
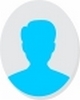
- Open Access
- Authors : El-Sayed M. El-Refaie , Sherin El Essawy , Elham M. Hegazi, Mohammed Fayiz , Amal Abdel Razek El-Gamel, Karim El-Adham
- Paper ID : IJERTV10IS080070
- Volume & Issue : Volume 10, Issue 08 (August 2021)
- Published (First Online): 10-12-2021
- ISSN (Online) : 2278-0181
- Publisher Name : IJERT
- License:
This work is licensed under a Creative Commons Attribution 4.0 International License
Gamma Shielding Properties for Rock Fiber Reinforcement Concrete for Nuclear Applications
El-Sayed M. El-Refaie a Sherin El Essawy b, Elham M. Hegazi b , Mohammed Fayizc,
Amal Abdel Razek El-Gamel d, Karim El-Adham e
a Faculty of Engineering, Helwan Univ., Cairo, Egypt
b QC and QA Depart., Egyptian Atomic Energy Authority, Cairo, Egypt
c Exp. Nucl. Phys. Dep., N. R. C., Egyptian Atomic Energy Authority, Cairo, Egypt d Radiation Safety Department, Egyptian Atomic Energy Authority, Nasr City, Egypt e Egyptian Atomic Energy Authority, Cairo, Egypt
Abstract:- In this study, the linear attenuation coefficient (µ cm-1), half value layers (HVL) has been modified and transmission curves have been plotted with different loading of Rock Fiber (RF) by weight (0, 2, 7.5, 12.5, 17.5 and 30) % were studied in gamma radiation shielding application. The Monte Carlo N-Particle (MCNP) transport code and XCOM were used to verify the experimental results of concrete with and without different percentages of RF. Measurements were carried out using 70% High Purity Germanium (HPGe) detector at different gamma radiation energies (185, 240.7, 294, 305.78, 608.7, 1121 and 1767.6) keV for radiation shielding design. The results concluded that the linear attenuation coefficient (µ) of the all prepared samples increase with increasing the rock fiber content.
Keywords: Concrete, Rock Fiber, Linear Attenuation Coefficient, Half Value Layers, XCOM, MCNP5.
-
INTRODUCTION
Shielding from ionizing radiation plays a vital role in protecting the man and environmental from these radiation. Nuclear safety depends on the safe operation of all parts and components of a Nuclear Power Plants (NPP) during its designed operational life. One of the important aspects of safe operation is ensuring the safe operation of fixed parts during its life, in addition to minimizing the radiation levels in the surrounding environment. Different types of concretes, such as ordinary concrete, heavy weight concrete (e.g., barite, serpentine, steel magnetite, limonite, magnetite) etc. are used in the manufacturing of these fixed parts depending on the energy, radiation types [1]. In recent years, several studies had been made for different construction materials used for gamma radiation shielding [2-6]. Cracks are a major cause of deterioration in concrete structures in a nuclear power plant [7]. Cracks lead to moisture penetration into the concrete resulting in its gradual destruction. Previous studies indicate that reinforced concrete with fiber reduces the rate of crack formation in reinforced concrete. Recently, other types of fibers such as Polymer Fibers and Basalt Fibers have been developed and produced for use in the concrete reinforcement of a nuclear power plant resulting in increased safety for the surrounding environment of nuclear power. Basalt Fibers reinforced concrete are a new construction materials established through broad research and progress during the past years. The addition of fibers to concrete had proved the enhancement the properties of concrete structures as tensile and shears strength, fracture toughness and crack resistance [8 – 12]. Basalt Fibers have different properties from other high-tech fibers (Polypropylene Fiber, Carbon Fibers, Organic Fibers, etc.) due to its high chemical and thermal stability and do not produce any harmful gases. Basalt Fiber has a strength that far exceeds other natural and synthetic fibers and it also greatly meets the requirements of environmental protection. Basalt Fibers have a high modulus of elasticity that is very similar to the modulus of elasticity of Carbon Fibers [13]. The aim of this study is to determine the shielding properties of local Rock Fiber reinforced concrete which will help in the shielding design. Different gamma radiation energies (185 1767.6) keV were used to determine attenuation parameters experimentally and theoretically.
