
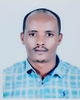
- Open Access
- Authors : Getnet Adugna , Brook Abate
- Paper ID : IJERTV10IS010035
- Volume & Issue : Volume 10, Issue 01 (January 2021)
- Published (First Online): 11-01-2021
- ISSN (Online) : 2278-0181
- Publisher Name : IJERT
- License:
This work is licensed under a Creative Commons Attribution 4.0 International License
Flood Modeling of Jemo River Catchement in Addis Ababa City, Ethiopia
Getnet Adugna1, a*
1Masters Student at Department of Civil Engineering, College of Architecture and Civil Engineering,
Addis Ababa Science and Technology University, Addis Ababa, Ethiopia
Brook Abate2, b
2Associate Professor at College of Architecture and Civil Engineering, Addis Ababa Science and Technology University, Addis Ababa, Ethiopia
Abstract:- Flood affects lives and livelihoods in parts of Ethiopia. The flood from the Jemo river is causing damages to river side houses, infrastructures and displacement of affected population that resulted overflow on the surface following heavy rains and inundated lowland areas in the Nifas Silk Lafto district of Addis Ababa city, Ethiopia. This research involves the integration of Hydrologic Engineering Center-Hydrologic Modeling System (HEC-HMS) and Hydrologic Engineering Center-River Analysis System (HEC-RAS) to develop a two- dimensional (2D) river model for flood inundation determination and mapping. The model Nash-Sutcliffe efficiency (ENS) was found to be 0.751 during calibration and
-
during validation and the coefficient of determination (R2) was found to be 0.786 during calibration and 0.801 during validation. The HEC -RAS model was calibrated by comparing the results of the water level in each selected cross section obtained by the model with the observed historic flood mark levels of the year 2010 and 2013. The peak estimated time series discharge of HEC HMS model result was used to simulate the unsteady state of flow to determine flood extent, water depth and velocity of the study river. Flood hazard vulnerable areas both left and right side of the Jemo river delineated for the return periods 2, 5, 10, 25, 50 and 100 years. The research showed community houses ranging from mud houses to regular story buildings can be affected during each return period maximum flooding event. Jemo river flow capacity also checked at different cross sections and less carrying capacity sections identified for recommended flood protection measures like dyke and retaining masonry walls construction to tackle the flooding impact and avoid possible erosion of the river banks.
Key words: Hydrodynamic model, HEC RAS 2D flood modeling, RAS Mapper, GIS, HEC HMS, Jemo river
-
INTRODUCTION
Flooding is the most common natural hazard that can happen any time in wide variety of locations due to high intensity rainfall events. Floods can be explained as excess flows exceeding the transporting capacity of River channel, lakes, ponds, reservoirs, damage system, dam and any other water bodies, whereby water inundates outside water body areas [1]. Flood affects lives and livelihoods in parts of Ethiopia. Especially during the rainy season (June-September), the major perennial rivers as well as their numerous tributaries forming the countrys drainage systems carry their peak discharges. Floods are already having very large impacts on cities and smaller urban centers in many African nations for instance the floods in Mozambique in 2000 which included heavy floods in Maputo, the floods in Algiers in 2001(with around 900 people killed, and 45,000 affected); heavy rains in East Africa in 2002 that brought floods and mudslides
forcing tens of thousands to leave their homes in Rwanda and the very serious floods in Port Harcourt and in Addis Ababa in 2006 [2].
Flash flood from the Jemo river is causing damages to houses, infrastructures and threat to loss of life for human beings every year in Nifas silk lafto sub city of Addis Ababa city. The need for delineating flood risk areas and determining its magnitude is quite important in order to take required protection measures.
HEC-HMS and HEC-RAS Computer modeling software is used for determination of river characteristics as well as delineation of flood affected areas to indicate the extent of flooding arising from the river considering different return periods.
