
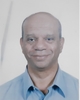
- Open Access
- Authors : Mohamed Z. Ramadan
- Paper ID : IJERTV11IS010118
- Volume & Issue : Volume 11, Issue 01 (January 2022)
- Published (First Online): 19-02-2022
- ISSN (Online) : 2278-0181
- Publisher Name : IJERT
- License:
This work is licensed under a Creative Commons Attribution 4.0 International License
Effects of Pencil Design and Hand Skin Temperature on Tracking Task Performance
Mohamed Z. Ramadan
Production Engineering Department, Faculty of Engineering, Helwan University, Helwan, Egypt
Abstract Small handheld devices have allowed daily life to become extremely convenient. Accordingly, they have become increasingly important, particularly for devices that have been utilized as user interface for character drawing inputthe key topic of this research. Experiments were conducted to determine the effect of pencil shape and size, and hand skin temperature on a tracking task performance. Eighteen high school students and 18 primary school students used a total of nine different pencils of three shapes (i.e., circular, elliptical, and hexagonal) and three sizes (large, medium, and small with 320, 180, and 50 mm² cross-sectional areas, respectively). The task was performed at three hand skin temperatures (i.e., 10, 20, and 30
°C). The tracking performance was measured by having the participants follow a maze. The dependent variables were the tracking movement speed and accuracy, and incremental discomfort rating. Results revealed that the use of small and medium-sized pencils with circular and hexagonal cross- sectional areas were superior to other conditions under the medium environmental temperature in terms of high movement speeds, fewer errors, and lower discomfort ratings. In the case of drawing under cold weather, a small circular pencil is recommended for the use of young students.
Keywords Design; Shank design; Human-performance; Drawing.
I. INTRODUCTION
Despite the widespread use of technology, handwriting continues to significantly influence communication and self- expression. Numerous pen-based products, such as palm pilots, mobile phones, and tablet PCs, have been developed in recent years and have increased screenwriting frequency with touch-pens [1]. On the other hand, Pape and Ryba [2] reported that students spend one-third to one-half of a school day engaged in pencil and paper activities. The similarity between the use of new pens and pencils is on the manner of how the pen is held in a person's hand. Kiss [3] emphasized that a legible handwriting is a necessary skill in a students education. Different problems in handwriting are studied by occupational therapists because it has a significant function in practically all of the educational experiences of students [4]. Chase [5] reported that an educational task written with good penmanship is graded higher than work with poor penmanship. Poor writing performance could result from an application of the wrong grip at the crucial development stage of a child, which could lead to a low self-esteem, lack of self- confidence, and negative influence on the childs life [6, 7]. Moreover, a poor handwriting position can slow down writing as the hand quickly tires and causes stiff wrists, sore fingers, tired hands, and in some cases, even structural problems, such as bad back and improper shoulder postures [8]. Nevertheless, the essential practice of writing has also been hampered by the use imperfect writing instruments [4, 9].
One matter that has been investigated since the 1940s is the question on the effect of the size and shape of the writing instrument on a childs handwriting [10]. Researchers have explored various sizes and shapes of pencils in order to determine whether these have an influence on childrens handwriting [11]. Krzesni [12] and Graham [13] reported that using a pencil with a large diameter aids in properly positioning the finger by developing the childs large arm muscles. Tseng and Cermak [14] emphasized that the most important factor that influences handwriting is the development of a dynamic tripod grip, which implements the thumb, index finger, and middle finger in a coordinated manner so as to manipulate the writing instrument. In a study conducted by Ziviani and Elkins [15], no significant difference was found for either speed or legibility when using different pencil sizes.
Specifically, Wiles [16] used three different pencil diameters (7.4, 8.6, and 9.8 mm) in first-grade children to measure their writing skills. The result of the study showed that the size of the writing pencil had little influence on the handwriting achievement. The same result was obtained by Carlson and Cunningham [17] when they observed 48 preschool children, who used a regular pencil, as well as a primary pencil in their handwriting activities. Oehler et al.