-
EXPERIMENTAL
2.1 Used Materials
Ordinary Portland cement was used. The chemical composition of cement is shown in Table 1. The coarse and fine aggregates are chosen according to the Egyptian requirements and ASTM C33 (2013). The water used for all the concrete mixes are normal drinking water. Rock fiber used in this work is provided from a local factory Egyptian Rock Wool Factory, Cairo, Egypt. All materials used are locally produced.
The concrete samples were casted in the laboratories with rock fiber of 6.5 m diameter and 0.5 mm length with different weight ratios (2, 7.5, 12.5, 17.5 and 30) % Table 2. Samples formed as disks with 2 cm thickness were used to study radiation shielding properties Fig. 1.
Table 1: Rock Fiber Chemical Composition
Sio2
AlO3
Fe2O3
MgO
Na2O
K2O
TiO2
49.79%
14.02%
10.23%
5.25%
1.83%
1.01%
2.66%
Table 2: The mix proportions of all attenuation specimens.
Mix No
Cement
Fine Aggregate
Coarse Aggregate
RF (wt %)
of Cement
1
350
656
583
0
4
348
656
583
2
5
342.5
656
583
7.5
6
337.5
656
583
12.5
7
332.5
656
583
17.5
8
320
656
583
30
Fig. 1: Prepared Samples with Rock Fibers
2.2. Measurement of Gamma Ray Intensity
The linear attenuation measurements of the prepared Concrete/ RF samples with thickness 2 cm were carried out using narrow beam gamma-ray transmission geometry. Radium-226 source was used to apply strong and well resolved gamma rays of energies (185, 240.7, 294, 305.78, 608.7, 1121, 1767.6) keV.
A high-resolution spectroscopic system (in Nuclear Reaction Units Laboratory at Atomic Energy Authority, NRC, Inshas) is used for the measurement of the energy spectrum of the emitted gamma rays Fig.2. The system consists of a 70% Hyper-Pure Germanium (HPGe) detector (closed end coaxial cylinder of 54.5 mm in diameter and 49 mm in length). The detector is mounted on a cryostat which is dipped into a 30-L Dewar filled with liquid Nitrogen. The detector is surrounded by a special heavy lead shield cylinder of 5 cm thickness with 1 cm Copper layer inside, which provides an efficient suppression of background gamma radiation present at the laboratory site.
Figure 2: Experimental measurements of -ray attenuation coefficient
The primary data came from the counter registering the gamma-ray intensity. The incident intensity (I0) of the gamma ray was reduced to intensity (I) after the passing through the specimens. The Radiation shielding parameters like the linear attenuation coefficient is obtained values using Beer-Lambert's equation (1) as follows [14];
I = Io e x (1)
where I0 and I are the unattenuated and attenuated photon intensities respectively, and µ(cm-1) is the linear attenuation coefficient of the material.
Once the linear attenuation coefficients values were determined, additional radiation shielding parameers, such as the Half- Value Layer (HVL), were used to evaluate the gamma ray shielding ability of the prepared samples. The HVL values represent the thickness of an absorber that will reduce the gamma radiation intensity to one-half of its original value, as the following equation (2):
HVL = ln2/µ (2)
-
THEORETICAL CALCULATION
XCOM and MCNP5 are used to calculate the linear attenuation coefficient and Half-Value Layer.
-
(XCOM)
XCOM program is a calculation program and its input parameter specifications are quite flexible and easy to access. First, the types of shielding material were determined by elementary mass fractions. XCOM is used to evaluate cross-sections of photon for scattering, photoelectric absorption and attenuation coefficients for energies ranging from (185 1.767.6) keV. Then, the shielding parameters were determined by elementary mass fractions. The total attenuation factors for all components are calculated as the sum of the quantities of the corresponding atomic components. Then the defined weights are calculated as fractions of the weight of the components by XCOM from the chemical formula [13].
