
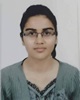
- Open Access
- Authors : Kabita Poudel , Keshav Basnet , Bikash Sherchan
- Paper ID : IJERTV10IS080220
- Volume & Issue : Volume 10, Issue 08 (August 2021)
- Published (First Online): 06-09-2021
- ISSN (Online) : 2278-0181
- Publisher Name : IJERT
- License:
This work is licensed under a Creative Commons Attribution 4.0 International License
Hydrological and Hydraulic Modeling for Flood Analysis: A Case Study for Modi Catchment
Kabita Poudel |
Keshav Basnet |
Department of Civil Engineering |
Department of Civil Engineering |
Pashchimanchal Campus, Institute of Engineering |
Pashchimanchal Campus, Institute of Engineering |
Tribhuvan University, Nepal |
Tribhuvan University, Nepal |
Bikash Sherchan
Department of Geomatics Engineering Pashchimanchal Campus, Institute of Engineering Tribhuvan University, Nepal
Abstract With the development of floodplains and climate change, the risks associated with flooding have increased, leading to increased flooding in floodplains. According to data from the Department of Hydrology and Meteorology, Nepal ranks 20th among countries in the world in the number of people affected by floods each year. Assessing the extent and depth of flooding has always been one of the main goals of water resources managers. This research aims to develop the Hydrological Engineering Center Hydrological Modeling System (HEC-HMS) and Hydrological Engineering Center River Analysis System (HEC- RAS) models for the Modi River Basin in Nepal. HEC-HMS is one of the hydrological models used to simulate the process of runoff and rainfall in different geographical areas. In this study, the precipitation data of seven meteorological stations and flow data of the hydrological station within the Modi River basin were used. The calibration was performed using data from 2006 to 2010 and was consequently validated using data from 2011 to 2013 at a daily time step. The efficiency of model was evaluated based on calculated statistical parameters (NSE, R2) and comparing the simulated and observed discharge flows. The performance of this model during calibration was very good, with the coefficient of determination R2 = 0.81, Nash-Sutcliffe Efficiency NSE = 0.78. Similarly, the performance of the model during the validation period was also very good, R2 = 0.80, NSE = 0.78. Based on these performance results, the HEC-HMS model made a satisfactory prediction of water flow in the Modi Basin. In addition, the flood map produced by HEC-RAS was used to study the flood range and its characteristics. The Modi River basin is dominated by forest lands and hence the flood plain mapping showed the inundation of forest and trees at the lower reach of basin. It indicated that the future settlement at the vicinity of river has been found to be at high risk of flood. The study also showed that the structure of hydropower station also lies in flood hazardous zone. The model can be used as a reference for water resources management and flood control in the Modi River Basin, and can also be applied to other river basins.
Keywords Flood, Flood Management, Hydropower, Inundation, Modi Basin, River Flooding
-
INTRODUCTION
-
Background
Nepal is made up of many small rivers that flow from north to south. The Koshi River, Narayani River and Karnali River are
the three main river systems in Nepal. These are the perennial rivers. They originate from the Himalayas and after descending from the hills, flow through the Terai plains. Nepal's huge hydropower potential is due to these perennial rivers. However, the changing climate pattern is resulting in extreme rainfall during monsoon and shortage of water for the remaining periods. Also, the hydro morphology of the river is severely altered. Overall, floods and landslides make up almost 75 percent of disasters in Nepal (Parajuli et al., 2017). Floods are caused by rainfall and storms, glacial lake overflow floods (GLOF), dam floods (LDOF), floods caused by infrastructure failures, sheet floods, or floods in lowland areas caused by imposing barriers to flow.
The coverage of the hydro-meteorological station network throughout Nepal is poor, and the country is severely lacking in time series of flow and rainfall data. The Modi River is a perennial snow-covered river and is the main tributary of the Kaligandaki River in the Gandaki Basin. The bed slope is steep in these mountainous rivers where water flows rapidly and has a high potential for hydropower generation. The natural factors as deposition of sediments and anthropogenic cause like encroachment of floodplains, obstruction of the natural flow of rivers, river training works, induces flood-related risk. Therefore, the simulation and prediction of rainfall and related runoff is essential for planners, decision makers and flood risk management. In addition, design flood estimation is essential for hydraulic structure design, flood management, insurance research, development, and planning.
This research used Hydrological Modeling System (HEC- HMS) and the Center for Hydrological Engineering-River Analysis System (HEC-RAS) to develop a physical model that simulates rainfall-runoff events. HEC-HMS deals with the basic water balance equation, which takes into account the main parameters that control runoff and can simulate rain runoff events, while HEC-RAS can simulate runoff hydraulics through the channel according to the channel morphology, and can generate the range of inundated zone. The HEC-HMS and HEC-RAS models can assess areas flooded with known storms. Additionally, the calibrated model can be used for future
floodplain mapping, future rainfall data and land use scenarios, and sustainable management of water resources.
-
Study Area
The Modi River is one of the main tributaries of the Kaligandaki River in Gandaki Province (Fig.1). The river begins in the glaciers of the Annapurna Mountains in the Kaski District and finally merges into the Kaligandaki River at Modibeni in Parbat District. The main stream of Modi River is about 50 kilometers long and its source is about 755 meters above sea level. The upper reaches of the river are located in the Annapurna Conservation Area, which is famous for its tourism industry, while the downstream has huge potential for hydroelectric power generation.
