
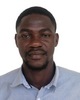
- Open Access
- Authors : Edmund Nana Asare, Richard Asiamah
- Paper ID : IJERTV11IS010124
- Volume & Issue : Volume 11, Issue 01 (January 2022)
- Published (First Online): 30-03-2022
- ISSN (Online) : 2278-0181
- Publisher Name : IJERT
- License:
This work is licensed under a Creative Commons Attribution 4.0 International License
Geotechnical Site Evaluation and Stability Analysis of a Tailings Dam, A Case Study
Edmund Nana Asare, Richard Asiamah Geological Engineering Department University of Mines and Technology, (UMaT), Tarkwa, Ghana
Abstract The safety of tailings dam is of great concern because of how catastrophic their failures can be. This project was conducted to ascertain the stability of a proposed tailings dam. The geotechnical properties of the designated construction site and the building material were tested for. A two- dimensional model of the proposed tailings dam was then developed with the use of GEO5 Geotechnical Software Suite. The geotechnical parameters obtained from the laboratory testing was inputted into the developed model. A Finite Element Method (FEM) of analysis within the GEO5 Geotechnical Software Suite was employed to perform a slope stability analysis, steady state seepage analysis as well as a consolidation analysis to assess the long-term stability of the tailings dam. From the analysis, the factor of safety of the dam was 1.88 which means the dam is safe. Steady stage seepage analysis revealed that the dam was safe from undergoing failure by hydraulic fracture or internal erosion. The consolidation analysis revealed a settlement value of 28 mm which means the foundation was strong enough to hold the tailings storage facility without undergoing settlement above the allowable range.
Keywords Tailings Dam, Stability Analysis, Finite Element Method, Dam Modelling, Site Evaluation
-
INTRODUCTION
Tailings dam, also referred to as tailings storage facility (TSF) is a very important engineering structure in the extractive companies. Large quantities of waste in the form of slurry are generated in the processing of ores to extract the main mineral of interest. These wastes generated from ore processing happens to be environmentally hazardous due to the reagent (chemicals) that are introduced to aid in the extraction. These wastes are therefore directed to and stored in the tailings dam. Globally, quite a number of catastrophic incidents of tailings storage facility failures have been recorded. A few include the TSF failure at Vales Feijo Iron Mine in Brumadinho, Brazil in January 2019, resulting in over three hundred (300) deaths. Another one is the Samarco TSF failure in Brazil, in the year 2015 which resulted in nineteen
(19) fatalities and then the tailings dam failure at Mt. Polley Mine in Canada in 2014 which caused significant environmental damage [5].
There is therefore the need to perform laboratory test to define geotechnical parameters of the building material in order to perform stability evaluation so as to safely design and construct these facilities to withstand the test of time. It is in light of this, the paper seeks to conduct engineering studies to assess the properties of the soil material and perform stability evaluation on embankments and foundation for the purpose of constructing a tailings dam using the finite element method (FEM). FEM is one of the most effective and applied
numerical methods in the simulation of the time-dependent, anisotropic and nonlinear behaviour in advance soil and rock mechanics [2].
-
GEOLOGICAL INFORMATION ABOUT STUDY AREA
Wassa Abreshia is a town in the Wassa Amenfi East Municipality of the Western Region, Ghana. The Project lies along the western flank of Ashanti Belt which is one of the NortheastSouthwestern (NE-SW) trending Birimian volcanic belts exposed in south western Ghana. Most of south-western Ghana is dominated by metamorphosed rocks of the Paleoproterozoic age, which fall mainly between the ages of 2300 and 1900 Ma [3]. The immediate concession area is underlined mainly by meta-sediments of the Paleoproterozoic Birimian flysch type consisting of dacitic volcaniclastics, greywackes plus argillaceous (phyllitic) sediments, intensely folded, faulted and metamorphosed to the upper green schist facies [9]. Numerous small Basin-type granite bodies are reported to have intruded the sediments along several regional structures. The shape of the intrusions varies from nearly ovoid plugs 200 m to 400 m long by 40 m to 150 m wide to relatively long (+ 2000 m) and narrow (50 m to100 m) sills or dykes [9]. Fig. 1 shows the geological map of the area.