-
MATERIALS AND METHODS
-
Description of the study area
The Jemo river catchment is located between 8057.192 N Latitude and 38040.923 and 38043.728 Longitude, the river catchment delineated and covers an area of 12.67km2. The main river course is located centrally in the catchment generally creating a V-shaped terrain profile.
Figure 1: Study area map
-
HEC-HMS model setup
The Hydrologic Engineering Centers Hydrological Modeling System (HEC-HMS) has four main model components: basin model, meteorological model, control specifications and input data (time series, paired data and gridded data). The Basin Model, for instance, contains information relevant to the physical attributes of the model, such as basin areas, river reach connectivity, or reservoir data. Likewise, the Meteorological Model holds rainfall
data. The Control Specifications section contains information pertinent to the timing of the model such as when a storm occurred and what type of time interval is to be used in the model, etc. Finally, the input data component stores parameters and boundary conditions for basin and meteorological models [3].
Watershed delineation done at selected river outlet and required physical parameters for the HEC HMS model collected.
-
HEC -RAS Model Development
The three steps to run the Hydrologic engineering center river analysis system (HEC RAS) model are pre-processing of data, modeling phase, and post processing of data [4].The first step in developing hydraulic model HEC -RAS is to establish which directory the researcher wishes to work in and enter a title for the new project. Then, the river cross section at each location will be opened and geometric data called by Geographical information system (GIS) format and edited. And all RAS layers like stream center line, bank lines; bank stations, flow path center lines, cross-section cut lines, and others were generated from DEM of the study area.
The discharge values for different return periods can be entered manually for unsteady flow. The roughness coefficients (Mannings coefficient) and boundary
-
-
MODEL CALIBRATION AND VALIDATION
-
Model calibration
-
HEC HMS model calibration
A total of Sixteen years (2000-2015) metrological data and Twenty-seven-years (1989-2015) was collected from Ministry of Water, Irrigation and Energy and National Metrological Agency respectively and Nine years data (2000 to 2008) are used for calibration. Validation of the model was done for the years (2008-2015)
-
HEC RAS model calibration
-
In the calibration, Mannings coefficient n was altered continually until the variations between observed and simulated flood water level marks for the year 2010 and 2013 were within the acceptable limits. The calibration was performed by comparing the results of the water level in each selected cross section obtained by the model with the observed historic flood mark levels of the year 2010 and 2013.By starting with an initial value of mannings roughness coefficient suggested by ERA technical manual, the n value was altered until the differences between observed and simulation water levels became small as far as possible. So, the outcomes of the HEC-RAS model for distinct values of n were compared with the observed water surface profile.
=
=
conditions were added to the model. The values selected for
mannings coefficient were 0.05 and 0.17,
for the stream channel and overflow banks, respectively referring Ethiopian roas authority (ERA) standard
RMSD=
1( ) 2
Equation-51
(1)
document and fixed during calibration. The model was run for mixed flow regime conditions and unsteady flow water surface profile computations. The iterative solution of the energy equation, using the standard step method, solved the unsteady flow, while Mannings equation and contraction/expansion coefficients determined head losses before applying the computation process the model set up for boundary condition. There are various methods of boundary condition used. The method used in this paper is the Normal depth at the downstream end of the reach. The model calculates the depth from the given elevation data and discharge. Finally, the plan must be established for each model simulation.
2.4 Terrain Preprocessing
2.4.1 HEC-GeoHMS
HEC GeoHMS allows to preprocess terrain in two approaches either step by step or batch mode. In this research step by step process was used in order to examine out puts and made necessary corrections.
HEC-GeoHMS is a set of ArcGIS tools specifically designed to process geospatial data and create input for the
Where, RMSD is the root mean square deviation
is simulated water depth at cross section i
is observed flood mark depth at cross section i
N is the total number of data (total number of cross sections).