The study area is located in Parbat, Kaski and Myagdi districts. The river passes through two Rural municipalities (Annapurna and Machhapucchre) of the Kaski district and Kusma municipality, Modi Rural municipality, Falewas Rural municipality, of Parbat district. The total area of the river basin is 676.0321 sq. km.
Fig.1. Location of Study Area
-
Statement of Problem
The upper reaches of the Modi River Basin are found in the Annapurna Conservation Area (ACA), which provides a habitat for endangered species. In addition to environmental services, the steep slope (high slope) of the river and the water resources available throughout the year provide enormous potential for hydroelectric power generation. These structures are easily damaged by devastating floods and landslides. Furthermore, there is a lack of research on the design of hydraulic structures for hydrological events in the watershed.
-
Objectives
The main objective of this study is to analyze the flood around Modi catchment. To achieve this goal following are the secondary objectives of this study:
-
To develop a rainfall-runoff hydrologic model for Modi River basin using HEC-HMS.
-
To map the flood inundation in the river basins using HEC-RAS/HEC-Geo-RAS.
-
To identify flood susceptible areas around the Modi Catchment.
-
-
Scope of the Study
This study will prepare a flood forecasting model along with flood hazard and inundation maps in the flood-affectedregion of the Modi River Basin. Also, it provides in-depth knowledge in using hydrological and hydraulic models for flood forecasting. This research will contribute to flow forecasting in different parts of countries. The countries will have extended flood forecasting and early warning system to minimize the loss of life and properties.
-
Limitations of the Study
The limitations of the study include the following:
-
The spatial distribution of climate and meteorological data collection stations in the basin is poor.
-
The entire basin is represented by single set of meteorological component. Therefore, this model used Thiessen Polygon Method for spatial distribution of rainfall. Snowmelt component was not considered for the runoff contribution in the river basin.
-
The assigned parameters were not necessarily the physical reflection of the basin.
-
-
LITERATURE REVIEW
-
HEC-HMS Modeling
Hydrologic Engineering Centre-hydrological modeling system (HEC-HMS) of United States Army Corps of Engineers (USACE) is a very flexible and efficient semi-distributed conceptual hydrological model for simulation of the rainfall- runoff process in a wide range of geographic areas, from large river basin water supplies and flood hydrology to small urban and natural watershed runoffs. It requires daily rainfall, long- term average monthly potential evapotranspiration, discharge and stage data of the basin (for calibration and validation), and geographical information of the basin to get the simulated runoff as output [2].
HEC-HMS uses separate models to represent each component of the runoff process, including models that compute runoff volume, models of direct runoff, and models of base flow. Each model run combines a basin model, meteorological model, and control specifications with run options to obtain results. The selection of an appropriate model for each component depends upon the applicability and limitations of each method, availability of data, suitability for the same hydrologic condition [3].
In Germany, [3] performed event and continuous modeling of HEC-HMS to confirm the applicability of the model in Manitoba basins. The study confirmed that the model is well suited to determine runoff values for flood forecasting and other purposes. The performance of different rainfall estimators in hydrological modeling was evaluated in two basins in Japan [4]. Furthermore, [4] conducted a study on hydrological simulations of small river basins in Northern Kyushu, Japan, during the extreme rainfall events of July 5-6, 2017. The study focused on only a single rainfall event and demonstrated the possibility of applying a hydrological model developed in a gauged basin to a neighboring ungauged basin to estimate its hydrological properties. [5] applied the HEC-HMS model to simulate the river flow in the Gilgel Abay Catchment (1609 km2) watershed located in Upper Blue Nile Basin, Ethiopia. [2] calibrated and
validated the HEC-HMS model in the Wanan catchments in China. [6] used HEC-HMS to develop the rainfall-runoff simulation model has for the Sutlej river basin situated in Northern India. [7] simulated the rainfall-runoff process by dividing the Shipra river basin into three sub-basins and created one routing reach using the HEC-GeoHMSand Arc-Hydrotool in ArcGIS.
[8] calibrated and validated the hydrological model and used the model to simulate the flow at the outlet of each sub-basin of the Marshyangdi river basin. Likewise, many studies show the ability of the HEC-HMS model to simulate and forecast streamflow and its suitability in the Kankai, and Narayani river basin of Nepal [9,10]. Also [11] used HEC-HMS to analyze the performance of the different channel sections that will produce the best results for the Gandaki River basin. Furthermore, in the Koshi River basin the simulation was carried out by model software HEC-HMS and four types of modeling results in terms of parameters namely deficit and Constant loss parameters, Clark runoff transform parameters, Monthly base flow (m3/s) parameters, and Muskingum routing parameters were estimated. -
HEC-RAS Modeling
One of the most widely used models used by hydraulic engineers in the channel flow analysis and floodplain delineation is HEC-RAS (Hydraulic Reference Manual, 2010). It is a one-dimensional steady flow hydraulic model developed by the US Army Corps of Engineers; it has wide acceptance among government agencies. With HEC-RAS, it is possible to simulate water surface profiles for gradually varied steady flow and the effects of various obstructions such as culverts, bridges, structures in the overbank region, and weirs. Several applications of the HEC-RAS model to determine the extent of inundation resulting from a given flood have been reported [12,13]. [14] applied the HEC-RAS model to calculate water surface profiles corresponding to selected flood events downstream of Al Wahda Dam in Sebou basin in Northern Morocco. Software often used in combination with HEC-RAS is ArcGIS. It comprises a set of tools, utilities, and procedures for working with geospatial data. The basic inputs to HEC-RAS include the river discharge, channel, floodplain geometry, and channel resistance.
