
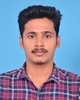
- Open Access
- Authors : Jishnu M V , Dr. Rajesh K N
- Paper ID : IJERTV10IS020206
- Volume & Issue : Volume 10, Issue 02 (February 2021)
- Published (First Online): 01-03-2021
- ISSN (Online) : 2278-0181
- Publisher Name : IJERT
- License:
This work is licensed under a Creative Commons Attribution 4.0 International License
Hollow Core FRP- Concrete- Steel Column with Inner Square Steel Tube Subjected to Eccentric Loading
Jishnu M V
Dept. of Civil Engineering Govt. College of Engineering Kannur
Kannur, India
Dr. Rajesh K N
Dept. of Civil Engineering Govt. College of Engineering Kannur
Kannur, India
Abstract Hollow Core FRP- Concrete- Steel (HCFCS) columns are an advancement over hollow core columns, in which concrete is confined between an outer FRP tube and an inner steel tube. They consists of different types of materials and hence, combines and make advantage of all these materials. Steel tube in these columns itself act as a formwork and also as longitudinal and shear reinforcement. The concrete sandwiched between FRP tube at outside and steel tube inside is effectively confined. Outer FRP and inner steel tube offer continuous confinement to the concrete core, thus improving the strength and ductility of column. They are mainly used as bridge columns in seismic areas. This study is concerned with the behavior of Hollow Core FRP- Concrete- Steel columns with inner square steel tube subjected to eccentric loading. Finite Element study was done in Ansys Workbench. Various parameters were examined including the thickness of steel tube, type of fiber and the shape of column section. HCFCS column with inner square steel tube has shown a larger ultimate load compared to the column with inner circular steel tube.
KeywordsHollow core, FRP, Concrete, Steel
-
INTRODUCTION
Different types of column construction are followed and practiced from years. Composite columns were introduced years ago, that consists of two or more types of materials, usually concrete and steel. Concrete Filled Steel Tubular (CFST) columns consisted of steel hollow sections filled with plain or reinforced concrete. Then, Hollow Core columns were introduced with the idea of reducing the self-weight of the structure. Double skin tubular column was such a type, with the concrete confined within two concentric steel tubes. Despite the various advantages, one of the limitation with such a construction was the corrosive nature of steel tubes, which affect the performance of the column adversely. In this scenario, an advancement over such columns is presented by Hollow Core FRP- Concrete- Steel (HCFCS) Columns. HCFCS columns incorporates FRP tube and steel tube respectively at outside and inside, with concrete filled in between them. They are mainly used as tall piers in bridge construction. Being a hollow structural member, they have low self weight, which make constructions easy and reduce freight costs when used in precast constructions. They make use of advantages of various kinds of materials: concrete, steel and FRP. The outer FRP tube, being corrosion resistant, eliminate the disadvantage of double skin tubular members. The concrete is effectively confined by FRP and steel tube, which by itself function as a formwork for construction.
Several research works were conducted to evaluate the behaviour of HCFCS columns subjected to axial compression, flexural, seismic and impact loads. Under axial compression, failure of HCFCS column was due to rupture of FRP tube [1]. The concrete in HCFCS column was efficiently constricted by both steel and FRP tube and the behaviour of confined concrete was same as that of concrete in solid columns confined only by FRP. Failure of all tested specimens occurred because of the rupture of FRP tubes, generally at the position where deformation of steel tube was found [2]. Steel tube at the interior of the column failed by elephant foot buckling.
The concrete in an HCFCS column has shown highly ductile compressive behaviour due to effective confinement. The ultimate axial stress and strain of concrete was found to increase with larger diameter of inner steel tube [3].
The performance of HCFCS column with expansive consolidating concrete and self-consolidating concrete was studied. The number of FRP layers were found to influence the prestressing. It is also affected by the diameter of steel tube [5]. A reduction of prestress in concrete was observed with the increase in diameter of the steel tube.
Seismic performance of HCFCS column was studied by many researchers. Bending strength was found to increase with increasing axial load, concrete compressive strength and the number of FRP layers, while with the increase in concrete wall thickness and the diameter-to-thickness ratio of the steel tube, it reduced [7]. A non- linear flexural analysis of column was also done and the bending strength was calculated following analytical procedure. Comparison of the experimental and analytical results was done and found overestimated strength results in analytical procedure.
Very low lateral displacement was observed for columns with larger steel tube. When concrete is filled inside the hollow steel tube, HCFCS column has shown significant increase in the lateral deformation capacity. In addition to that, when larger steel tube is used, higher lateral deformation is observed than that seen in a column with smaller steel tube [10].