-
conducted a research that examined the effect of the pencil size and shape on the writing achievement of children in kindergarten. The result of their study indicated that neither the size nor the shape of the pencil has an effect on the writing quality of children. Amundson [19] suggested some contributions that parents and teachers provide to help the child determine which writing instrument is the most comfortable and efficient for them. Moreover, Goonetilleke et al. [2] tested subjects performing a writing task in the Chinese and English languages using 36 ballpoint pens. The results indicated that the Chinese subjects preferred a circular-shaped pen for writing in both languages, and that the pen with an equivalent diameter of 8 mm had the best accuracy, even though it had the lowest movement time during drawing. Moreover, the results confirmed the applicability and validity of the Drury tracking model [21] for such a task.
The effect of low temperature on the tracking skill has not been reported. However, there was a study related to tactile sensitivity. This revealed that tactile sensitivity decreased when the temperature is low [22]. Morton and Provins [23] reported that each activity has a moderately sharp critical temperature at which performance considerably deteriorates. This reduction in sensitivity makes the manipulation of small objects difficult. Therefore, assembling small parts and tracking tasks can be negatively affected by cold temperatures if no proper countermeasure is employed.
Scientists have proposed the use of gloves under low temperature work conditions as a hand safety measure. However, the dexterity linked to the manipulation of small parts and assembly operations is reduced with the use of gloves [24]. Thus, this is not necessarily an appropriate solution. Therefore, it is a necessity to identify the lower limitation of the tracking skill without any performance reduction when bare hands are used. Even though Sanders and McCormick [25] reported that the lower limit for unimpaired tracking skill remains undefined, and they proposed an ambient temperature range of 413 °C.
In addition, instead of the emphasis being placed on the so-called 'correct' pencil grip, considerable attention should be focused upon other underlying factors of poor handwriting performance. In an investigation conducted by Goonetilleke and Hoffmann [22], 18 subjects participated in the study on hand skin temperatures associated with bath temperatures of 10, 20, and 30 °C. Tracking performance was measured using the same procedures employed by Drury [21], where lateral constraints were imposed on the movements involved in the task of drawing between two continuous straight lines of length 20 cm and widths 0.15, 0.2, 0.25, 0.3, and 0.4 cm. Performance measures considered the movement time to draw the line and number of errors committed by counting the cross-over points of the drawn line with the printed lines. Both performance measures were consistent and showed the significant effects of line spacing and hand skin temperature. The performance measures under a bath temperature of 10 °C were significatly lower than those under bath temperatures of 20 and 30 °C. In the experiments of Brajkovic and Ducharme [26], significant reductions in finger dexterity were associated with reductions in the finger skin temperature. Giesbrecht et al. [27] concluded in their study that the cooling of the body and/or arm produces significant decrements in the arm, hand, and finger performance. These decrements are ultimately because of the local effect of arm tissue cooling.
A pencil used for drawing and writing is a hand tool that can be considered as an extension of the hand. Several factors, such as the length, diameter, and shape of the pencil affect handwriting performance and efficiency [28]. Therefore, identifying the appropriate shapes and sizes of pencils are crucial design problems. In addition, because school days were always in winter in most countries all over the world, and the decrement in drawing and writing performances could be quite significant, hand skin temperature should be considered in the evaluation. An effectively designed pencil may improve student performance and reduce musculoskeletal injuries related to pencil use in cold weather. Consequently, the question on whether varying the hand skin temperature, and pencil shank shape and size could affect the speed and accuracy of drawing and writing arises.
-
MATERIALS AND METHODS
This study attempted to provide students with the most highly practical and functional drawing instrument to be used in winter. All pencil shapes and sizes available in the local market were documented and reported. The nine pencils shapes and sizes selected were manufactured using wooden material. They were selected because they are the most used pencil for drawing and writing purposes, similar to those used
in the study of Goonetilleke et al. [20], as shown in Fig. 1a. Three temperature-controlled water baths were used to alter the participants hand skin temperatures, as those used in the studies of Goonetilleke and Hoffmann [22], and Ramadan [27]. The results of their investigation are compared with those of the present study, as shown in Fig. 1b. Tracking the shape of a maze was used as the drawing task, as shown in Fig. 1c.
-
Participants
Eighteen male high-school students (mean ± SD: age 16.2
± 0.99 years; mass 69.8 ± 2.9 kg; height 165.1 ± 5.3 cm) as well as 18 male primary school students (mean ± SD: age 10.8
± 1.1 years; mass 36.5 ± 8.6 kg; height 140.9 ± 7.5 cm) were participants in this experiment. Letters explaining the aims of the study along with consent forms and health history forms were sent to the homes of students. In the letter, the parents were requested to return all completed forms to the school if they were willing to allow their children to participate in the study. The main investigator discussed the goals and procedures of the study with the teachers during their meeting sessions. Three hours divided in three separate days were implemented, and data were collected during school weekends. At the end of each data collection session, each student went home with a gift.