[15] used HEC-RAS (Hydrologic Engineering Centers River Analysis System) for comprehensive hazard mapping and risk assessments in the downstream region of the Karnali River basin for different return-period floods. In Nepal, based on the HEC-RAS and its ArcGIS extension (HEC-GeoRAS), previous studies have mapped the flood hazard in the Bishnumati and Balkhu rivers of Kathmandu [16]. [17] used HEC-RAS, HEC- GeoRAS, and ArcGIS for the preparation of 1D flood plain maps and the WECS/DHM method to analyze the flood frequency in different return periods. The maps were prepared based on field observation of damages and hazards as well as using available topographic maps, digital data, satellite imageries, and aerial photographs and the information gathered was digitized and analyzed using mainly ArcView and ILWIS GIS systems and HEC-RAS by [18]. [19] performed HEC-RAS modeling for flooding situations to determine the water surface profile along the eight cross-sections of the Ramghat and prepared floodplain map using the topographic map and theGoogle Earth Map. [20] used HEC-RAS and ArcGIS for flood modeling of Patu River in Tulsipur city of Nepal and analyze the flooding impact on encroached settlement along the river. However, flood hazard mapping and risk assessment in Nepal is still very rudimentary where most of the flood protection works are carried out at the local level without proper planning and without considering the problem at the river basin scale [21].
-
Research Gap
Nepal lacks long series of flow and precipitation data. In addition, research on flood modeling and flood mapping in Nepal, especially in small and medium rivers, is limited. Existing researches lack to cover the flood modeling and inundation mapping at once. Therefore, researches need to be carried out with the most recent data.
The hydrological model estimates the frequency of floods and provides information for flood routes and flood forecasts. The calibrated and validated models can be used for climate impact assessment, land use change and integrated watershed management. The results of the hydraulic model can be used for flood risk mapping, flood disaster assessment, real-time flood forecasting, flood-related engineering, water resources planning, floodplain erosion research, water transportation, sediments, floodplain ecology and river systems [10].
-
-
DATA/ MATERIALS USED
The data required for hydrologic modelling (HEC-HMS) are
-
Digital Elevation Model (DEM)
-
Land Use and Soil Cover
-
Climate Data (precipitation, temperature)
-
Discharge Data.
-
Digital Elevation Model(Dem)
The Digital Elevation Model (DEM) is the basic terrain daa required to develop the model using HEC-GeoHMS. DEM of 30 m resolution was downloaded from the open source USGS as represented in Fig. 2. The mosaicked DEM was then clipped for Modi River Basin. Once the mosaic operation was completed, the coordinate system was chosen. In case of Nepal, Modi Basin, Projected Coordinates Systems UTM WGS1984 zone 44N was chosen.
Fig.2. 30m Resolution SRTM DEM Covering Whole Basins of Nepal Rivers for Mosaic Operation
-
Land Use
Land use map for Modi was downloaded from ICIMOD. The land use in the Modi River basin has been classified into seven categories as forest, shrub land, grass land, agriculture areas, water body, snow glacier and built-up area. The developed area in the river basin is less and is dominated by forest as shown in Fig. 3. The upper reaches of the basin area are covered by the snow.
Fig.3. Land Use Classification of Modi River Basin
-
Soil Cover
Soil cover map of Modi River basin was downloaded from Soil and Terrain (SOTER) database programme ISRCI (Fig. 4). Soil layers for Modi constitutes of six types of soil with Gelic LEPTOSOLS the dominant type.
Fig.4. Soil Classification of Modi River Basin:
Table I. Details of Meteorological Stations Located in Modi Catchment
-
Rainfall
The precipitation data were acquired from the Department of Hydrology and Meteorology (DHM) of the Government of Nepal. Fig. 5 shows the location of precipitation gauging stations. The data gaps were filled using the normal ratio method. The subbasin average precipitation data was computed externally using the Thiessen polygon method in ArcGIS. Daily precipitation from the following seven gauges (available nearby stations of the Modi Basin) was used in this study: Ghandruk, Ghorepani, Kushma, Karkineta, Bhadaure Deurali, Salyan, and Lumle. The relative areas of each rainfall measuring station along with their latitude and longitude are mentioned in Table
-
The areal rainfall depth (weighted rainfall) was calculated for the year 2006 to 2013 and given as input values for HEC-HMS. The graph thus obtained is shown in Fig. 6. The month of July recorded the highest average rainfall from 2006-2013 whereas December is seen as the driest month for the same period.
Fig.5. Precipitation Gauge Station of Modi Basin
Fig.6. Precipitation Pattern in Modi River Basin from 2006 to 2013
Index No.