-
Radiation Shielding Modeling MCNP
MCNP is a general code used for modeling interactions of radiations with materials and tracking all particles at all energies. Being fully 3D, it uses a random nuclear cross-section and uses physical models of particle types [15]. In order to confirm a validation of modeled MCNP5 code for the used geometry. The experimental geometry was simulated using MCNP5 Monte Carlo code as in Fig. 3, which was used to calculate the attenuation parameters for the concrete composites. The gamma rays are collimated by the lead shielding, attenuated by the sample of the Concrete with different weight ratio (0, 2, 7.5, 12.5, 17.5 and
30) % RF and then collimated again and detected by the detector.
Fig. 3: experimental geometry simulated using MCNP5 Monte Carlo code
-
-
RESULTS AND DISCUSSION
The linear attenuation coefficients were calculated in photon energy range from (1851767.6) keV. The values of the linear attenuation coefficients for concrete samples with different ratio of Rock Fiber are listed in Table 3. It shows that linear attenuation coefficient increases with fiber loading for all photon energies, and decrease with the increase of gamma ray energy. This is due to different interaction mechanism of photons at different energies. The strength of these interactions depends on the energy and the elemental composition of the material, but not much on chemical properties, since the photon energy is much higher than chemical binding energies. [16]. Fig. 4 shows a linear attenuation coefficients for concrete samples with (0, 2, 17.5 and 30) % RF, which recorded the highest linear attenuation values. The Half-Value Layer (HVL) thicknesses were calculated using Eq. (2) for gamma shielding. HVL thicknesses for concrete specimens as a function in Rock Fiber ratios are shown in Table 4. As it can be seen, the required thickness decreases with increases in Rock Fiber ratio.
Table 3: Linear Attenuation Coefficients (cm-1) for the prepared concrete/RF samples.
-ray energy(keV) |
||||||||
RF % |
185 |
240.7 |
294 |
305.78 |
608.7 |
1121 |
1767.6 |
|
0 |
0.207 |
0.203 |
0.2011 |
0.179 |
0.159 |
0.073 |
0.073 |
|
2 |
0.273 |
0.252 |
0.2136 |
0.217 |
0.1738 |
0.1417 |
0.0962 |
|
7.5 |
0.097 |
0.185 |
0.179 |
0.156 |
0.147 |
0.08 |
0.075 |
|
12.5 |
0.1615 |
0.2 |
0.194 |
0.188 |
0.152 |
0.1002 |
0.1002 |
|
17.5 |
0.23 |
0.227 |
0.214 |
0.213 |
0.16 |
0.117 |
0.117 |
|
30 |
0.347 |
0.327 |
0.278 |
0.23 |
0.1775 |
0.115 |
0.115 |
0 2 17.5 30
0 2 17.5 30
0.4
0.35
0.3
0.25
(cm-1)
(cm-1)
0.2
0.15
0.1
0.05
0
0 500 1000 1500 2000
Photon Energy, keV
RF % |
-ray energy(keV) |
||||||
185 |
240.7 |
294 |
305.78 |
608.7 |
1121 |
1767.6 |
|
0 |
0.207 |
0.203 |
0.2011 |
0.179 |
0.159 |
0.073 |
0.073 |
2 |
0.273 |
0.252 |
0.2136 |
0.217 |
0.1738 |
0.1417 |
0.0962 |
7.5 |
0.097 |
0.185 |
0.179 |
0.156 |
0.147 |
0.08 |
0.075 |
12.5 |
0.1615 |
0.2 |
0.194 |
0.188 |
0.152 |
0.1002 |
0.1002 |
17.5 |
0.23 |
0.227 |
0.214 |
0.213 |
0.16 |
0.117 |
0.117 |
30 |
0.347 |
0.327 |
0.278 |
0.23 |
0.1775 |
0.115 |
0.115 |
RF % |
-ray energy(keV) |
||||||
185 |
240.7 |
294 |
305.78 |
608.7 |
1121 |
1767.6 |
|
0 |
0.207 |
0.203 |
0.2011 |
0.179 |
0.159 |
0.073 |
0.073 |
2 |
0.273 |
0.252 |
0.2136 |
0.217 |
0.1738 |
0.1417 |
0.0962 |
7.5 |
0.097 |
0.185 |
0.179 |
0.156 |
0.147 |
0.08 |
0.075 |
12.5 |
0.1615 |
0.2 |
0.194 |
0.188 |
0.152 |
0.1002 |
0.1002 |
17.5 |
0.23 |
0.227 |
0.214 |
0.213 |
0.16 |
