Fritzsche, M., Tekalign, J.,Lehmann, W Zech. (2002). Soil organic matter composition in the sub-humid Ethiopian highlands as influenced by deforestation and agricultural management. Soil .Sci. Soc. Am. J 66: 68-82 [15]. FAO (Food and Agriculture Organization of the Unted Nation). (2005). Global Forest Resources Assessment Progress towards Sustainable Forest Management. Forestry Paper 147, Rome. [16]. FAO (Food and Agriculture Organization of the Unted Nation). (2006). Plant nutrition for food security: A guide for integratednutrient management. FAO, Fertilizer and Plant Nutrition Bulletin 16, Rome.
[17]. Foth, H.D. and Ellis, B.G. (1997). Soil Fertility. 2nd Edition. Lewis Publication, Boca Raton, Florida. [18]. Fungo, B.S., Gundwald, M.M., Tenywa, B., and Nkedi-Kizza, P. (2011). Lunnyu soil in the lake Victoria basin of Uganda : Link to top sequence and soil type. African journal of Environmental Science Technology, 5(1): 15-24. [19]. Gao G, Change C. (1996). Changes in Cation exchange capacity and particle size distribution of soil as associated with long term annual application of cattle feed lot manure. Soil Sci 161:115120 [20]. Gete, Z. (2002). Resource use and poverty in the Ethiopian high lands, 51-61. In T. Amede (Ed.), Proceedings of the conference on: Natural resource degradation and environmental concerns in the Amhara: Regional State, Ethiopia: Impact On Food Security, March 2003. Bahir Dar, Ethiopia. [21]. Gol . (2009). Effects of Land Use Change on Soil Properties and Organic Carbon at Dagdami River Catchment in Turkey. Journal of Environmental Biology, 30 : 825-830. [22]. HAANRO (Hidebu Abote Agricultural and Natural Resource Office). 2016. Report about natural resource of Hidebu Abote to North Shoa Zone Agricultural and Natural Resource Office, Fitche, Ethiopia. [23]. Hazelton P, Murphy B. (2007). Interpreting Soil Test Results: What do all the numbers mean? 2ndEdition. CSIRO Publishing. [24]. Heluf, G. and Wakene, N. (2006). Impact of land use and management practices on chemical properties of some soils of Bako area, Western Ethiopia. Ethiopian Journal of Natural Resources, 8(2) : 177-197. [25]. Islam, K.R. and Weil, R.R. (2000). Land Use Effects on Soil Quality in a Tropical Forest Ecosystem of Bangladesh. Agriculture, Ecosystems and Environment, 79: 9-16. [26]. Jackson, M.L. (1973). Soil chemical analysis Printice Hall. Ind, Englewood, Chiffs N.J. USA. [27]. Karltun, E., Lemenih, M., and Tolera, M. (2013).Comparing farmers perception of soil fertility change with soil properties and crop performance in Beseku, Ethiopia, Land Degrad. Dev., 24: 228 235, [28]. Khan, S.K . and Chatterjee, A. K. (2001). Effect of continuous rice cropping on change in pedon characteristics in an Ustalf.J Indian Soc. Soil Science, 49:368-370. [29]. Kizilkaya, R., and Dengiz, O. (2010). Variation of land use and land cover effects on some soil physicochemical characteristics and soil enzyme activity. Zemdirbyste-Agriculture, 97(2): 15-24. [30]. Lal, R. (2000). Soil management in the developing countries. Soil Science, 165(1), 57-72. [31]. Landon, J. R. (2014). Booker Tropical Soil Manual: a handbook for survey and agricultural land evaluation in the tropics and subtropics. Routledge, Abingdon,UK.532p. [32]. LVBC (Lake Victoria Basin Commission). (2007). Regional Transboundary Diagnostic Analysis (RTDA) of Lake Victoria Basin. No. 4, Lake Victoria Basin Commission Publication, Kisumu [33]. Matsumoto, S.; Ae, N. (2004). Characteristics of extractable soil organic nitrogen determination using various chemical solutions and its significance for nitrogen uptake by crops. Soil Sci. Plant Nutr., 50: 19. [34]. Mojiri, A., Aziz, H.A. and Ramaji, A. (2012). Potential decline in soil quality attributes as a result of land use change in a hillslope in Lordegan, Western Iran. African Journal of Agricultural Research, 7(4), pp. 