
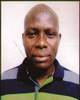
- Open Access
- Authors : Durojaye Adeyemi J , Oluyemi- Ayibiowu Bamitale D. , Durojaye Omolola T
- Paper ID : IJERTV11IS050127
- Volume & Issue : Volume 11, Issue 05 (May 2022)
- Published (First Online): 23-05-2022
- ISSN (Online) : 2278-0181
- Publisher Name : IJERT
- License:
This work is licensed under a Creative Commons Attribution 4.0 International License
Improvement In Permeability and Strength Characteristics of Lateritic Soil using Bacillus Mycodies_MTO47265
Durojaye Adeyemi J
The Polytechnic Ibadan, Department Of Civil Engineering, Ibadan ,
Oyo-State, Nigeria.
Oluyemi- Ayibiowu Bamitale D.
The Federal University Of Technology, Department Of Civil And Environmental Engineering,
Akure, Ondo-State, Nigeria;
Durojaye Omolola T
University Of Ibadan, Department Of Microbiology, Ibadan, Oyo-State, Nigeria.
Abstract The relatively new and innovative soil improvement technique known as MICP (Microbial-Induced Calcite Precipitation) has been successfully used as a treatment to decrease permeability and increase strength characteristics of lateritic soils. Bacillus mycodies_MTO47265 was employed in the treatment of lateritic soil. The lateritic soil particle size range defined by coefficient of uniformity Cu is 13.36, while its coefficient of gradation Cg is 0.6819. The dominant minerals present are Quartz, Muscovite, Kaoline and Geothite. The peak strength attained for cured treated specimens was 497.9 kPa after 28 days of curing. The percentage strength increase of
138.33 % was recorded at the 3 days of curing age when compared with the control specimen (CON) of the same age. The maximum percentage decrease in the coefficient of permeability of 42.92 % was achieved on 28 days of curing age in measure with the value obtained for control specimen of the same curing age of 28 days. Increase in atomic concentration of calcium and carbon, after treatment with Bacillus mycodies_MTO47265, was found to be 0.32 and 1.02 respectively. The specimen treated with Bacillus mycoides_MTO47265 shows fully clustered crystals morphology of calcite cementing larger portion of the pores of the soil matrix when compared with that of untreated specimen.
to the soil and environment [3]. This approach has been long ignored in the geotechnical community. This innovative technique that harness the activity of bacteria to improve the physical properties of soils as a sustained and environmentally responsible method for soil strengthening and other engineering applications [5], [6].
MICP is a biologically mediated method to induce in-situ cementation of granular soils [7] [8] through the bacteria- driven urea hydrolysis, which is calciumrich environment results in calcium carbonate (CaCO3) precipitation.
PRODUCTION OF CACO3
This process is often called microbially induced calcium carbonate precipitation (MICP). Urease-producing bacteria (UPB) through its metabolic activity under proper cultivation process. The urease enzyme triggered the MICP biochemical reaction by hydrolyzing urea (CO (NH2)2) through the following:
NH2)2CO + 3H2O 2NH4 + + HCO3 – + OH-
(1)
+
The ammonium (NH4 ) increased the pH and caused the
3
biocarbonate (HCO -) to precipitate with calcium ion (Ca
2+)
Keywords Permeability, Bacillus mycodies_MTO47265, Lateritic Soil, Improvement, MICP
INTRODUCTION
from calcium chloride supplied in to form the calcium calcite (CaCO3):
CaCl2 + HCO3 – + OH- CaCO3+ H2O + 2Cl-
The use of lateritic soils has been employed in tropical areas as an engineering material in the construction of roads. The heavy and seasonal rainfalls common to this area posed a great threat on the constructed road because of the quick saturation with rainwater and after a short while no longer accessible to motorized transportation [1]. The relatively new and innovative soil improvement technique known as MICP (Microbial-Induced Calcite Precipitation) has been successfully used as a treatment to decrease permeability and increase the strength characteristics of such soils. [2] [3] described MICP has a great advantage on the existing soil improvement techniques such as chemical grouting.also in the transformation of rock and soil properties [4]. Soil improvement by MICP has met the requirement of green construction because the process exerts minimal disturbance
(2)
Bio-cementation was achieved when calcite crystals precipitated on the surface or formed bridges between existing soil grains. These calcite crystals bonded the soil particles and minimized movement of the grain, and hence the strength and stiffness properties of the soil [9] [1]. The increased strength and stiffness of bio-cemented soils allow considering them even as construction materials [10]. MICP is achieved by transporting (e.g. injecting, flushing or percolating) chemical reagents (urea and calcium chloride (CaCl2) and ureolytic bacteria, typically Sporosarcina pasteurii (formerly known as Bacillus pasteurii), to the location where soil treatment is required. Ureolytic bacteria catalyse the hydrolysis of urea to produce ammonium and carbonate ions, which react with calcium ions (e.g. calcium chloride) to form calcite that precipitates throughout the soil
matrix. The microbial induced calcite crystals bridge the adjacent soil particles, and hence increase the shear strength and stiffness of soil while retaining high permeability [11],[12].