Fig. 1 Geological Map of the Study Area
-
MATERIALS AND METHODS USED Careful and detailed field and laboratory tests were carried
out to determine the geotechnical properties of the material at the proposed tailings dam site. Geotechnical laboratory works carried on soil samples are herein in accordance with standard protocols for soil mechanics studies. The procedures and techniques employed in these studies conform to the
performance criteria (detection limits, accuracy and precision) as outlined in the [8] protocol for mechanical and index methods for assessing the properties of soils for engineering projects. The British Standard for the determination of geo- mechanical properties of soils for civil engineering projects were employed for the analysis. At all times laboratory procedures were optimised during analyses to assure the quality of data. Results relate only to the selected samples tested.
-
Field and Laboratory Works
Surface and subsurface conditions were explored by excavation and logging. In-situ test known as dynamic cone penetration test (DCPT) was also performed. Two samples each, a disturbed and an undisturbed, were also collected from five sampling locations between the depths of 25 cm to 300 cm and conveyed to the Geotechnical Laboratory for analysis. Eight laboratory tests were performed on each of the samples collected in order to evaluate the index and engineering properties that influence the performance of the
proposed tailings dam. These include the following: Natural Moisture Content (BS 1377-2:1990) Specific Gravity (BS 1377-2:1990)
Unit Weight (BS 1377-2:1990) Atterberg Limits Tests (BS 1377-2:1990)
Particle Size Distribution (BS 1377-2:1990) Standard Proctor Compaction (BS 1377- 4:1990)
Direct Shear Test (BS 1377- 7:1990)
Falling Head Permeability Test (BS 1377- 5:1990).
-
Modelling of the Proposed Tailings Dam Using Finite Element Method (FEM).
The finite element method is a versatile numerical method which was developed to solve problems related to the concentration of stress in continuum mechanics. This method also accounts for the heterogeneities of materials under study, irregular geometries, nonlinearities as well as complex boundary conditions [2]. It is the most applied numerical method in the simulation of the time dependent, anisotropic and nonlinear behaviour in advance soil and rock mechanics.
The guiding principle of this method is to divide the section of the problem into sub-sections which is termed finite element. These sub-sections are analysed individually before they are assembled to generate a global solution to the problem. The generation of the finite elements which can be addressed individually enable this method to account for heterogeneities and non-linear stress-strain behaviour as well as stress-pore pressure variations. GEO 5 FEM program allows for the modelling of various types of problems and analyses. These modelling and analyses are carried out in various stages. The first stage is the topology stage and then subsequent constructional stages [6].
In the topology stage, a two-dimensional (2D) model of the tailings dam and its foundation was developed. In order to find a balance between cost and safety, an angle of 26.56 ° was used for both upstream and downtream slopes in developing the model. An embankment height of 5 m was considered for the modelling of the dam. The geotechnical parameters of the soils were assigned to the various soil interfaces in the model. This was then followed by a generation of the finite element mesh.
Fig. 2 A Generated Mesh of Tailings Dam Model
-
-
ANALYSIS AND DISCUSSION OF RESULTS
-
Dynamic Cone Penetration Results
Results from the dynamic cone penetration test is presented in the graph of depth of penetration against bearing capacities in Graph 1.
Graph-1 Depth against Bearing Capacity
A general rule of thumb is that bearing capacity generally increases with increasing depth. From the Fig. 4 however, the plot of bearing capacities against depth is not entirely continuous. This depicts heterogeneities within the soil layers in the subsurface. Averagely, the deviation from a regular downward increment in depth and bearing capacity is minimal and therefore there is no need for deep excavations and reinforcements for the tailings dam foundation.
-
Logging of Excavated Sample Pits
Results from the logging of the sample pits are described individually in Table I and the general profile is presented Fig. 3.