Station Name
District
Station Type
Longitude(Decimal Degrees)
Latitude(Decimal Degrees)
Area(Square Km)
613
Karki Neta
Parbat
Precipitation
83.748
28.177
34.409
614
Kushma
Parbat
Climate
83.677
28.225
65.342
813
Bhadaure Deurali
Kaski
Precipitation
83.822
28.266
8.134
814
Lumle
Kaski
Climate
83.818
28.297
21.367
821
Ghandruk
Kaski
Precipitation
83.801
28.376
388.539
829
Sallyan
Kaski
Precipitation
83.796
28.277
74.204
619
Ghorepani
Myagdi
Precipitation
83.701
28.399
84.035
-
-
Evapotranspiration
Evapotranspiration is responsible for returning approximately 50-60% of precipitation to the atmosphere. For this study, the monthly average evapotranspiration was obtained using Thornthwaite (1948) equation at the subbasin level and the calculated accordingly. Fig. 7 shows the line chart of average potential evapotranspiration (PET) of the Modi river basin based on the temperature by using Thornthwaite Method. The mean monthly temperature from 1992 to 2016 of the Kushma Climate station was used. It is required for computing the potential evapotranspiration.
Fig.7. Monthly Average Potential Evapotranspiration of Modi River Basin
-
Discharge
-
There are three discharge stations in the Modi River basin namely Annapurna, Nayapul, and Kaligandaki. Daily discharge data of these stations were obtained from the Department of Hydrology and Meteorology (DHM) of Government of Nepal (Fig. 8). Out of the above three mentioned discharge stations, the Modi River Station No. 406.5 was used for calibration and validation of the hydrological model. The geographical location detail of the station is demonstrated in Table II. The pattern of daily discharge of the Modi River Station is illustrated in the graphs Fig. 9 from the data obtained in the period of 2006 AD to 2013 AD.
Fig.8. Hydrological Stations of Modi River Basin
Table II. Location of Nayapul Modi River Hydrological Station
Station No. |
Station Name |
River |
Latitude |
Longitude |
Elevation (m) |
Selected Period (AD) |
Modi |
||||||
River- |
Modi |
2006- |
||||
406.5 |
Nayapul |
River |
28.2250 |
83.7042 |
701 |
2013 |
600
500
400
300
200
100
0
Year
600
500
400
300
200
100
0
Year
Discharge (m3/s)
Discharge (m3/s)
Fig. 9. Observed Flow of Modi River of Nayapul Station (406.5) for the Year 2006 to 2013
-
METHODOLOGY
-
Hydrological Model Development
Rainfall runoff modelling was performed with the help of HEC- HMS and HEC-GeoHMS a hydrological extension in ArcGIS. An overview of working mechanism of rainfall runoff model is shown with the help of framework in Fig. 10.
Fig.10. Methodology Flow Chart for Hydrological Modelling
Terrain Preprocessing Using HEC-GeoHMS
For Terrain Preprocessing Mosaic DEM, the clip was loaded to the ArcMap. Terrain preprocessing uses DEM to create a stream network and catchments. Before carrying out terrain pre- processing, the input terrain data DEM was refined using DEM reconditioning. The steps in the terrain preprocessing menu were executed in order, from top to bottom. The steps included were filing sinks, flow direction, flow accumulation, stream definition, stream segmentation, catchment grid delineation, catchment polygon processing, drainage line processing, and adjoint catchment processing.
Basin Processing
The batch point was created at the outlet of the catchment and the watershed was delineated. The resulting catchment area for
the Modi river basin was 676.034 km2. The HEC-GoeHMS project setup menu was completed by defining two feature classes: ProjectPoint and ProjectArea. For the sub-basin and river, physical characteristics were calculated based on the refined DEM. The computed characteristics for river included river length and river slope. Similarly computed characteristics for basin included basin slope, longest flow path to the basin, basin centroid, centroid elevation, and centroidal longest flow path. To calculate basin slope, the watershed slope was required which was calculated using the ArcHydro tool.
Hydrologic Parameters
The Hydrological Parameters menu in HEC-GeoHMS provides tools for estimating and plotting various watershed and river parameters used in HEC-HMS. Accordingly, the river auto name, sub-basin auto name was assigned. HMS processes for modeling loss, transform, base-flow, and routing were selected.
Developing HEC-HMS Model Files
In this step, model files such as background- map file, basin model file, and meteorological model file required for HEC- HMS were generated. Finally, the background map file and basin file was created to export to HMS.
In this case, Simple Canopy and Simple Surface methods were used. A canopy component was included to represent interception and evapotranspiration. Similarly, a surface component was added to represent water captured in surface depression reservoir. The SCS curve number was employed to simulate the infiltration loss. The SCS (Soil Conservation Service) unit hydrograph method was used to model the transformation of precipitation excess into direct surface runoff. The constant monthly model was employed to simulate baseflow. Initially, some appropriate values were given in optimization trials and the sensitivity of each parameter was checked.
Evaluation of Model Performance
The goodness of fit was assessed using hydrograph visualization and computed statistical performance measures. Factors like Nash-Sutcliffe Model Efficiency (NSE), Root Mean Square Error (RMSE) and R2 were the basis to assess the efficiency of the hydrological model. NashSutcliffe (NSE) coefficient of model efficiency was used to measure the goodness-of-fit between the observed and simulated flow time series.
HEC-HMS Modelling
= 1
=1
( )2
( )2
(1)
The Modi HMS file was created in the HEC-GeoHMS and was opened in the HEC-HMS 4.6 window. After opening the file, the basin file looked as shown in Fig. 11.