0.117 |
0.117 |
30 |
0.347 |
0.327 |
0.278 |
0.23 |
0.1775 |
0.115 |
0.115 |
Fig. 4: Linear Attenuation Coefficient for samples with (0, 2, 17.5 and 30) % RF Table 4: HVL (mm) parameters for the prepared concrete/RF samples
Fig. 5 shows the linear attenuation coefficients of Concrete /RF by using MCNP5. It is observed that the linear attenuation of the cement with 17.5 % RF has higher values. The measured results are compared with calculated values of linear attenuation coefficient in table 3 for samples with different rock fiber ratios at different photon energies is presented in Table 5. It can be seen that the results of () show good agreement between experimental and calculated results for high energies. Moreover, there is a rearkable deviation between the results values of () for low energies.
Linear Attenuation Coeff. (Cm-1)
Linear Attenuation Coeff. (Cm-1)
0.30
0.25
0.20
0.15
0.10
0.05
0 % RF
2 % RF
17.5% RF
30 % RF
0 % RF
2 % RF
17.5% RF
30 % RF
0.0 0.2 0.4 0.6 0.8 1.0 1.2 1.4 1.6
photon energy (MeV)
Fig. 5: linear attenuation coefficent of cement /RF versus incident photon energy using MCNP5.
Table 5: Comparison between experimental and theoretical linear attenuation coefficient results.
energy (keV) |
0% RF |
2% RF |
17.5% RF |
30% RF |
||||
Exp. |
MCNP |
Exp. |
MCPN |
Exp. |
MCPN |
Exp. |
MCNP |
|
185 |
0.18 |
0.16015 |
0.273 |
0.16015 |
0.23 |
0.2732 |
0.347 |
0.18096 |
240.7 |
0.207 |
0.13578 |
0.252 |
0.13578 |
0.214 |
0.23197 |
0.227 |
0.15375 |
294 |
0.2011 |
0.12099 |
0.214 |
0.12099 |
0.227 |
0.20667 |
0.278 |
0.13699 |
350.78 |
0.179 |
0.11028 |
0.217 |
0.11028 |
0.213 |
0.1884 |
0.23 |
0.1249 |
608.7 |
0.159 |
0.10194 |
0.174 |
0.10194 |
0.16 |
0.17414 |
0.1775 |
0.11546 |
1121 |
0.073 |
0.07199 |
0.1417 |
0.13578 |
0.117 |
0.123 |
0.115 |
0.08154 |
1767.6 |
0.073 |
0.06563 |
0.0962 |
0.06563 |
0.117 |
0.11212 |
0.115 |
0.07434 |
For a better view of the comparison between XCOM theoretical results and experiment results values for the concrete reinforcement with Rock Fibers (0, 2, 17.5 and 30) % is plotted in Fig. 6 (a, b, c), respectively. It is clear that there is a high deviation between experimental and theoretically results for all energies. The important reason for this deviation is that the composite elements do not distribute uniformly. XCOM homogeneous mixture defined as any mixture part has the same proportions of its components and the mixture has only one phase. The attenuation coefficient for mixture is calculated by XCOM as the sum of the corresponding quantities for the atomic constituents (17). Specifically, fibers contains of many elements such as (Sio2, Fe2O3, MgO), therefore, this elements not distribute in the composite as it defined in the XCOM theory, and the photon rays path traveled in the material was changed due to the RF agglomerates. The RF tends to agglomerate as more is added, resulting deviation between measured and theoretical attenuation. Fig. 7 shows the transition factor of cement with different ratios of RF and different thickness of the samples. It is observed that the cement with 17.5 % RF is the best compound as shielding for gamma radiation. Also, a thickness of 4 cm of the proposed compound may reduce up to 2.75% from gamma intensity. Fig. 8 shows the Half value layer versus Incident Photon Energy for concrete / RF calculated by MCNP5. Its observed that the optimum value of HVL recorded by 17.5 % RF ratio for all energies.