DEPTH (m)
DESCRIPTION
TP 01
TP 02
0.00 0.25
Light brown to dark brown, moderately loose to firm, silt to fine sand (topsoil).
Light brown to dark brown, moderately loose to firm, silt to fine sand (topsoil).
0.25 3.08
Pale yellow colour. Wet, moderately loose silty clay soil (subsoil). Groundwater was encountered at the depth of 2 m approximately.
Pale yellow colour. Wet, moderately loose siltyclay soil (subsoil). Groundwater was encountered at the depth of 2.2 m approximately.
TABLE I. PROFILE OF THE SUBSURFACE LAYERS OF THE AREA
3.08
Grey to white firm transitional material. Mostly phyllite.
Grey to white firm transitional material. Mostly phyllite.
DEPTH (m)
DESCRIPTION
TP 03
TP 04
0.00 0.23
Light brown to dark brown, moderately loose to firm clayey silty fine sand (topsoil).
Light brown to dark brown, moderately loose to firm clayey silty fine sand (topsoil).
0.23 3.00
Pale yellow colour. Wet, moderately loose silty clay soil (subsoil). Groundwater was encountered at the depth of 2 m approximately.
Pale yellow colour. Wet, moderately loose siltyclay soil (subsoil). Groundwater was encountered at the depth of 2.1 m approximately.
DEPTH (m)
DESCRIPTION
TP 05
0.00 0.25
Light brown to dark brown, moderately loose to firm clayey silty fine sand (topsoil).
0.25 3.00
Pale yellow colour. Wet, moderately loose clayey silty fine sand (subsoil). Groundwater limit at 3 m.
TABLE II. SUMMARY OF SPECIFIC GRAVITY, MOISTURE CONTENT AND FALLING HEAD PERMEABILITY TEST RESULTS
Sample ID
Depth(m)
Moisture Content (%)
Specific Gravity
Falling Head (m/day)
TP 01
0.25 3.08
26.59
2.54
0.02497
TP 02
0.25 3.08
25.89
2.53
0.02732
TP 03
0.23 3.00
23.58
2.52
0.02759
TP 04
0.23 3.00
27.40
2.54
0.02293
TP 05
0.25 3.00
22.53
2.52
0.02759
From the logging of the 300 cm deep excavation of the sampling points, two main layers were observed. The first observed layer of a 25 cm thickness was identified as the top soil. This layer was black to brown colour (humus) which was mostly sandy to silty grain loose material with moderate moisture content. This layer needs to be stripped off before beginning the project. The second layer which happens to be the subsoil lies between 25 cm to 300 cm depth. This layer was made up of a pale yellow phyllitic soil which is silty in nature. Groundwater was encountered at an approximate depth of 200 cm. Beneath the loose phyllitic sub soil was a grey to white coloured transitional material. This layer is believed to be phyllite (parent material). This layer was found at an average depth 300 cm.
Fig.3 Profile of the Sub Surface layers of the Area
-
Moisture Content, Specific Gravity and Falling Head Permeability Test
The summary of results obtained from the moisture content, Specific gravity and falling head permeability test are presented below in Table II. It can be observed that the soil has a normal specific gravity of natural soils, moderate permeability rate which is characteristic of sandy silt soils. Water content is low which means the soil properties are not going to change much with water. Hence the soil is not problematic.
-
Atterberg Limits Test
From Atterberg Limits results in Table III it can be established that the soil in the study area has an intermediate to low plasticity and this is as the result of small quantities of clay within the soil. This means the soil has low expansive nature.