All participants were right hand dominant, as established by their preferred usage. None of the participants reported a history of orthopedic injury, upper extremity trauma, deformities, or vascular disease. Each participant's parent signed a consent form approved by the University Human Subjects Internal Review Board. The evaluation included a thorough clinical examination to ensure the absence of hand or arm pathology that might affect hand drawing.
-
Pencil design
A total of nine pencils were modeled in three different cross sections: hexagonal, circular, and elliptical, all resembling common drawing instruments. Each shape was made in three sizes: large (cross-sectional area of 320 mm2 with a mass of 43 g), medium (180 mm2 and 24 g) and small (50 mm2 and 13 g). The equivalent circular diameters are 20, 15, and 8 mm, respectively. These pencil sizes were chosen to cover the range of pencil sizes in common use.
-
Hand skin temperature
Three temperature-controlled water baths maintained at 10, 20, and 30 °C were used to alter the participants skin temperatures. During the entire experiment, two helpers were responsible to provide water continuously from large controlled vessels to keep water temperatures constant as indicated in each bath. The hand skin temperature was not measured in this experiment but previous research has suggested that the hand skin temperature can be assumed to approximate the water bath temperature [30].
-
Maze parameters
A maze design (16 cm × 10 cm) was used in the study, as shown in Fig. 1c. The maze was a cut-out design and consisted of 22 segments, which were connected at their intersections. The maze consisted of vertical, horizontal, inclined, and curved segments arranged from left to right movement directions to test several motion directions in one maze. The total correct path length of the maze design was
77.2 cm. The width of each segment was 0.25 cm. The design
formed a closed loop, i.e., the endpoint of one segment was the starting point of the next segment, and the design could be traced continuously. Participants traced and practiced two forms of the 22-segment maze.
-
Discomfort Ratings
The ratings considered locally perceived discomforts (LPD) on hand. The LPD method consisted of a detailed handwrist map with five regions, as shown in Fig. 1c. Three 6-cm line drawings associated with each reported body part were visually presented to the participant to express his underlying discomfort in terms of felt pain, numbness and pressure, and tiredness. A six-point scale was used to assess discomfort ranging from 0 (= no discomfort) to 5 (= extreme discomfort, the highest) per region [31]. Discomfort ratings are easy to use and virtually require no training [32]. Each participant was asked to indicate and rate any discomfort by marking the prescribed line with an asterisk at the start of each trial and immediately after completing each task. The rate of increase of the discomfort was considered for statistical analysis.
-
Experimental design as data processing
The pencil shape, pencil size, and hand skin temperature were employed in this experiment as independent variables. The time spent to complete the maze, drawing accuracy, and discomfort ratings were measured and computed. The drawing speed was computed by dividing the total movement distance by the time to complete the maze moving from the edge of the start circle entry point to the edge of the end circle passing through the center of the maze. Movement time was measured with a Q & Q stopwatch (Japan). The drawing accuracy was computed by counting the number of points at which the line drawn by the participant crossed the maze borders. The total number of such points was recorded as total out-touches. If a participant was unable to complete the maze in a given 30-s duration, the participant was asked to stop at that point, and each incomplete section of the maze was considered as 10 additional errors. Moreover, the discomfort rating was the participants perception regarding the difficulty of the drawing using the assigned instrument.
The order of the pen presentation to each participant and temperature were completely randomized, but each participant was tested through all of the nine pens and three available temperatures. Participants were instructed not only to draw as fast as possible, but also to maintain high accuracy. Each participant performed two trials with the maze. Accordingly, all of them used each pen to draw on a sheet of paper twice in each hand skin temperature.
A mixed design was conducted with three within factors: pencil shape, pencil size, and hand skin temperature. The student age group was a factor in the between-subject design. This design was implemented to collect data, which were thereafter analyzed using the Statistical Package for the Social Sciences Software (SPSS Version 2; www.spss.com). The main factors identified as significant were further analyzed using Tukey's test to differentiate among the factor levels. Additionally, if the interaction effect was identified, the simple effect technique [33] was conducted to demonstrate the impact of the factor on each level of the other factors. The Shapiro-Wilk [34] test was implemented to test data
normality. The statistical significance was set at a 95% confidence level.