577-582. [35]. Murty, D., Kirschbaum, M.F.U., Mcmurtrie, R.E. and Mcgilvray, H. (2002). Does Conversion of Forest to Agricultural Land Change Soil Carbon and Nitrogen? A Review of the Literature. Global Change Biology, 8: 105-123. [36]. Nega,E. and Heluf ,G. (2009). Influence of land use changes and soil depth on cation exchange capacity and contents of exchangeable bases in the soils of Senbat Watershed, western Ethiopia. Ethiopian Journl of Natural Resources, 11(2): 195-206. [37]. Nega, E. and Heluf ,G. (2009). Influence of land use changes and soil depth on cation exchange capacity and contents of exchangeable bases in the soils of Senbat Watershed, western Ethiopia. Ethiopian Journal of Natural Resources, 11(2): 195-206. [38]. Olsen, S.R., Cole, C.V., Watanable, F.S. and Dean, L.A. (1954). Estimation of variable phosphorus in soil by extraction with sodium bicarbonate. USDA Circular. 939: 1-19. [39]. Prasad R, Power JF. (1997). Soil Fertility Management for Sustainable Agriculture. Lewis Publisher, Boca Raton. [40]. Rai, S.C. and Sharma, E.(1998). Comparative Assessment of Runoff Characteristics under Different Land Use Patterns within a Himalayan Watershed. Hydrological Process, 12: 2235-2248. [41]. Richter, D.D., Markewitz, D., Heine, P.R., Jin, V., Raikes, J., Tian,K. and Wells, C.G. (2000). Legacies of Agriculture and Forest Regrowth in the Nitrogen of Old-Field Soils. Forest Ecology and Management, 138: 233-248.
[42]. Ronggui, W. (2001). Effect of land use on soil fertility and phosphorus dynamics in sub-alpine grassland soil of Gansu, China. Doctoral Dissertation, University of Saskatchewan, Canada. [43]. Sahlemedhin, S. and Taye, B. (2000). Procedures for soil and plant analysis. National Soil Research center Techl. Paper, 74. NFIA,Addis Ababa, Ethiopia
[44]. Saikh, H., Varadachari, C., Ghosh, K . (1998). Effects of deforestation and cultivation on soil CEC and contents of exchangeable bases: a case study in Simplipal National Park, India.Plant Soil, 204: 6775
[45]. Samadi, A., and Gilks, R.J. (1998). Forms of phosphorus in virgin and fertilized calcareous soils of Western Australia. Aust J Soil Res., 36:585601 [46]. Sheleme, B. (2011). Characterization of soils along a top sequence in Gununo area, southern Ethiopia. Journal of Science and Development, 1(1): 31-41. [47]. Tekalign, T. (1991). Soil, plant, water, fertilizer, animal manure and compost analysis. Working Document No. 13. International Livestock Research Center for Africa, Addis Ababa [48]. Tesfahunegn, G. B. (2016). Soil quality indicators response to land use and soil management systems in Northern Ethiopias catchment, Land Degrad. Dev.,27: 438448, doi:10.1002/ldr.2245,. [49]. Teshome, Y., Heluf, G., Kibebew, K. and Sheleme, B . (2013). Impacts of Land Use on Selected Physicochemical Properties of Soils of Abobo Area, Western Ethiopia. Agriculture, Forestry and Fisheries, 2(5): 177-183. [50]. Tisdale, S.L., Nelson W.L., Beaton J.D. and Havlin J.L . (2002). Soil Fertility and Fertilizers, 5th edn. Prentice Hall of India, New Delhi [51]. Wakene, N. (2001). Assessment of important physicochemical properties of Dystric Udalf (Dystric Nitosols) under different management systems in Bako area, western Ethiopia. M.Sc. Thesis, Alemaya University,Ethiopia. [52] Walkley, A. and Black, I.A. (1934). An examination of the different method for determining soil organic matter and a proposed modification of the chromic acid titration method. Journal of Soil science, 37(1): 29-38. [53]. Whitebread, A.M., Lofroy, R.B.D., Blair, G.J. (1998). A survey of impact of cropping on soil physical and chemical properties inNortheastern NewSouth Wales. Aust J Soil Res 36:669681 [54]. Yitbarek, T., Gebrekidan, H., Kibret, K., Beyene, S., (2013). Impacts of Land Use on Selected Physicochemical Properties of Soils of Abobo Area,Western Ethiopia. Agriculture, Forestry and Fisheries 2(5): 177-183.