TABLE III. SUMMARY OF RESULTS OBTAINED FROM ATTERBERG TESTS
Sample ID
Depth(m)
Atterberg Limit
LL (%)
PL (%)
PI (%)
TP 01
0.253.08
37.00
25.90
11.10
TP 02
0.253.08
37.00
26.50
10.50
TP 03
0.23 3.0
38.00
29.80
8.20
TP 04
0.23 3.0
37.00
28.10
8.90
TP 05
0.25 3.0
37.00
26.30
10.70
-
Particle Distribution Analysis
From the particle size distribution curve plot for the various samples, the soils from TP 01, TP 02, TP 04 show a well graded fine soil. TP 03, TP 05 also showed a well graded coarse soil. From the percentages of the various particle sizes, the soil sample can be referred to as a sandy silt according to the British Standard Soil Classification System. The relative percentages of clay, silt, sand and gravel are presented in Table IV.
TABLE IV. PERCENTAGES OF EACH SOIL TYPE BASED ON PARTICLE SIZES
Sample ID
Depth(m)
Particle Size Distribution
Clay (%)
Silt (%)
Sand (%)
Gravel (%)
TP 01
0.25 3.08
6.00
47.60
41.10
5.40
TP 02
0.25 3.08
3.80
48.50
44.50
3.00
TP 03
0.23 3.00
4.10
23.46
37.00
35.44
TP 04
0.23 3.00
4.90
44.00
42.60
8.60
TP 05
0.25 3.00
4.40
15.20
39.60
40.80
-
Standard Proctor Compaction Test Results
From the compaction results in Table V, the soil was found to be of an average Unit Weight () of 16.91 kN/m3. An average Maximum Dry Density (MDD) of 1.795 g/cm3 can be attained at an Optimum Moisture Content (OMC) of 13.6 %.
Sample ID
Depth (m)
Compaction Test Results
Unit weight
MDD (g/cm3)
OMC (%)
, (kN/m3)
TP 01
0.25 3.08
1.796
12.00
16.78
TP 02
0.25 3.08
1.794
14.00
17.01
TABLE V. SUMMARY OF COMPACTION TEST RESULTS AND UNIT WEIGHT
TP 03
0.23 3.00
1.791
14.40
16.88
TP 04
0.23 3.00
1.795
13.00
16.98
TP 05
0.25 3.00
1.797
14.60
16.92
-
Result of Direct Shear Strength Test
Direct shear test revealed that the of the soils in the area had average shear strength parameters of 11.98 kN/m2 for cohesive force and 23.89 ° for internal frictional angle. This suggest that the soil has a moderate to high shear strength.
TABLE VI. SUMMARY OF DIRECT SHEAR STRENGTH TEST PARAMETERS
Sample ID
Depth (m)
Direct Shear Test
Cohesion, C (kN/m2)
Frictional Angle, (°)
TP 01
0.25 3.08
11.98
23.60
TP 02
0.25 3.08
11.97
24.01
TP 03
0.23 3.00
11.97
24.18
TP 04
0.23 3.00
11.99
23.75
TP 05
0.25 3.00
11.98
23.90
-
Modelling and Analysis of Tailings Dam
The finite element method analysis tool within the GEO5 Software Suite was used in the analysis. Analysis of the tailings dam model was done using the Mohr-Coulomb approach. The embankment was analysed completely and homogeneously using the result from the laboratory tests. Table VII shows a summary of the soil parameters that were used in the analysis.
TABLE VII. SUMMARY OF SOIL PARAMETERS FOR THE ANALYSIS
Parameters
Notation
Value
Unit
Material model
Model
Mohr – Coulomb
Unit weight
16.91
kN/m3
Poisson's ratio
0.40
Elastic modulus
E
10.00
MPa
Modulus unloading / reloading
Eur
15.00
MPa
Angle of internal friction
23.89
°
Cohesion of soil
c
11.98
kN/m2
Dilation angle
0.00
°
Saturated unit weight
sat
18.96
kN/m3
Permeability (both x, and y- direction)
Ksat
0.0257
m/day
Slope Stability Analysis
The stability analysis result is shown in Fig.5. The factor of safety was found to be 1.88. The failure surface in the resultant displacement mode is seen to be towards the upstream of the dam. Since the factor of safety is 1.88 which is higher than the accepted value of 1.5[1], it can be considered as safe.