3.2 HEC HMS & HEC RAS model evaluation
The performance of selected model was evaluated using statistical measures to determine the quality and reliability of predictions when compared to observed values. Coefficient of determination (R2) and Nash-Sutcliffe simulation efficiency (ENS) were the goodness of fit measures used to evaluate model prediction. The R2 value is an indicator of strength of relationship between the observed and simulated values. The Nash-Sutcliffe simulation efficiency indicates how well the plot of observed versus simulated value fits the 1:1 line. If the measured value is the same as all predictions, ENS is approximately1. If the ENS is between 0 and 1, it indicates deviations between measured and predicted values. If ENS is negative, predictions are very poor, and the average value of output is a better estimate than the model prediction. The R2 and ENS values are explained in equations below.
HEC-HMS. HEC-GeoHMS provides the connection for
2 = [()()]
(2)
2
2
() 2 () 2
translating GIS spatial information in to model files for
Where:
=1
HEC-HMS. The GIS capability is for data formatting, processing and coordinate transformation. Currently, HEC- GeoHMS operates on DEM to derive sub-basin delineation and to prepare a number of hydrologic units. HEC-HMS supports these hydrologic inputs as starting point for hydrologic modeling.
qsi: – is the simulated value, qoi: – is the measured values, qs: – is the average simulated value and qo: – is the average measured value
The ENS simulation efficiency for n time steps is calculated as:
())2
= 1 =1 . (3)
=1
() 2
Where: qsi is the simulated value and qoi is the measured value.
The proposed model of this study was calibrated by adjusting sensitive parameters and validation was done using observed data of the existing gaging station.
-
-
RESULTS AND DISCUSSIONS
-
-
Study area basin characteristics
ARC-GIS 10.4 modeling software is used to delineate catchment for different river system in the Big Akaki and Jemo river at selected outlet catchment and hydrological, physical parameters and spatial information of the catchments were obtained. Road Networks, houses and buildings as well as vegetation cover of the catchment is digitized and corresponding areas is calculated.
Figure 2: Study area watershed delineation
Hydrological parameters of the study area extracted and curve number (CN), water shed area, soil types obtained and used to run the model. The study area soil, land use characteristics and curve number (CN) were extracted during terrain processing using GIS extensions (Arc Hydro and HEC-GeoHMS).
-
Best fit flood probability distribution
The test statistic for yearly maximum precipitation and discharge data of selected study area outlet was analyzed using EasyFit software and best fit probability distributions among Normal, Log Normal, General Extreme Value, Chi- Squared and Log Pearson 3 identified.
The five probability distributions were compared using Kolmogorove Smirnov,Anderson Darling and Chi-Squared test statistic using EasyFit software. The rank with respective test statistic listed and accordingly, General Extreme Value distribution rank first using Kolmogorov Smirnov and Anderson Darling statistic and Normal distribution rank first using Chi-Squared statistic
Figure 3: Goodness of fit comparison
4.2 HEC HMS hydrological model result
4.2.1 Calibration and Validation
Calibration is adjusting of model parameters based on checking results against observations to ensure the same response over time. This involves comparing the model results, generated with the use of historic meteorological data, to recorded stream flows. The calibration of HEC- HMS for this particular study area was carried out using nine years from 2000-2008 daily rainfall and daily stream flow data of nearby Akaki river.
Optimization of the parameter values was carried out within the allowable ranges recommended by the US Army corps of Engineers Hydrologic Engineering Center [5]. Coefficient of determination(R2) (0.68 to 0.88 for Calibration and 0.62 to 0.86 for Validation and Nash- Sutcliffe efficiency (ENS) 0.5 to 0.88 for Calibration and
0.61 to 0.85 for Validation).
The Nash-Sutcliffe efficiency (ENS) was found to be 0.751 during calibration and 0.79 during validation. The stochastic nature of precipitation effect on the simulated hydrograph was handled by stochastic calibration. In the stochastic calibration observed and simulated discharge time series were arranged in descending order and the objective function, (Qobs-Qsim) ² was minimized by observing the plot of observed and simulated discharge.
Figure 4: Observed and Simulated flow time series
Observed flow and simulated flow time series graph obtained during calibration and validation was significantly within the recommended Nash-Sutcliffe efficiency and the time series graph matches with the observed flow.