Improved flexural capacity was observed for HCFCS column [12]. The flexural strength of column was found to increase with the confinement, strength of concrete and the applied load. Outward local buckling of the steel tube was delayed because of the presence of concrete filled between FRP and steel tube [1, 12].
Under lateral impact loading also, the HCFCS column behave in a ductile manner [13, 14]. With increase in the
number of FRP layers, the lateral deformation of column decreased.
-
VALIDATION
-
Description of experimental model
The results of experiment conducted by researchers [5] on hollow core FCS columns under axial loading condition was validated. 20 Hollow core FCS column with both inner and outer circular cross section were cast and applied with axial compressive load. The outer diameter and inner diameter of the column were 150.334 mm and 52 mm respectively. The height
To study the behaviour of column with square steel tube, a new HCFCS column with inner square steel tube is modelled. The steel tube dimension is selected so that the area of cross section is same as that in circular section. So, in that case, the calculated value of steel tube dimension is 53.2 mm and thickness of 3.5 mm. Under axial loading, the column has shown an increase of 5.09% in the ultimate load compared to column with circular steel tube.
1400
1200
Load (kN)
Load (kN)
1000
of the column was 300 mm. FRP tube of 0.334 mm thickness and steel tube of 4 mm thickness were used. The cross sectional details of the specimen (Qui Cao et.al, 2018) is shown in Fig. 3.
-
Finite element model
The validation of hollow core column is done using ANSYS Workbench 16.1. The cross sectional dimensions as obtained from the study of Cao et.al is used in the model. The column
800
600
400
200
0
CIRCLE
SQUARE
0 0.5 1 1.5 2 2.5 3 3.5 4
Deformation (mm)
Fig. 1. Load- Deformation curve
specimen was modelled with the help of design modeller in ANSYS Workbench. Youngs modulus and Poissons ratio of steel tube were 200 GPa and 0.3 and that of concrete were 28284.27 MPa and 0.15 respectively. Yield and ultimate strength is 394 MPa and 565.2 MPa for steel. Concrete ultimate strength is given as 26 MPa. Carbon FRP tube is used in the study having an elastic modulus of 224 GPa and Poissons ratio of 0.33. The yield strength and ultimate tensile strength of the same were 372 MPa and 3400 MParespectively. A resin was used having Youngs modulus of 2620 MPa and ultimate tensile strength equal to 20 MPa.
The concrete wall and steel tube were modelled using SOLID 186 element. It is a higher order 3D twenty-node solid element that exhibit quadratic displacement behaviour, with three degree of freedom per each node: translations in the x, y and z directions. Since the thickness is less, FRP tube and epoxy are modelled by SHELL 181 element. It is well suited for analyzing thin elements. Shell 181 is a four-node element with six degree of freedom at each node: translations in x, y and z directions and rotations about the x, y and z- axes. The interface between concrete shell and FRP tube, and between concrete and steel tube were provided as Bonded. To simulate the experimental support condition of cantilever column, displacement and rotation in all directions were prevented at the
Thus, HCFCS columns with inner square steel tube shows a better performance over that with circular steel tube in terms of its ultimate load. Moreover, inner square steel tube is helpful in connecting column to the corresponding superstructures like girder and footing. Thus, square steel tube is a better option.
B. Eccentric loading
For the parametric study, a new HCFCS column with inner square steel tube is modelled with steel tube of size 60 mm. First, the column is loaded axially and analysed. Then, eccentric loading is applied in x- direction. Load is applied as displacement on top of the column at different eccentricities in x-direction. Minimum possible theoretical eccentricity is calculated as 20 mm. The model is analysed for eccentricity of 20 mm, 25 mm and 30 mm. When load is applied at some eccentricity with respect to an axis, it is found that the column fails at a smaller load as compared to the load value at axial loading condition. When loaded at an eccentricity, the column is subjected to a uniaxial moment in addition to the axial load. This may lead to a reduced load bearing capacity for columns. Also, the ultimate load capacity goes on decreasing with increase in eccentricity value.
1400
bottom face of the column. Load is applied as displacement at the top face of the model. The ultimate load value as obtained from the numerical study was compared with the experimental results of researchers [5]. Comparison of the results is given in Table 1.