0.24
Linear attenuation Cofficient Cm-1
Linear attenuation Cofficient Cm-1
0.22
0.20
0.18
0.16
0.14
EXP. XCOM
a
a
17.5 %
0.60
Linear Attenuation Coefficient (Cm-1)
Linear Attenuation Coefficient (Cm-1)
0.55
0.50
0.45
0.40
0.35
0.30
0.25
b exp.
XCOM
RF 2%
0.12
0.10
0.08
0.20
0.15
0.10
0.05
0.0 0.2 0.4 0.6 0.8 1.0 1.2 1.4 1.6
photon energy (MeV)
0.0 0.2 0.4 0.6 0.8 1.0 1.2 1.4 1.6
Photon energy (MeV)
Linear Attenuation Coefficient( Cm-1)
Linear Attenuation Coefficient( Cm-1)
0.35
0.30
c exp.
XCOM
RF 30%
0.25
0.20
0.15
0.10
0.05
0.0 0.2 0.4 0.6 0.8 1.0 1.2 1.4 1.6
Photon energy (MeV)
0% RF
2% RF
17.5% RF
30% RF
0% RF
2% RF
17.5% RF
30% RF
Fig. 6: Comparison between experimental and theoretical linear attenuation coefficient values for (a) 2% RF, (b) 17.5% RF, (c) 30% RF
0.75
transmission of gamma rays
transmission of gamma rays
0.70
0.65
0.60
0.55
0.50
0.45
0.40
0.35
0.30
0.25
0.20
0.15
2 3 4 5 6
Thickness(cm)
Fig. 7: the variation of transimation of cement /RF samples versus thickness at 0.185 MeV. using MCNP5
0% RF
2% RF
14 17.5% RF
30% RF
12
Half-Value Layer (HVL)
Half-Value Layer (HVL)
10
8
6
4
2
0
0.2 0.4 0.6 0.8 1.0 1.2 1.4
Photon energy (MeV)
Fig. 8: Half value layer versus Incident Photon Energy for concrete / RF using MCNP5
4. CONCLUSION
The effect of rock fiber on shielding properties of concrete matrices were studied. Concrete samples were prepared using different ratio of rock fiber with length 0.5 mm. Shielding properties were determined experimentally and theoretically used XCom program and Monte Carlo Simulations. The main conclusions of this study can be summarized:
-
The gamma shielding properties of concrete is significantly improved by reinforcement by Rock Fiber for different photon energies
-
the linear attenuation coefficient decreases with photon energy increasing,
-
A linear attenuation coefficients for concrete samples with (0, 2, 17.5 and 30) % RF recorded the highest linear attenuation values.
-
High value Layer decreases with increases of Rock Fiber content.
-
There is observed that deviation between experimental and theoretically results for low energies, this is due to Irregular distribution of fiber in concrete matrix.
-
Theoretically results are in good agreement with experimental results for the optimum ratios of RF (0, 2, 17.5 and 30)
% RF.
DECLARATION OF COMPETING INTEREST
The authors declare that they have no known competing financial intercessor personal relationships that could have appeared to influence the work reported in this paper.