Correlation of simulated & observed flow-calibration
y = 0.977x – 0.3092
R² = 0.786
200
150
100
50
0
0 50 100 150
Simulated Flow
Correlation of simulated & observed flow-calibration
y = 0.977x – 0.3092
R² = 0.786
200
150
100
50
0
0 50 100 150
Simulated Flow
y = 0.8004x + 17.82
R² = 0.801
y = 0.8004x + 17.82
R² = 0.801
Observed Flow
Observed Flow
Observed Flow
Observed Flow
Figure 5: Correlation of flow (calibration)
Corelation of simulated & observed flow -validation
300
200
100
0
Corelation of simulated & observed flow -validation
300
200
100
0
0 100 200 300 400
Simulated Flow
0 100 200 300 400
Simulated Flow
Figure 6: Correlation of flow (validation)
The coefficient of determination (R2) was found to be 0.786 (Figure 4) during calibration and 0.801 during validation (Figure 5). The relationship of simulated and observed flow found to be good and coefficient of determination and Nash- Sutcliffe matches as per the standard indicated above.
-
HEC HMS model result
The calibrated and validated HEC HMS model was used to estimate peak flood magnitude of the Jemo river selected outlet are using rainfall depth calculated by the best fit distribution for respective return periods. The 24hour depth frequency curve values were used from the Ethiopian Roads Authority drainage manual [6]. Accordingly, the output of the peak flow result for each return period was found as in the below Table 1.
Return Period, years
Peak flood, M3/s
2
39.2
5
43.8
10
47
25
51.2
50
54.3
100
57.5
Table 1: HEC HMS peak flow result
-
HEC RAS model result
The outcomes of the HEC-RAS model for different values of Mannings roughness coefficient (n) were compared with the observed historic water surface flood level marks collected from specific site which occurred during the year 2010 and 2013 as shown in the Table 2 and represented in Figure 7.
Table 2: Observed and simulated water level
i
Cross section
Flood Water Level Observed
Water level simulated
Simulated for n=0.17
Simulated for n=0.05
Simulated for n=0.01
1
3314
2271.150
2272.850
2271.713
2271.781
2
3146
2266.000
2266.150
2265.972
2265.898
3
2829
2264.720
2264.640
2264.695
2264.654
4
2578
2260.820
2261.031
2261.000
2261.003
5
2202
2256.370
2256.461
2256.061
2255.011
6
1877
2251.240
2252.000
2251.450
2253.000
7
1142
2240.350
2240.102
2240.121
2240.123
8
902
2238.870
2239.843
2239.651
2239.843
9
685
2235.720
2235.501
2235.479
2235.446
10
304
2230.000
2230.531
2230.500
2230.530
11
183
2229.750
2230.512
2230.542
2230.512
12
11
2229.300
2229.678
2229.664
2229.652
2275.000
2270.000
2265.000
Water Surface Level
Water Surface Level
2260.000
2255.000
2250.000
2245.000
2240.000
2235.000
2230.000
2225.000
Observed and Simulated Water Surface profile
Distance,m
0 500 1000 1500 2000 2500 3000 3500
Flood Water Level Observed,m Water level simulated Simulated,n=0.17
Water level simulated Simulated,n=0.05 Water level simulated Simulated,n=0.01
Figure 7: Observed and simulated water surface profile
The root means square deviation (RMSD) was tested between observed and simulated water surface elevations as shown in the Table 3.
Manning's Roughness Coefficient (n)
0.17
0.05
0.01
RMSD
0.48387473
0.140726389
0.618011124
Table 3: RMSD for Mannings n
Mannings coefficient with low differences of RMSD were selected and used for the model.
Using ERA drainage manual left and right-side banks and channel section roughness coefficient estimated and calibrated with the HEC RAS model until historic flood marks observed and simulated water depths difference significantly reduces using root mean squared error deviation approach.