Ultimate load (kN)
Experimental
1177
FE Analysis
1148.2
% Error
2.45
Ultimate load (kN)
Experimental
1177
FE Analysis
1148.2
% Error
2.45
TABLE I. COMPARISON OF RESULTS
1200
Load (kN)
Load (kN)
1000
800
600
400
200
0
e: 0
e: 20
mm e: 25
mm
e: 30
mm
The ultimate load of the column in experiment and that obtained by numerical analysis differ by a small value of 2.45%. Thus, the model created in the study is validated using the experimental study of Cao et.al, 2018 [5].
-
-
PARAMETRIC STUDY
A. HCFCS column with inner square steel tube
0 1 2 3 4
Deformation (mm)
Fig. 2. Load- Deformation curve for different eccentricity
-
Effect of steel tube B/ts ratio
Thickness (ts) of steel tube is varied while keeping the width
(B) same. Here, width- to- thickness ratio is taken as the parameter for study. So, the model is analysed under different B/ts ratio of 10, 12, 15 and 20 corresponding to thickness of 6
mm, 5 mm, 4mm and 3 mm at an eccentricity of 20 mm. Width of steel tube was 60 mm. There is an increase in ultimate load
Fig. 3. Cross section details of HCFCS column
available to take the load and hence, more load carrying capacity. The loaded side of the steel tube is stressed maximum with the top and bottom portion of steel tube showing smaller value of stress.
800
700
600
300
Load (kN)
Load (kN)
200
100
0
CFRP
with the increment in thickness of steel tube. As the width to
700
thickness ratio (B/ts) of steel tube is changed from 10 to 20, the
600
ultimate load decreased by 16.41% from 698.2 to 583.6 kN.
500
With the increase in thickness, more area of steel tube is
400
with the increment in thickness of steel tube. As the width to
700
thickness ratio (B/ts) of steel tube is changed from 10 to 20, the
600
ultimate load decreased by 16.41% from 698.2 to 583.6 kN.
500
With the increase in thickness, more area of steel tube is
400
ARAMID FRP
GLASS
FRP
0 0.5 1 1.5 2 2.5 3 3.5 4
Deformation (mm)
Fig. 5. Load- Deformation curve for different fibers
Load (kN)
Load (kN)
500
400
300
200
B/t: 20
B/t: 15
B/t: 12
The analysis has shown a large load carrying capacity for column with aramid fiber compared to that with glass fiber, while both being lower than column with carbon fiber. Compared to column with Glass fiber tube, ultimate load of
100 B/t: 10
0
0 1 2 3 4
Deformation (mm)
Fig. 4. Load- Deformation curve for different B/t values
-
Effect of various type of fibers
The performance of column with FRP tubes of various kinds of fibers were analysed. The validated model has an FRP thickness of 0.334 mm. Thus, columns were modelled with FRP tube of same thickness, made of two different types of fibers: Aramid and Glass fibers. Type of fiber significantly affect the load carrying power of HCFCS columns. Aramid FRP has a tensile strength of 2663 MPa and poisson ratio of
0.25. Modulus of elasticity is 125.7 GPa. For the glass fiber, modulus of elasticity is 46 GPa, poissons ratio is 0.18 and the ultimate tensile strength is taken as 1200 MPa.
column with Aramid fiber has an increased value of 4.53%. This significant increase in load value is due to better material properties of aramid fiber compared to glass fiber. It is very much evident from the tensile strength and elastic modulus value of different fibers.
-
Cross sectional shape
To study how the outer shape of the column affect the behaviour of HCFCS column, two models were created having rectangular and elliptical cross sections. The dimensions in each models were selected so that the cross sectional area of each three materials remain the same as that of the validated model. Thus, for square column, outer dimension is 132.9 mm, with all other dimensions remaining the same. For the elliptical section, the major axis and minor axis were 90 m and 62.5 mm respectively. The square column has gained more load carrying capacity. i.e., 8.24% more than circular column. The ultimate load and the load- deformation curve were comparable for both circle and elliptical column section, with the former showing 1.33% higher value of ultimate load.
800
700
600
Load (kN)
Load (kN)
500 CIRCULAR
normal-and high-strength concrete." Journal of Composites for Construction 18.1, ASCE 04013027, 2013
-
Zhou, Yingwu and Dhawang Li (2017) "Behaviour and modelling of FRPconcrete-steel double-skin tubular columns made of full lightweight aggregate concrete." Construction and Building Materials 139, ELSEVIER, 52-63, 2017
400
300
200
100
0
COLUMN
ELLISE
SQUARE
0 1 2 3 4
Deformation (mm)
-
Cao Qi, Jinju Tao, Zhimin Wu and John Ma (2018) "Behaviour of FRPsteel confined concrete tubular columns made of expansive selfconsolidating concrete under axial compression." Journal of Composites for Construction, ASCE, 04017037, 2018
-
Togay Ozbakkaloglu and Yunita Idris (2014) Seismic Behaviour of FRPHigh Strength Concrete- Steel Double Skin Tubular Columns.