Civil Engineers, during construction face challenges with certain regional soil deposits with poor geotechnical properties. Over the years, most soil stabilization had been carried out with different chemical additives such as lime, asphalt, sodium silicate, urethane, cement, fibres and even geo-synthetic materials which are not only eco-friendly but also toxic, costly and also contaminate the underground water. This study was aimed at reducing the permeability and
increasing strength characteristics of lateritic soil using
Bacillus Mycodies_MTO47265
MATERIALS AND METHODS
Soil
The Lateritic soil sample used in this study was collected at laterite mass deposit site (N070221 49.7211, E 040001 3.2811) located at Adegbayi, along Ile-Ife Ibadan Expressway in Egbeda Local Government Area, Oyo-State. The soil samples were collected at a depth of 1.5 m below the natural earth surface to avoid organic matter.
Plate 1: Map of Egbeda Local Government Area, Oyo-State, showing the collection centre.
X-ray Diffraction
This was carried out by using Empyrean X-ray Diffraction instrument (Malvern Pan Analytical) with Pan Analytic Xpert Highscore plus attached with International Centre for Diffraction Data (ICDD) powder diffraction files (pdf) database.
Bacterial isolate
The Bacillus mycodies_MTO47265 previously screened for Urease enzyme production was collected from Microbial Physiology and Biotechnology Laboratory, Department of Microbiology, University of Ibadan, Ibadan, Oyo State, Nigeria.
Inoculum preparation
The previously screened Bacillus mycodies_MTO47265 was re-cultivated under aerobic conditions in a medium containing 30 g/l of Tryptic Soy Broth and 20 g of urea per litre of deionized water and the pH of the medium was adjusted to pH 7 and 9 and then was sterilized at 121oC and 15 psi for 15 min. The flask was incubated for 24 -36 h on a rotary shaker at 150 rpm and 30oC. Duplicate flasks were used. The bacteria was then incubated and grown until it reached approximately OD600 (Optical Density at 600 nm wavelength) of 0.8 1.2 in order to yield about 107 cells/ml. After incubation the bacterial suspension is centrifuged at 8000 rpm for 10 min. The supernatant was decanted and the pellet was used for treating the lateritic soil samples.
Cementation Reagents
The cementation reagents used in this study comprises 20 g of urea (CO (NH2)2, 2.8 g of calcium chloride (CaCl2), 3 g of Nutrient broth, 10 g ammonium chloride (NH4Cl) and 2.12 g sodim bicarbonate (NaHCO3) per 1 litre of deionized water.
Soil Treatment
Alongside the soil inoculum, a calcium chloride (CaCl2) solution was formulated in a separate container at a molar concentration of 0.5 M. The soil inoculum was manually mixed into the dry soil material, after which the CaCl2 was also added to the soil in an identical fashion. This was not only in order to deliver the bacteria cells and urea evenly to the soil, but also to prevent the premature precipitation of calcite crystals. The volume of inoculum and CaCl2 solution added remained constant for all samples, and was set in order to bring the air-dried soil moisture content up to the optimum moisture content of 16.5%. The wetted soil was then compacted into the polyvinyl tube using the methodology found in BS1377-6:1990 3.3.6.5, which employs the use of a
2.5 kg hammer. Each sample was compacted and leveled in order to reach a specified dry density of 1.7 mg/m3. This was conducted to ensure that the soil has a denser matrix configuration as possible. Each of the specimens was trimmed to obtain sample length of 100 mm and diameter of 50 mm.
Unconfined Compression Strength Test (UCS)
The unconfined compression strength test was carried out using BS1377:1990 Part 7:7. The soil samples were extruded from polyvinyl tube sampler. They were cut so that the specimen ratio (L/d) is approximately between 2 and 2.5. Where L and d are the length and diameter of soil specimen, respectively. The exact diameter of the top of the specimen at three locations 120o apart, and then make the same measurements on the bottom of the specimen. The average measurements were recorded as, well as that of diameter on the data sheet. The exact length of each of the specimens was measured at three locations 120o apart and the average of the measurements was recorded on the data sheet. The samples were weighed and the masses recorded, then the deformations (L) correspond to 15% strain (). Where Lo = Original specimen length. Each of the specimens was carefully placed in the compression device and centered it on the bottom plate. The device was adjusted so that the upper plate makes contact with the specimen and then set the load and deformation dials to zero. The load is then applied, so that the device produced an axial strain at a rate of 0.5% to 2.0% per minute, and then the load and deformation dial readings were recorded at every 20 to 50 divisions on deformation the dial. The load was kept applying until there was significant decreased on the specimen on the load (load dial) when the load held constant for at least four deformation reading and when the deformation passed 15% strain.