Based on the calibration result Manning coefficient of 0.05 for the channel and 0.05 and 0.17 for selected left and right river bank sides were chosen at downstream and upstream section to run the model.
-
2D flow area, water depth and water surface elevation
The river two-dimensional (2D) flow area polygon was drawn using aerial imagery and existing terrain data. The 2D flow area mesh generation made and boundary conditions fixed at upstream and downstream locations. Time series flow hydrograph output of calibrated HEC HMS model for 2,5,10,25,50 and 100 years return period was used as an input for boundary conditions for channel and 2D flow area. The unsteady state simulation model run and depth of flow, water surface elevation and velocity for different return periods of the study river area generated.
Figure 8: 2D flow area of the Jemo river
Figure 9: 100 years return period unsteady simulation water surface elevation The 100-year return period simulated water depth simulated
high at river stations 902m and 595m from selected outlet and similarly simulated water depth for 50,25,10,5 and 2 years estimated with the HEC RAS model.
The river analyzed at different cross sections and less carrying capacity of the river observed at low elevations where there are community settlements very close to the existing river.
-
Flood hazard water surface extents & velocity map
Water surface extents of the river for each return period was generated with GIS extension (HEC GeoRAS) tool. Areas flooded both left and right side of the river for respective
Figure 10: 100-year flood hazard and velocity map
return period delineated and shown as in the Figure 9. The 100-year return period result showed that majority of left
side of the river where communities are settled in close proximity is vulnerable and can be affected by the flood. It is observed that the downstream culvert found around Anbessa Garage is overtopped by flash water during rainy season and cause problem to normal traffic movement. Storm water drainage which mark and will have great impact to erode the existing soil at concerned locations. The flood model simulated flow velocity of magnitude less than 2 m/s for major sections of the river and exclusively river stations at
183m,435m,595m,1753m and 3252m found to have flow velocity more than 2m/s and between 3-5m/s.
-
Flood hazard vulnerability
Flooding area vulnerable for different return period also identified with the model and identified as shown
With respect to river sections. Communities settled very close to the river upstream of the Jemo 01 condominium houses and newly built private houses are susceptible by the recurrence flood that can happen any time to cause potential harms to the human lives and livelihoods.
Table 4: 100-year flooding area
River station
Flooding Area (m2)
Remark
11 m to 821m
1809.64
Upstream of Jemo Anbessa Garage area
902m to 2433m
4310.1
Upstream of Jemo 01 condominium houses
2483m to 3314m
2106.87
Total Area, M2
8,226.61 M2 (0.82ha)
-
Flood damage estimate
Using HEC RAS model flood inundated area and overlaying the layer on to the latest google satellite image and Addis Ababa maser plan, specific river cross sections that were affected by the flood delineated and specific areas also calculated. In order to estimate cost of flood damage an average unit price of birr 12,500 per square meter were assumed and used to estimate the probable damage that can be caused by the model flood. The following table shows residential buildings at respective river cross section that was affected by flood of more than 2m water depth and estimated cost of flood damage.
Return period
Residential buildings area covered by flood at River cross section stations
Estimated unit damage price for buildings, Birr/M2
Estimated residential building flood damage cost, Birr
183m-356m
1926m-1518m
2742m-
3071m
12,500
2-years
212
716
555
17,796,000.00
5-years
271.45
748
635
19,853,400.00
10-years
283
846
630
21,108,000.00
25-years
248
780
615
19,716,000.00
50-years
289
798
855
23,304,000.00
100-years
325
650
1015
23,880,000.00
Table 5: Flood damage estimate cost
The HEC RAS flood model showed that for the 2 year return period the flood can cover an area of 1,483 m2 community properties of majority residential buildings and similarly an area of 1,654m2 , 1,759m2 ,1,643m2 ,1,942 m2,1,990m2 can
be affected by flood of return period 5,10,25,50 and 100 years respectively. Flood damage of birr 17,796,000 for 2 years return period 19,853,400 for 5 years,21,108,000 birr for 10 years ,19,716,000 birr for 25 years, 23,304,000 birr for 50 years and 23,880,000 birr for 100 years estimated in this study and the flood damage can be more of this if human life and detail livelihoods assets were considered which were not conducted in this study.