Journal of Structural Engineering, ASCE, 04014019, 2014
-
Omar I Abdelkarim and Mohamed A. ElGawady (2014) "Analytical and finite-element modeling of FRP-concrete-steel double-skin tubular
Fig. 6. Load- Deformation curve for different shapes of column
-
-
CONCLUSION
This study presents FE model and parametric studies for HCFCS column under eccentric loading. A numerical model is validated against the experimental results of Cao et al, 2018 and the below conclusions can be made from the study:
-
HCFCS column with inner square steel tube has much higher load carrying capacity as compared to that with inner circular steel tube.
-
HCFCS column shows excellent performance in terms of load carrying capacity and deformation under eccentric loading.
-
Different fibers have significant effect on the performance of column. The load carrying capacity and deformation ability of column increases with the thickness of steel tube.
-
A square HCFCS column with inner square steel tube and made of Carbon FRP tube show better performance as a column.
REFERENCES
-
Yu Tao and J. G. Teng, "Behaviour of hybrid FRP-concrete-steel double- skin tubular columns with a square outer tube and a circular inner tube subjected to axial compression." Journal of composites for construction 17.2, ASCE, 271-279, 2012
-
Fanggi, Butje Alfonsius Louk, and Togay Ozbakkaloglu, "Compressive behaviour of aramid FRPHSCsteel double-skin tubular columns."
Construction and Building Materials 48, ELSEVIER, 554-565, 2013
-
Ozbakkaloglu Togay and Butje Louk Fanggi, "Axial compressive behaviour of FRP-concrete-steel double-skin tubular columns made of
columns." Journal of Bridge Engineering 20.8, ASCE, B4014005, 2014
-
Omar I Abdelkarim and Mohamed A ElGawady (2016) Behaviour of hollow FRPconcretesteel columns under static cyclic axial compressive loading." Engineering Structures 123, ELSEVIER, 77-88, 2016
-
Abdelkarim, Omar I Mohamed A. ElGawady, Ahmed Gheni, Sujith Anumolu and Mohanad Abdulazeez (2016) "Seismic performance of 46 innovative hollow-core FRPconcretesteel bridge columns." Journal of Bridge Engineering 22.2, ASCE 04016120, 2016
-
Idris Yunita and Togay Ozbakkaloglu (2016) "Behaviour of square fiber reinforced polymerhigh-strength concretesteel double-skin tubular columns under combined axial compression and reversed-cyclic lateral loading." Engineering Structures 118, ELSEVIER, 307-319, 2016
-
Omar I Abdelkarim, Mohamed A. ElGawady, Sujith Anumolu, Ahmed Gheni and Gregory E. Sanders (2017) "Behaviour of hollow-core FRPconcrete-steel columns under static cyclic flexural loading." Journal of Structural Engineering 144.2, ASCE, 04017188, 2017
-
Mohanad M. Abdulazeez, Mohamed A. ElGawady and Omar I. Abdelkarim (2019) Bending and Buckling Behaviour of Hollow-Core FRPConcreteSteel Columns, Journal of Bridge Engineering, ASCE, 04019082, 2019
-
Wang, Rui, Lin-Hai Han and Zhong Tao (2015) "Behaviour of FRP concretesteel double skin tubular members under lateral impact: experimental study." Thin-Walled Structures, ELSEVIER, 363-373, 2015
-
Abdelkarim, Omar I. and Mohamed A. ElGawady (2016) "Performance of hollow-core FRPconcretesteel bridge columns subjected to vehicle collision." Engineering Structures, ELSEVIER, 517-531, 2016
-
T. Yu, J. G. Teng and Y. L. Wong (2010) Stress-Strain Behaviour of Concrete in Hybrid FRP-Concrete-Steel Double-Skin Tubular Columns. Journal Of Structural Engineering, ASCE, 136(4): 379-389, 2010
-
J.G. Teng, T. Yu, Y.L. Wong and S.L. Dong (2006) Hybrid FRP concretesteel tubular columns: Concept and behaviour. Construction and Building Materials, ELSEVIER, 846854.