=1
2 = ()2()2
()2
= 1 (
)2
=1
RMSE ranges from 0 (optimal value) to 0.5 (very good performance) ratings. Lower values of RMSE indicate a lower root mean square error normalized by the standard deviation of the observations, which indicates the appropriateness of the model simulation. The performance ratings of watershed models based on the above statistical parameters are summarized in Table IV.
Fig.11. HMS Model in HEC-HMS Window of Modi River Basin
Basin Model
In addition to building the model schematic in HEC-HMS, model components of the sub-basin were defined. Since the sub-basin element is used to convert rainfall to runoff, the information about the method used to compute loss rates, hydrograph transformation and baseflow was needed for sub- basin element. Table III summarizes the model components and the calculation methods selected in this study.
Table III. Model Components and Calculation Methods
Component
Calculation Method
Canopy
Simple Canopy
Surface
Simple Surface
Loss
SCS Curve Number
Transform
SCS Unit Hydrograph
Baseflow
Constant Montly
Table IV. General Performance Ratings for Watershed Models
Performance Rating
NSE
R2
Very Good
0.75<NSE1.00
0.75<R21.00
Good
0.65<NSE0.75
0.65<R20.75
Satisfactory
0.50<NSE0.65
0.50<R20.65
Unsatisfactory
NSE0.50
R20.50
Sensitivity Analysis
A sensitivity analysis was performed on five parameters. The evaluated parameters were the CN Number, lag time, impervious percentage, maximum canopy storage and maximum surface storage. The initial calculated and assumed parameter values were kept as reference values, and the analysis was performed by changing one parameter at a time from -75% to + 75% and keeping all other parameters unchanged in 15% increments. The sensitivity of the parameters that define the initial conditions was not evaluated. The percentage change in NSE due to the percentage change of each parameter was plotted on the graph and ranked accordingly. The parameter showing the largest change in the simulated volume was
considered to be more sensitive to each situation. Firstly, the sensitivity of each parameter was checked and then the values of parameters were adjusted.
HEC-HMS Calibration and Validation
Once all the parameters were assigned as input in the HEC- HMS project of the basin, output was obtained as the simulated flow values, which were then compared with the observed data over the same period to determine the consistency of the result. The data for the flow was available from 2006 to 2013. [22] conducted the study by dividing two-third of available data for calibration and one-third for validation. Likewise, in this study calibration was done for five years (2006 to 2010 AD) and the remaining three years period (2011-1013 AD) was used for validation. Calibration procedure was carried out manually and also through optimization techniques to match the observed values and simulated values. The manual calibration of seven parameters was performed using observed streamflow data from the Modi River Station (Station No. 406.5). The calibration was initialized using ranges obtained from the previous research studies [23, 24, 25, 26]. The values of the parameters were estimated by observation and analysis of stream and basin characteristics in ArcGIS. Since some of the required parameters cannot be estimated precisely, the parameters were calibrated by systematic approach for the best fit of the observed and simulated stream flow. The validation of the optimized parameters was performed by running the prepared model using the same parameters used in the calibration period.
-
Hydraulic Model Development
The floodplain mapping method is completed in two stages: the data processing from the RAS mapper in stage 1 and the execution of the HEC-RAS model in stage 2. The floodplain mapping method is shown in Fig. 12.
Fig.12. Methodology Flow Chart for Hydraulic Modelling
At first, HEC-RAS 6.0.0 was downloaded. Georeferencing of the area of the case study was completed after the shapefile of Modi Basin was created, which is a key parameter of the RAS mapper. Digital elevation model (DEM) images are used to classify magnitude of the flood and flood mapping. DEM of
12.5 m resolution was downloaded from ALOS PALSPAR. The geometry in HEC-RAS contains information on cross- sections, river banks, flow paths, roughness coefficient, etc. The
river geometries such as river centerline, bank line, flow path line, and cross-section were created in RAS mapper tools of HEC-RAS. Following are floodplain mapping input parameters in the RAS-mapper.
River Center line: The center line of the river indicating dark blue marked was used to build the river reach of the Modi river basin in the HEC-RAS model as in Fig. 13.
Bank lines: The riverbank line marked in red was used to distinguish the main river channel from the riverbank floodplain area shown in Fig. 13.
Flow path lines: The flow path lines marked in light blue was used to adjust the length of the river along the lower reaches of the river, the left bank and the right bank, as shown in Fig. 13. Cross-sections cut lines: Section cut lines with green cross marks drawn perpendicular to the flow direction were used to extract altitude data to create a surface profile throughout the channel flow. In addition, it must cross the center line of the channel, bank paths and flow path lines as in Fig. 13.
Fig.13. RAS-mapper Processing Data
The river centerline and cross section profile were importedfrom RAS mapper into the Geometric Data Editor window. In addition, the elevation data from the DEM was extracted. Fig.
14 shows the Modi river basin centerline imported RAS- mapper file with blue marked and green marked cross-section cut lines in river terrain background in the HEC-RAS Geometric data editor window.
Fig.14. Geometric data Editor Window in HEC-RAS
The Mannings roughness coefficient was taken 0.03 for the main water flowing channel and 0.035 for right over bank and left over bank portion of the river [1].