-
Experimental protocol
Each participant underwent 27 treatment sessions. During the first day, screening processes were done by the investigator, and the consent forms of students were collected. The selected students were asked to take their shoes off before they were weighed. Furthermore, anthropometric data of each participant was taken with the participants demographic data before the execution of the experiment. The dimensions of their fingers (thumb, index finger, and middle finger) were measured. Finally, each participants schedule for the test was set.
Drawing sessions were started from the second to the fourth day. Nine sessions were planned for a day to minimize any physical activities that may introduce hand tiredness. At the beginning of each session, the participant was asked to dip his hand in a temperature-controlled water bath for 1 min in a case where the temperature of the previous treatment was approximately 10 °C, and for 2 min in a case where the temperature of the previous treatment was approximately 20
°C. Morton and Provins [23] reported that in their study, the finger temperature of their participants dropped at a rate of approximately 10 °C/min. All sessions started with a 1-min dip. Thereafter, the participant was asked to remove his hand from the water and dry it. Moreover, each participant was asked to express any feeling of hand discomfort before starting the task on the first maze from the group of two mazes using one of the assigned pencils.
The maze drawing procedure was similar to that used by Srinivasan [35]. Participants were asked to directly position their hands in such a way that the pencil point was set in front of the starting point of the maze picture, and to start drawing the line from the beginning point to the end point, as shown in Fig. 1c. They were asked to complete the task with their dominant hand. A 30-s time limit was given and was verbally counted down by the experimenter as the task was being completed by the participant. All the participants were asked not to support their arm on the horizontal surface while drawing the line. Directions pertaining to the task were stated as follows: Now we will complete the maze posted in front of you. You will have 30 s to trace the maze with your pencil. Do not place your arm on the table surface. You must hold the pencil like this (demonstrate proper hand position). Avoid going outside of the maze line. Do your best to finish the maze within 30 s. I will count down your time like this30, 29, 28, 27, and so on. Okay, lets begin. Go ahead and pick up your pencil, and get ready. Ready. Start (counting down) If any participant was unable to complete the maze in the given 30-s period, the participant was asked to stop the line wherever he was in the maze. Nobody among the students failed to complete the maze on time.
When a participant has completed the drawing, the experimenter stopped the timer. Thereafter, the participant was asked to rate his discomfort on regions of his hand in relation to the assigned pencil used, according to previously described rating scale. The drawing speed and accuracy ratings were collated. The participant was asked to dip his hand again in a temperature-controlled water bath for 1 min. Thereafter, he was requested to remove his hand from the
water and dry it. Finally, the participant was asked to complete the second maze with the use of the same assigned pencil. Drawing time, accuracy, and discomfort ratings were also collected. After completing the first treatment, the participant was given a 3-min break before starting with the second treatment. The average ratings from the two treatments on drawing speed, accuracy, and discomfort were computed for statistical analysis.
a
b
a
b
c
Figure 1. a) Pencils used in the experiment, b) participant preparation before executing the experiment, c) a collecting data sheet showing the maze that was employed in the experiment and the employed subjective measurement.
c
Figure 1. a) Pencils used in the experiment, b) participant preparation before executing the experiment, c) a collecting data sheet showing the maze that was employed in the experiment and the employed subjective measurement.
-
Movement Speed
-
-
RESULTS
-
Drawing accuracy
The main independent variables had effects on the drawing
The main effects of all independent variables on the movement speed were significant. Also, the hand skin temperature by pencil shape had significant effects with F(4,136)=24.505, p<0.0001; hand skin temperature by pencil size with F (4, 136) = 6.558, p<0.0001. Moreover, it was found that high-school students were significantly faster in drawing than primary school students by approximately 39.17% at p<0.0001.
Figure 2 Effect of hand skin temperature by pencil shape on movement speeds.