5.-CONCLUSSION
Best fit flood probability distribution for the study area identified based on how good the distribution was fitted to the data. Easy Fit software was applied to evaluate goodness of fit at various significance level. The analysis resulted the General Extreme value probability distribution as best fit probability distribution for the study area among Normal, Log Normal, Chi-Squared and Log Pearson 3 probability distributions.
The study presents a systematic approach in the preparation of Two-dimensional (2D) flood model of the Jemo River found in the Nifas Silk Lafto woreda of Addis Ababa city with the application of hydrodynamic models and GIS software. Two dimensional (2D) unsteady flood simulation was done using HEC RAS 5.0.6, Arc GIS 10.4 and HEC GeoRAS GIS extensions and HEC HMS hydrological models.
Peak flood discharge estimated using HEC-HMS software application and ERA Intensity Duration Curves for different return periods (100,50,25,10 and 2). The 100-year return period peak flood was found 57.5 m3/s and 54.3 m3/s, 51.2 m3/s, 47 m3/s, 43.8 m3/s and 39.2 m3/s for 50, 25, 10, 5 and 2-year return period respectively.
The simulated flood map showed that large flooding is observed near the sides of Jemo 01 condominium residential areas and upstream area where communities settled very near to existing river reach. The total area affected by the 100-year return period flood was 8,226.61 m2 (0.82ha) and 10,383.18 m2 (1.04ha), 11,383.16 m2 (1.14ha), 11,562.84 m2 (1.15ha), 10,527.96 m2 (1.05ha), 8,992.74 m2 (0.89ha) were
affected areas simulated for 50, 25,10,5 and 2 years return period consecutively.
The simulated depth of water was found in between 0 to 6m for the return periods and varied along the sections depending with the specific terrain. The flow velocity upstream of Jemo 01 condominium areas and upstream sections of the reach were higher than 2.0m/s and this can lead to erosion of the soil and collapse of the existing river banks to cause further flood vulnerability of communities residing closer to the reach. The hydrologic response of the river was for the river carrying capacity of each return period was checked and locations with less carrying capacity for probable maximum flow were at low elevations and river cross sections at 1142m and 435m, 2899m,902m,595m as discussed in the result section.
The study flood model showed that communities upstream of the reach and around Jemo 01 residential areas were identified within the flooding area and can be affected badly if no immediate measure is taken to tackle the probable flooding problem. Based on the flow simulation result of this research the decision makers should consider structural measures such as construction of dike and retaining walls at low elevations along the river which would prevent the flooding caused by over toping.
ACKNOWLEDGEMENT
First and for most, I would like to thank my Almighty God for his care, limitless love and support during my study in Addis Ababa Science and Technology University and reach this point in my life. I would like to express my sincere gratitude to my Advisor, Dr. Brook Abate for his persistent support and guidance throughout this study work. I am grateful to Ethiopian Roads Authority for granting this sponsorship program. My appreciation also goes to Ministry of Water, Irrigation and Energy and National Metrological Agency for providing me necessary data to conduct thesis work.
Finally, I would like to extend my deepest gratitude to my family, for there encouragement and moral support without your encouragement and care this would not have been possible.
REFERENCE
-
Aris MM (2003). Modeling for river and tidal flood hazards in waterfront city case study in samurangu city, Java, Indonesia.
-
HABITAT UN (2007). Situation analysis of informal settlements in Addis Ababa city.
-
US Army corps of engineers, Technical manual (2006).
-
Technical reference manual, US Army corps of engineers (2000).
-
Patel, C.G. and P.J. Gundaliya (2016). Floodplain Delineation Using HECRAS Model.
-
Ethiopian Roads Authority Technical manual (2013).
-
-