To perform the flood analysis, 1D steady flow simulation was carried out under subcritical flow regime. The Gumbel
distribution method was used to calculate flood values for 10 50, 100 and 150 return periods. The results of the flood frequency analysis of the Modi River are shown in Table V.
Table V. Predicted Discharge of Modi River at Different Return Periods
Return Periods
Discharge (m3/sec)
10
776.7
50
1079.0
100
1206.8
150
1281.4
These flood values and the appropriate boundary conditions were used as input for steady flow data. Since the selected flow regime was subcritical, boundary condition was defined only at the downstream end of the river. It was defined by the normal depth which is the slope of the river bed. The boundary condition at the junction was predefined by the software. After simulating the system in HEC-RAS, the results were obtained in the form of depth, velocity and elevation of the water surface in the RAS-mapper window.
-
-
RESULTS AND DISCUSSIONS
-
Sensitivity Analysis
During the sensitivity analysis, it was found that the CN number, impervious percentage, maximum canopy storage, maximum surface storage, were the most sensitive parameters for simulated flow during the calibration of the Modi river basins. This is also evident in the chart in Fig. 15. Based on the sensitivity analysis, the classification of model parameters is shown in Table VI.
Fig.15. Sensitivity Analysis of HEC-HMS Model for % Change in NSE for the Calibration Period
S.N.
Model Parameters
1
CN number
2
Impervious
3
Maximum Surface Storage
4
Maximum Canopy Storage
5
Lag Time
S.N.
Model Parameters
1
CN number
2
Impervious
3
Maximum Surface Storage
4
Maximum Canopy Storage
5
Lag Time
Table VI. Sensitivity Ranking of Model Parameters of Modi River Basin
-
Model Calibration
The calibration of the HEC-HMS model was carried out based on the observed flow data of the Modi River Basin Hydrological Station (Station No. 406.5) from 2006 to 2010. Fig. 16 compares observed flow to simulated flow for the calibrated years, 2006 to 2010. The blue line represents the total simulated outflow and the orange line represents the observed outflow measured at the gauging station. The NashSutcliffe coefficient of efficiency (NSE) was found to be 0.78 as summarized in Table VII. Higher values of NSE (closer to one) indicated better agreement. These values were within acceptable ranges and indicated a very good fit. Fig. 16 shows a comparison of the observation and simulation results for the continuous modeling of the daily flow series of the Modi river basin. Fig. 17 shows a scatter diagram of the simulated flow and the measured flow, and shows a strong positive correlation (0.81).
The model simulated the daily flow series very well, but the peak flow was underestimated. Except for the extreme peaks, the HMS model represented almost all the peaks that were seen around the monsoon of each year.
Table VII. Performance Measures for Calibration Period
Modi Basin
Performance Measures
Calibration Period (2006-2010)
Performance Rating
NSE
0.78
Very Good
R2
0.81
Very Good
RMSE
0.50
Very Good
Fig.16. Daily Observed and Simulated Discharge for the Entire Calibration Period
Fig.17. Comparison between the Observed and Simulated Flow for Calibration Period
-
Model Validation
To verify the output of this model, it was also validated from the year 2011 to 2013 AD. The resulting graph for the validation period is shown in Fig. 18. The flow pattern of simulated flow at all stations during validation was similar to the calibrated flow pattern.
The statistical performance indicators for the HEC-HMS are summarized Table VIII. Overall, based on the Nash-Sutcliffe coefficient of 0.78, indicated good model performance. Finally, according to the coefficient of determination (R2) of 0.80, there was a good correlation between the simulated flow and the flow observed at the outlet of the basin, as shown in Fig. 19. The time of peak August 3, 2012 was well captured by the model during the validation.
Table VIII. Performance Measures for Validation Period
Modi Basin
Performance Measures
Calibration Period (2006-2010)
Performance Rating
NSE
0.78
Very Good
R2
0.80
Very Good
RMSE
0.50
Very Good
Fig.18. Daily Observed and Simulated Discharge for The Entire Validation Period
Fig.19. Comparison between the Observed and Simulated Flow for Validation Period
-
Hydraulic / Flood Inundation Modelling Results
The flow values from Modi River Station 406.5 was used to calculate the flood values for different return periods. The flow value was obtained from Gumbel distribution method. The inundation map of Modi river due to 10, 50, 100 and 150 year return flood is shown in the Fig. 21. The dark blue color shows the greater depth in the Fig. 21 and light blue color shows the reduced depth. The inundated area and maximum water level given by HEC-RAS during 10, 50, 100 and 150-year flood is tabulated in Table IX. The highest value of water depth during 10, 50, 100 and 150-year flood in this river are 8.36, 8.56, 8.67 and 8.71 respectively. Cross sections representing the
maximum water level during the four return periods along the lower reach of Modi River basin is shown in Fig. 22.
The results from the flood analysis show that the area covered with the forest is more vulnerable to flood as demonstrated in Fig. 21 (a), (b), (c), (d). With an increase in the flow value, the inundation in the forest area seems to increase. Approximately two-fifths land of the river basin belongs to forest. It has been found that 9.73%, 9.90%, 9.97% and 10.00% of forest gets
drowned due to 10 years, 50 years, 100 and 150 years flood. At present there exists no human habitation in the risk-prone area but construction of any structures and human habitation should be discouraged in the future due to possibility of inundation by the flood in that area, as observed.