As shown in Fig. 2, students drew in a significantly faster manner at 20 °C hand skin temperature when using a pencil with a circular or hexagonal cross section when compared to drawing with one having an elliptical cross section (p<0.0001 and p<0.007, respectively). Similarly, students drew in a significantly faster manner at 30 °C hand skin temperature, using a pencil with a circular or hexagonal cross section when compared with using a pencil having an elliptical cross section (p<0.0001 and p<0.033, respectively). As shown in Fig. 3, students drew in a significantly faster manner at a hand skin temperature of 30 °C with the use of a small pencil size than drawing with a large pencil size (p<0.007).
Figure 3 Effect of hand skin temperature by pencil size on movement speeds
accuracy and hand skin temperature with F(2,68)=32.623, p<0.0001; pencil shape with F(2,68)=100.512, p<0.0001; pencil size with F(2,68)= 37.504, p<0.036. In addition, the interaction between hand skin temperature and pencil size was significant with F(4, 136)=2.159, p<0.036.
As shown in Fig. 4, the simple effect technique showed that the comparisons among the pencil sizes were significantly different at a hand skin temperature of 10 °C, in which the use of small and medium pencil sizes resulted in less errors than with the use of a large pencil size (p<0.012 and p<0.02, respectively). Furthermore, at a hand skin temperature of 20
°C, there were significantly less errors when small and medium pencil sizes were used when compared with the use of a large one (p<0.001 and p<0.001, respectively). However, at a hand skin temperature of 30 °C, there were significantly more errors when a large pencil size was used compared with the use of medium or small ones (p<0.002, p<0.0001, respectively). In addition, as shown in Fig. 5, there were significantly less errors when circular and hexagonal pencil shapes were used compared with the use of one with an elliptical cross section (p<0.0001 and p<0.0001, respectively).
Figure 4 Effect of hand skin temperature by pencil size on path tracking
errors.
Figure 5: Effect of pencil shape on path tracking errors
-
Incremental discomfort rating
All main variables had significant effects on incremental discomfort: hand skin temperature with F(2, 68)=31.994, p<0.0001; pencil shape with F(2, 68)=124.921, p<0.0001; pencil size with F(2, 68)=33.489, p<0.0001.
As shown in Fig. 6 a, the use of a one-way analysis of variance showed that the participants were significantly more comfortable in drawing at hand ski temperatures of 20 and
30 °C when compared with drawing at a hand skin temperature of 10 °C (p<0.0001 and p<0.0001, respectively). There was no significant difference in the uncomfortable feeling with the use pencils at either hand skin temperatures of 20 or 30 °C.
As shown in Fig. 6 b, results showed that participants significantly felt more discomfort when they used a pencil with an elliptical cross section as compared with those that have circular or hexagonal shapes (p<0.0001 and p<0.0001, respectively). There was no significant difference in using pencils with circular or hexagonal cross sections.
Moreover, as shown in Fig. 6 c, results showed that participants significantly felt more discomfort in the use of a large pencil as compared with the use of medium and small pencils (p<0.0001 and p<0.0001, respectively). Furthermore, results showed that participants significantly felt more discomfort when they used a medium-sized pencil when compared with those with a small size (p<0.0001).
a
b
c
Figure 6. Effects of main variables on incremental discomfort ratings.
-
DISCUSSION
The authors should discuss the results of this study and determine how they can be interpreted in the perspective of previous studies and of working hypotheses. The findings and their implications should be discussed in the broadest context possible. Future research directions may also be highlighted.
The experiment was aimed at investigating the performance of various shapes and sizes of designed and manufactured pencils at different hand skin temperatures in relation to drawing speed, number of errors, and discomfort ratings. The pencils had three different cross-sectional areas (50, 180, and 320 mm2) and three different cross-sectional shapes (circular, elliptical, and hexagonal). Of primary interest is that the movement speed, tracking error performance, and discomfort rating were strongly dependent on hand skin temperatures, with errors as well as incremental discomfort ratings increasing with decreasing hand skin temperature. In addition, the movement speed increased with increasing hand skin temperature. These results agreed results obtained by other researchers, such as Gaydos and Dusek [36], Drury [21], Lockhart et al. [37], and Tikuisis et al. [38]. At a hand skin temperature of 10 °C, all tracking performances deteriorated. Consequently, rating differences among pencil shapes and sizes were not observed. These results are similar with those of the fine finger dexterity and gross finger dexterity reported by Brajkovic et al. [39], where there were no differences between assembly and disassembly tasks performed at 7 °C with bare and gloved hands.