REFERENCES
-
Hossain Md., RIPAN B., FAZLUL MD., ABDUS S. M. 2016. Calculation of Gamma-Ray Attenuation Parameters for Locally Developed Ilmenite- Magnetite Concrete. J. Bangladesh Acad. Sci., V. 40, 11-21.
-
Alam, M.N., Miah, M.M.H., Chowdhury, M.I., Kamal, M., Ghose, S., Rahman, Runi. 2001. Attenuation coefficients of soils and some building materials of Bangladesh in the energy range 2761332keV. Appl. Radiat. Isot. V. 54, 973976.
-
Akkurt, I., Akyldrm, H., 2012. Radiation transmission of concrete including pumice for 662, 1173 and 1332keV. gamma rays. Nucl. Eng. Des. V. 252, 163166.
-
Akkurt, I., Basyigit, C., Kilincarslan, S., Mavi, B., 2005. The shielding of -rays by concretes produced with barite. Prog. Nucl. Energy V. 46, 111.
-
El-Samrah, M.G., Abdel-Rahman, M.A.E., El Shazly, R.M. 2018. Effect of heating on physical, mechanical, and nulear radiation shielding properties of modified concrete mixes. Radiat. Phys. Chem. V. 153, 104110.
-
Raul F. F, 2016. Attenuation Properties of Cement Composites: Experimental Measurements and Monte Carlo Calculations. Missouri University of Science and Technology. https://scholarsmine.mst.edu/masters_theses/7550.
-
Duaa F., Nildem T., Abdullah A., 2018. The Investigation of Basalt and Glass Fibers on the Behavior of Reinforced Concrete Beams. Inter. J. of Adv. in Mech. and Civil Eng., V 5.
-
Naus D.J., 2007. Primer on Durability of Nuclear Power Plant Reinforced Concrete Structures – A Review of Pertinent Factors. Oak Ridge National Laboratory (NUREG/CR-6927).
-
Tassew S.T., Lubell A.S., 2014. Mechanical properties of glass fiber reinforced ceramic concrete. Construct. Build. Mater. V. 51, 215224.
-
Chandramouli K., Srinivasa R.P., Pannirselvam N., Seshadri S.T., Sravana P. 2010. Strength properties of glass fiber concrete, J. Eng. Appl. Sci. V 5, 16.
-
Nulk H., Ipbüker C., Gulik V., Biland A., Tkaczyk. A., 2014. The investigation of gamma and neutron shielding properties of concrete including basalt fiber for nuclear energy applications. Conference Paper.
-
Hao Z., Bin J., Hui H., Yanling M, 2020. Experimental Study on Basic Mechanical Properties of Basalt Fiber Reinforced Concrete. Material J. V. 13, 1362.
-
Hajar S. I., Amin A. 2020. Investigating the effect of basalt fiber additive on the performance of clay barriers for radioactive waste disposals. Bull. of Eng. Geol. and the Envy. J. DOI 10.1007/s10064-020-02044-x
-
Moharrama B.M., Nagyb M.E., Mohamed K. S., El Sayeda A.R., Mohammed F., Samy A. D., Mahmoud E. D., 2020. Performance of lead and iron oxides nanoparticle materials on shielding properties for -rays. Rad. Phys. and Chem. J., V.137. https://doi.org/10.1016/j.radphyschem.2020.108880
-
RSICC Computer Code Collection. MCNPX Users Manual. 2002. Version 2.4.0., Monte Carlo N-Particle Transport Code System for Multiple and High Energy Applications.
-
Nadin J. A., Mira N. A., Noorfatin A. B. A., Rafidah Z., 2020. Tungsten-based material as promising new lead-free gamma radiation shielding material in nuclear medicine. Physica Medica. V 78, 48-57
-
Yingwei H., Min L., Yizhuo G., Zhongjia Y., Ran L., Zuoguang Z. 2017. Gamma Ray Shielding Property of Tungsten Powder Modified Continuous Basalt Fiber Reinforced Epoxy Matrix Composites. Poly. Comp. DOI 10.1002/pc.24469