The man-made structures currently located in the study area are a suspension bridge and a hydropower station as shown in Fig.
20. The results show that the structure of the United Modi hydropower station is in flood haardous zone however the suspension bridge is not in danger due to its placement high above the maximum water level.
Table IX. Area inundated and Maximum Water Level in Modi River Basin during 10, 50, 100 and 150 Years
Return Period (year)
Inundated area (m2)
Max. Water level (m)
10
25647.49
8.36
50
26114.01
8.56
100
26283.04
8.67
150
26374.45
8.71
Fig. 20. Google Satellite Map of Modi River
a b
c d
Fig. 21. Inundated Areas of Modi River Basin: Flood Hazard Mapping for Return Periods (a. 10-year, b. 50-year, c. 100-year, d. 150-year)
Fig. 22. Cross-section of the Modi River (lower reach) at RS 1447 at 150 Years Return Flood
-
-
CONCLUSION
The hydrological model using HEC-HMS was developed to study the impact of rainfall on surface runoff and peak discharge in the Modi River basin. Interception and depression storage were modeled using canopy and surface Methods. The infiltration process was modeled using a SCS curve number method whereas the constant monthly method in HEC-HMS was used to model the baseflow. The SCS unit hydrograph method was used to model the transformation of precipitation excess into direct surface runoff. Observed precipitation data was obtained from DHM. Values were assigned to parameter in HMS to get a good calibrated model. Calibration and validation of the modeling system was conducted for five years period (2006-2010) and three (2011- 2013) years period respectively. The HEC-HMS conceptual model was successfully calibrated and validated for use in the Modi river basin. Statistical measurements of the performance of the HEC-HMS model are summarized in Table X. The global Nash-Sutcliffe Efficiency criteria were 0.78 and 0.78 for the calibration and validation
periods, respectively, indicating a very good fit. The coefficients of determination (R2) for the calibration and validation periods were 0.81 and 0.80, respectively, indicating a very good fit. The RMSE, which assesses the appropriateness of the model, was 0.50 (0.50) for the calibration (validation) period, indicating very good performance. Some discrepancies (two months) were seen in the timing of peak simulated and observed peak values for the calibration periods. However, the time of peak August 3, 2012 was well captured by the model during the validation as well as entire simulation period. Even with these considerations, the HEC-HMS model performed at a reasonably acceptable level and was able to replicate the rainfall-runoff process of Modi River Basin.
Table X. Performance Assessment of the HEC-HMS Model
Performance Measures
Calibration Period (2006-
2010)
Validation Period (2011-
2013)
Entire Period (2006-2013)
Performance Rating
NSE
0.78
0.78
0.78
Very Good
R2
0.81
0.81
0.80
Very Good
RMSE
0.50
0.50
0.50
Very Good
In addition to that, the flood map produced by HEC-RAS was used to study the extent and characteristics of floods. In Modi river, the discharge has been obtained as 776.7 m3 /s, 1079.0 m3
/s, 1206.8 m3 /s and 1281.4 m3 /s for 10, 50, 100 and 150 years flood, for which the inundation area was observed as 0.0256 km2, 0.0261 km2, 0.0263 km2, 0.0264 km2 respectively. The basin is dominated by forest lands. The flood plain mapping showed the inundation of forest and trees. It indicated that the future settlement at the vicinity of river is to be at high risk of flood. The study also showed that the structure of hydropower station lies in flood hazardous zone.
-
ACKNOWLEDGEMENT
The authors would like to thank Department of Hydrology and Meteorology (DHM), Nepal for providing the hydro meteorological data. Also, we are grateful to Pashchimanchal Campus, Pokhara, Nepal for rendering a friendly environment for the research work.
REFERENCES
-
B. Parajuli, D. K. Gautam, and R. Talchabhadel, Development of flood forecasting model for Narayani basin , Nepal A technical report on development of flood forecasting model in Narayani river basin , Nepal, April 2017.
-
J. O. Oleyiblo and Z. J. Li, Application of HEC-HMS for flood forecasting in Misai and Wanan catchments in China, Water Sci. Eng., vol. 3, no. 1, pp. 1422, 2010, doi: 10.3882/j.issn.1674- 2370.2010.01.002.
-
H. A. K. M. Bhuiyan, H. McNairn, J. Powers, and A. Merzouki, Application of HEC-HMS in a cold region watershed and use of RADARSAT-2 soil moisture in initializing the model, Hydrology, vol. 4, no. 1, pp. 119, 2017, doi: 10.3390/hydrology4010009.
-
P. C. Shakti, T. Nakatani, R. Misumi, K. Iwanamai, and K. Hirano, Runoff modeling for river basins in Japan using HEC-HMS, no. 48, pp. 104105, 2016.
-
M. Al-Mukhtar and F. Al-Yaseen, Modeling water quality parameters using data-driven models, a case study Abu-Ziriq marsh in south of Iraq, Hydrology, vol. 6, no. 1, 2019, doi: 10.3390/hydrology6010021.
-
P. Modi, C. M. Rao, and R. V Kale, Rainfall-runoff simulation using
HEC-HMS model for Sutlej rainfall-runoff simulation using HEC- HMS MODEL for Sutlej river basin , India, November 2019.