Although the effects of temperature on errors appear to be high (increasing approximately at 10.5% in the number of errors) in the range 2010 °C, the effects of temperature on errors seem to be low (decreasing approximately at 0.5% in the number of errors) in the range 2030 °C. Relative to a temperature of 20 °C, the drawing speed at 10 and 30 °C can be modelled approximately at a decrement of 5 mm/s and an increment of 3 mm/s, respectively. These values are approximately 50% of those reported by Goonetilleke and Hoffmann [20]. A rational explanation is that employed participants in this study were children and adolescent, whereas participants in the study of Goonetilleke and Hoffmann [20] were young adults. Finally, it was found that participants preferred to draw at a hand skin temperature of either 30 or 20 °C rather than at 10 °C.
The effects of the reduced hand skin temperature are clearly related to the reduction in the fine motor control. The recommendation given by Sanders and McCormick [25] of ambient temperature in the range 413 °C appears to be suitable for gross tracing, where the track widths are comparatively large. When only the hands are cooled, it appears that completion time is impaired and errors with smaller tracks increased at a temperature of 10 °C. These results are in line with physiological changes as well. At 10
°C, the hands are relatively cold and below the threshold of pain (Havenith et al. [40, 41]; Malchaire et al. [42]; Geng et al. [30]). Gaydos and Dusek [36] reported a significant decrease in finger dexterity at a finger temperature of 1013 °C. Lockhart et al. [37] found significant decrements in the performance of psychomotor tasks, such as block stringing, knot tying, and screw tightening, and on the Purdue pegboard when the back of the hands of subjects were air-cooled to 19.1
°C. Tikuisis et al. [38] attributed the decrement in the performance of psychomotor tasks to a possible loss of dexterity at a low temperature.
In addition, the study revealed that small pencils with circular or hexagonal cross sections (having an equivalent diameter of 0.8 m) are better used in tracking task in terms of high movement speed, lower number of errors, and less feelings of discomfort under room temperature. To a certain degree, these results agreed with those of Wu and Luo [43], who found that drawing performance time and errors were significantly lower using pens with diameters of 1.1 and 1.5 cm when compared with those resulting from the use of a 0.55-cm diameter pen. A reasonable explanation for this agreement is that Wu and Luo [43] adopted a track width of
0.3 cm in their experiment, which is similar the present study that used a track width of 0.25 cm. The middle-sized circular or hexagonal pencils (equivalent diameter of 15 mm; 180 mm2) yielded a lower number of errors and were also lower in discomfort ratings. These results contradicted the results of Udo et al. [44], who reported that smaller grips tend to impose a large muscle load, which imply that smaller grips may be related to low pencil control. However, this study proved the contrary fact that smaller grips may instead be linked to better pencil control.
The effect of the use of a pencil with a circular cross- sectional shape did not significantly differ from that with a hexagonal cross section in either movement speed or error scores. These minor differences that did occur showed instead that both cross sections were superior when compared with pencils with an elliptical cross section. The smaller pencil (equivalent diameter of 8 mm) had the lowest error rate measured by the number of excursions outside the marked track per unit length of the track. Errors increased with increasing pencil size, and the time spent for performing the drawing task increased as the pencil size decreased.
Pencil grips should be circular or close to circular. Pencils that do not have a circular cross-sectional shape gave poor subjective ratings on comfort and tracking ability. The findings of this study were similar to those of the study of Goonetilleke and Hoffmann [20]. Subjective ratings improved as the pencil shape approached a circular cross section. Large diameter, heavy pens were found to result in low comfort and tracking ability.
-
CONCLUSIONS
-
-
Most daily tracking tasks require speed as well as accuracy. From these data it is apparent that the selection of a pencil based on speed and accuracy is important; especially, it can be important when accuracy in control is required. The preferences seen in previous studies (Kao [45]) are consistent with the lack of the need for close control and desire for speed. In addition, the use of a smaller pencil size may result in better accuracy along with providing higher movement speed. It is in tasks where there is a need for accuracy, such as in engineering drawing, that the use of smaller sizes of pens may be superior. Hence, in conclusion, it may be remarked that a specification based on a set of standardized tasks may be helpful in the selection of drawing instrument. Such a specification could be valuable to artists and designers who need a specified level of accuracy required in tasks that they often perform. All pencils tested in this study were of the same wood surfaces to elimnate any influence of the material. However, a soft grip surface may affect performance. Finally, despite the authors repeated attempts to recruit females by distributing flyers and pamphlets in the girls section, only male participants responded to the recruitment for the study.