Fig. 4 Slope Stability Analysis of Tailing Dam
Steady State Seepage Analysis
Another factor that normally cause dam failure is hydraulic fracturing. This occurs when the phreatic line within the embankment daylight into the downstream side of the dam. From the steady state seepage analysis in Fig. 5, the phreatic line within the embankment seems to reduce gradually and water flow out through the existing groundwater table after long period of time. The tailings dam therefore can be considered safe from failing through hydraulic fracture.
Fig. 5 Steady State Seepage Analysis of The Tailing Dam
Consolidation
Consolidation or settlement is also an important factor during the construction huge structures. Soils with high settlement value may end up causing instability of structures built on them. From the consolidation analysis considering a period of 10 years, the maximum settlement value was 23.8 mm as shown in Fig. 6 below. Upon the increment of the number of years, no further increase in settlement value was noticed. The value of 23.8 mm is considered to be within an acceptable settlement range. The bearing capacity of the foundation soil can therefore be considered high enough to withstand the weight to be imposed by the tailings dam.
Fig. 6 Consolidation Analysis of the Tailing Dam
-
-
CONCLUSION AND RECOMMENDATION
-
Conclusion
From the research results, it can be concluded that the soil had a good shear strength. Geotechnical logging to a 300 cm deep excavation revealed that the site is heterogeneous. The soil material was a sandy silt type with an intermediate plasticity and an average permeability of 0.0257 m/day. Slope stability analysis gave a factor of safety of 1.88 which indicates that the soil is safe to be used for the construction of the tailings dam according to standard protocols. Consolidation analysis showed that the foundation is strong enough to hold the dam while undergoing minimal settlement which is within the allowable range. Steady state seepage analysis indicated that the tailings dam can resist failure through internal erosion and piping. The tailings dam project would be safe to be constructed on the designated site.
-
Recommendation
Plastic liners such as high-density polyethylene (HDPE) should be used to line the upstream slope face. This will prevent any future problems related to seepage in the case of further tailings dam raise because of the uncertainty in geotechnical properties of the soils that will available for the future raise.
A three-dimensional (3D) slope stability analysis of the tailings dam should be performed to complement the two- dimensional (2D) analysis which generally provide
conservative results. With higher factor of safety expected in 3D analysis, a more cost-effective slope may be designed while maintaining adequate stability.
ACKNOWLEDGMENT
I would like to express my gratitude to my Supervisor Edmund Nana Asare who gave me the opportunity, guidance, and funding to do this research which introduced me to a lot of new knowledge. I am really thankful to him.
REFERENCES
[1] Dunn, I. S., Anderson, L. R., and Kiefer, F. W. (1980), Fundamentals of geotechnical analysis, John Wiley and Sons, New York. 414pp. [2] Nikoli, M., Roje-Bonacci, T., and Ibrahimbegovi, A. (2016), Overview of the numerical methods for the modelling of rock mechanics problems, Tehniki Vjesnik, Vol. 23,No. 2, pp. 629 630. [3] Allen, G. D. (2011), Technical report on the fahiakoba gold project, Accessed: March 03, 2021. [4] Ghana Statistical Service (2014), Wassa Amenfi East district analytical report, www.statsghana.gov.gh. Accessed: March 12, 2021. [5] Goldfields Ghana (2019), Integrated annual report 2018, www.goldfields.com/reports/annual-report-2018/waste-and- tailings.php. [6] Geo5 Engineering Manual, www.finesoftware.eu/engineering- manuals/. [7] Armah, E. (2013), Impact of small-scale gold mining on some water parameters of River Ashire, Unpublished MSc Thesis Report, Kwame Nkrumah University of Science and Technology, Kumasi, 91pp. [8] British Standards Institution BS 1377, (1990), Methods of test for soils for civil engineering purposes, BSI, London. [9] Payne, P., Marwood, B. and Price, D., (2009), Technical Report, Central Ashanti Gold Project, Ghana, for Perseus Mining Limited