-
S. Sahu, S. K. Pyasi, R. V. Galkate, and R. N. Shrivastava, Rainfall- runoff modeling using HEC-HMS model for Shipra river basin in Madhya Pradesh, India, Int. J. Curr. Microbiol. Appl. Sci., vol. 9, no. 8, pp. 3440-, 2020, doi: 10.20546/ijcmas.2020.908.398.
-
K. Basnet, R. P. Paudel, and B. Sherchan, Analysis of watersheds in Gandaki Province , Nepal using QGIS, Technical Journal, vol. 1, no. 1, pp. 1628, 2019, https://doi.org/10.3126/tj.v1i1.27583.
-
N. P. Gautam, Flow routing with semi-distributed hydrological model HEC- HMS in case of Narayani river basin, J. Inst. Eng., vol. 10, no. 1, pp. 4558, 2014, doi: 10.3126/jie.v10i1.10877.
-
J. Khadka and J. Bhaukajee, Rainfall-Runoff simulation and modelling using HEC-HMS and HEC-RAS Models: Case Studies from Nepal and Sweden, Lund Univ., pp. 169, 2018, [Online]. Available: www.tvrl.lth.se.
-
E. Narayan and P. Gautam, Hydrological modeling with HEC-HMS in different Channel Sections in case of Gandaki river basin, Glob. Journals Inc, vol. 15, no. 2, 2015.
-
K. A. Al-Qudah, Floods as water resource and as a hazard in arid regions: A case study in southern jordan, Jordan J. Civ. Eng., vol. 5, no. 1, pp. 148161, 2011.
-
B. Manandhar, Flood plain analysis and risk assessment of Lothar Khola, pp. 77, 2010, doi: 10.13140/2.1.1664.1289.
-
A. Azouagh, R. El Bardai, I. Hilal, and J. Stitou el Messari, Integration of GIS and HEC-RAS in floods modeling of Martil river (Northern Morocco), Eur. Sci. Journal, ESJ, vol. 14, no. 12, pp. 130, 2018, doi: 10.19044/esj.2018.v14n12p130.
-
D. Aryal et al., A model-based flood hazard mapping on the southern slope of Himalaya, Water (Switzerland), vol. 12, no. 2, 2020, doi: 10.3390/w12020540.
-
S. Dangol and A. Bormudoi, Flood hazard mapping and vulnerability analysis of Bishnumati river, Nepal, Nepal. J. Geoinformatics, Surv. Dep. Nepal, pp. 5, 2015.
-
P. Banstola, P. Krishna, and B. Sapkota, Flood risk mapping and analysis using hydrodynamic model HEC-RAS: A case study of Daraudi River, Chhepatar, Gorkha, Nepal, Grassroots J. Nat. Resour., vol. 2, no. 3, pp. 2544, 2019, doi: 10.33002/nr2581.6853.02033.
-
M. R. Dhital, R. Shrestha, G. . Shrestha, and D. Tripathi, Hydrological hazard mapping in Rupandehi district, west Nepal, J. Nepal Geol. Soc., vol. 31, no. 1991, pp. 5966, 1970, doi: 10.3126/jngs.v31i0.261.
-
K. Basnet and D. Acharya, Flood analysis at Ramghat, Pokhara, Nepal Using HEC-RAS, Tech. J., vol. 1, no. 1, pp. 4153, 2019, doi: 10.3126/tj.v1i1.27591.
-
K. Basnet, B. B. Sadadev, and B. Sherchan, Flood modelling of Patu River in Tulsipur city of Nepal and analysis of flooding impact on encroached settlement along the river, Int. J. Adv. Res. Ideas & Inv. in Technology, vol. 6, no. 4, pp. 375-384, 2020.
-
S. Dangol, Use of Geo-Informatics in flood hazard mapping: A case of Balkhu river, Nepal. J. Geoinformatics, vol. 13, pp. 5257, 2014.
-
N. Pokharel, K. Basnet, B. Sherchan, and D. Thapaliya, Assessment of hydropower potential using SWAT modeling and spatial technology in the Seti Gandaki River, Kaski, Nepal, IEEE-SEM, vol. 8, no. 7, 2020, eoi: 10.11230/ieeesem.2020.07.16017.
-
R. Gyawali and D. W. Watkins, Continuous hydrologic modeling of snow-affected watersheds in the Great Lakes basin using HEC- HMS, J. Hydrol. Eng., vol. 18, no. 1, pp. 2939, 2013, doi: 10.1061/(asce)he.1943-5584.0000591.
-
A. Ahbari, L. Stour, A. Agoumi, and N. Serhir, Estimation of initial values of the HMS model parameters: Application to the basin of Bin El Ouidane (Azilal, Morocco), J. Mater. Environ. Sci., vol. 9, no. 1, pp. 305317, 2018, doi: 10.26872/jmes.2018.9.1.34.
-
F. Alam, Q. Alam, S. Reza, S. M. Khurshid-ul-Alam, K. Saleque, and
H. Chowdhury, A Review of Hydropower Projects in Nepal, Energy Procedia, vol. 110, pp. 581585, Mar. 2017, doi: 10.1016/j.egypro.2017.03.188.
-
J. A. Holberg, Downward model development of the soil moisture accounting loss method in HEC-HMS: Revelations concerning the soil profile, Purdue Univ. West Lafayette, pp. 94, 2015.