ACKNOWLEDGMENT
The author thanks the Deanship of Scientific Research and RSSU at the King Saud University for their technical support. This work was carried out during the author was working with Industrial Engineering Department, King Saud University.
REFERENCES
-
M. Andrew, J. Arnold, Collaboration, community, identity: Engaged e-learning and eteaching in an online writing course. In G. Williams,
P. Statham, N. Brown & B. Cleland (Eds.), Changing Demands, Changing Directions. Proceedings ascilite Hobart 2011, pp.106-117.
-
L. Pape, K. Ryba, Practical Considerations for School-Based Occupation Therapists. Bethesda, MD: American Occupational Therapy Association, 2004.
-
D. Kiss, Handwriting consultation in elementary schools. OT Practice 2007, pp. 1114.
-
T. Greer, J. Lockman, Using writing instruments: Invariance in young children and adults. Child Dev. 1998, 69(4), pp. 888902.
-
C.I. Chase, Essay test scoring: Interaction of relevant variables. J. Educ. Meas. 1986, 23(1), pp. 3341.
-
H. Kao, Cheuk-Ming, W. Pen pressure in Chinese handwriting. Percept. Motor Skills 1988, 67, pp. 778-778.
-
C. Schneck, Comparison of pencil-grip patterns in first graders with good and poor writing skills. Am. J. Occup. Ther. 1992, 45, pp. 701 706.
-
S. Athenes, Y. Guiard, Is the inverted handwriting posture really so bad for left-handers? In Wann, J., Wing, A., Sovik, N. (Eds.), Development of Graphic Skills: Research, Perspectives, and Educational Implications, Academic Press, London, 1991; pp. 137 149.
-
H.S.R. Kao, Differential effects of writing instruments on handwriting Performance. Acta. Psychol. 1979, Taiwan. 21, pp. 913.
-
V.E. Herrick, Writing tools for children. NEA J. 1961, 51, pp. 4951.
-
C.A. Readdick, Toddlers and preschoolers drawing with primary and standard markers, pencils and crayons. J Res Child Educ. 1994, 9 (1), pp. 6874.
-
J.S. Krzesni, Effect of different writing tools and paper on performance of the third grader. Elem. Engl. 1971, 48(7), pp. 821 824.
-
S. Graham, Issues in handwriting instruction. Focus on Exceptional Children 1992, 25, pp. 114.
-
M.H. Tseng, S.A. Cermak, The influence of ergonomic factors and perceptual- motor abilities on handwriting performance. Am J Occup Ther 1993, 47(10), pp. 919926.
-
J. Ziviani, J. Elkins, Effects of Pencil Grip on Handwriting Speed and Legibility. Educ. Rev. 1986, 38, pp. 247257.
-
M.E. Wiles, Effect of different sizes of tools on the handwriting of beginners. Elem Sch J 1943, 43(7), pp. 412-414.
-
K. Carlson, J. Cunningham, Effect of pencil diameter on the graphomotor skill of preschoolers. Early Child Res Q 5 1990; pp. 279293.
-
E. Oehler, H. DeKrey, E. Eadry, J. Fogo, E. Lewis, C. Maher, A. Schilling, The effect of pencil size and shape on the pre-writing skills of kindergartners. Phys Occup Ther Pedi 2000, 19(3/4), pp. 5360.
-
S.J. Amundson, Prewriting and handwriting skills. In J. Case-Smith (Ed.), Occupational Therapy for Children 2005, St. Louis: MO: Elsever Mosby, pp. 587610.
-
R.S. Goonetilleke, E.R. Hoffmann, A. Luximon, Effects of pen design on drawing and writing performance. Appl Ergon 2009, 40, pp. 292- 301.
-
C.G. Drury, Movements with lateral constraint. Ergonomics 1971, 14 (2), pp. 293305.
-
R.S. Goonetilleke, E.R. Hoffmann, Hand skin temperature and tracking performance. Int. J Ind Ergon 2009, 40, pp. 314325.
-
R. Morton, K.A. Provins, Finger numbness after acute local exposure to cold. J Appl Physiol 1960, 15, pp. 149154.
-
R.R. Bishu, G. Klute, B. Kim, Investigation of the effects of extra vehicular activity (EVA) gloveson performance. in the Advances in Industrial Ergonomics and Safety V (Editors: Nielson and Jorgensen), Taylor and Francis, London, 1993, pp 683-690.
-
M.S. Sanders, E.J. McCormick, Human Factors in Engineering Design. McGraw-Hill, New York.
-
D. Brajkovic, M.B. Ducharme, Finger dexterity, skin temperature, and blood flow during auxiliary heating in the cold. J Appl Physiol 2003, 95 (2), pp. 758770.
-
G.G. Giesbrecht, W. Wu, C.E. Johnston, G.K. Bristow, Isolated effects of peripheral arm and central body cooling on arm performance. ASEM 1995, 66, pp. 968975.
-
L. Lamme, B. Ayris, Is the handwriting of beginning writers influenced by writing tools? J. Res. Devel. Educ. 1983, 17, pp. 3238.
-
M.Z. Ramadan, The Effects of Industrial Protective Gloves and Hand Skin Temperatures on Hand Grip Strength and Discomfort Rating. Int.
J. Environ. Res. Public Health 2017, 14(12), 1506. doi:10.3390/ijerpp4121506
-
Q. Geng, I. Holmer, D.E.A. Hartog, G. Havenith, O. Jay, J. Malchaire, J., A. Piette, H. Rintamaki, S. Rissanen, Temperature limit values for touching cold surfaces with the fingertip. Ann Occup Hyg 2006, 50, pp. 851862.
-
L. Groenesteijn, S.M. Eikhout, P. Vink, One set of pliers for more tasks in installation work: The effects on (dis)comfort and productivity. Appl Ergon. 2004; 35(5): 485492.
-
C.G. Drury, B.G. Coury, A methodology for chair evaluations. Appl Ergon 1982; 13: 195202.
-
G. Keppel, Design and Analysis: A research's Handbook, 4th Ed. New Jersey: Englewood Cliffs Inc. 2004.
-
S.S. Shapiro, M.B. Wilk, An analysis of variance test for normality. Biometrika 1965; 52: 591611.
-
S.R. Srinivasan, Significance of Mental Rehearsal on Hand-Eye Coordination. M.Sc. Theses Presented to the Department of Health, Physical Education, Recreation, and Dance. North West Missouri State University, Maryville: MO, 2011.
-
H.F. Gaydos, E.R. Dusek, Effects of localized hand cooling versus total body cooling on manual performance. J Appl Physiol 1958, 12, pp. 377380.
-
J.M. Lockhart, H.O. Kiess, T.J. Clegg, Effect of rate and level of lowered finger surface temperature on manual performance. J Appl Physiol 1975, 60, pp. 106113.
-
P. Tikuisis, A.A. Keefe, J. Keillor, S. Grant, R.F. Johnson, Investigation of rifle marksmanship on simulated targets during thermal discomfort. ASEM 2002, 73, pp. 11761183.
-
D. Brajkovic, M.B. Ducharme, J. Frim, Relationship between heat content and finger temperature during cold exposure. J Appl Physiol. 90, 2445-2452.
-
G. Havenith, E.J.G. van de Linde, R. Heus, Pain, thermal sensation and cooling rates of hands while touching cold materials. Eur J ApplPhysiol 1992, 65, pp. 4351.
-
Havenith, G.; Heus, R.; Daanen, H.A. The Hand in the Cold, Performance and Risk. Arctic Med Res 54 (Suppl. 2), 1995; pp. 3747.
-
J. Malchaire, Q. Geng, E. Den Hartog, G. Havenith, I. Holmer, A. Piette, S.L. Powell, H. Rintamaki, S. Rissanen, Temperature limit values for gripping cold surfaces. Ann Occup Hyg 2002, 46, pp. 157 163.
-
F.G. Wu, S. Luo, Design and evaluation approach for increasing stability and performance of touch pens in screen handwriting tasks. Appl. Ergon. 2006, 37 (3), pp. 319327.
-
K. Udo, T. Otani, A. Udo, Yoshinaga, F. An electromyography study of two different types of ballpoint pens. Ind. Health 2000, 38, pp. 47 56.
-
H.S.R. Kao, Ergonomics in pen point design, Acta. Psychol. 1997, Taiwan, 18, pp. 4952.