Microanalysis
Scanning Electron Microscopy
Scanning Electron Microscopy (SEM) analysis was carried out to identify the morphological features of the natural and treated soil. The test was carried out with a Phenom World Pro desktop scanning electron microscope that was equipped with a software application that could automate data collection and image interpretation such as particle-metric, elemental identification (automated image mapping) and 3D roughness reconstruction. The sample was placed in a motorized tilt and rotation sample holder and controlled by a dedicated motion control pro suite application which initiates
an endless 360o compucentric rotation with a pseudo- eucentric tilting adapted focus ranging from 10o to 45o. The motorized tilt and rotation sample holder allows analysis of the sample from all visible sides and enables a unique 3D image of the sample.
RESULT AND DISSCUSSION
Index Properties of the Soil sample
The properties of the lateritic soil type used in this study is summarized in Table1. The lateritic soil belongs to the SM Group in the Unified Soil Classification System [13] or A-2-4
Table 1: Properties of the Lateritic soil |
|
Property |
Quantity |
Percentage pass BS No. 200 sieve (75 µ) |
0.06 |
Natural Moisture Content (%) |
11.78 |
Liquid Limit (%) |
40.3 |
Plastic Limit (%) |
35.1 |
Plasticity Index (%) |
5.2 |
Specific Gravity |
2.58 |
AASHTO classification |
A-2-4(7) |
Maximum Dry Density (Kg/m3) |
1700 |
Optimum Moisture Content (%) |
16.5 |
Average Bulk Density (Kg/m3) |
1873.1 |
Organic Matter Content (%0 |
3.71 |
Colour |
Reddish brown |
Dominant Clay Mineral |
Muscovite |
pH |
4.65 |
(7) soil group of the AASHTO soil classification system [14]. The soil particle size range defined by coefficient of uniformity Cu is 13.36, while its coefficient of gradation Cg is 0.6819. Thus the soil is gap-graded with an effective size D10 of 0.137689 [15]. The particle size distribution curve is presented in the figure 1. The liquid and plastic limits of the soil are 40.3 % and 35.1 % respectively. It is classified as low plastic since its plasticity index is 5.2, a value which is less than 7 [16]. The organic matter content of the soil is 3.71 % which is greater than the permissible value of less than 2 % for engineering soil as suggested by [17]. The presence of organic matter in soil may affect its quality and engineering behavior. High organic content increases soil permeability and reduces the strength of the soil [17].
Fig 1: Particle distribution size of the lateritic soil used.
X-ray Diffraction
The X-ray powder diffraction of the untreated lateritic soil was taken to determine the minerals present in the lateritic soil sample. The interplanar spacing i.e. d-values of the dominant minerals at different braggs angles (2 Theta positions) corresponding to its peak intensity counts were determined using Instanano. The X-ray wavelength employed was 0.15418 angstorms. Fig 2 shows the diffractograph for the soil. The dominant minerals are Quartz, Muscovite, Kaoline and Geothite. This result is in agreement with the previous findings reported by [18] [19]. Quartz and Muscovite have highest peak intensity count at Braggs angle (2Theta position) of 27 degree with corresponding d-value of
0.33 nm (3.3 angstorms). The maximum peak for Kaoline occurs at 2Theta position 12.5 degree with corresponding d- value of 0.71 nm (7.1 angstorms) , while the maximum intensity count for Geothite occur at 2 Theta position 34 degree with d-value of 0.26 nm (2.6 angstorms).
Figure 2: Diffractograph of the lateritic soil sample used.
Unconfined Compressive Strength
For uncured specimens, a steady increase in strength in the first 14 days from 240.9 kPa to 411.6 kPa was recorded, until a strength decrease was observed at 21 day. Meanwhile, at age 28 days, the strength picked up to 332.4 kPa as shown in figure 3. Thus the peak strength of 411.6 kPa was attained at the age 14 days while the highest percentage strength increase of 95.23 % was achieved at age 7 days, when compared with the control specimen of the same age table 2. But for cured specimen, there was continual increase in strength in the first 14 days curing age from 411.6 kPa to
482.1 kPa after which it dropped to 480.2 kPa at 21 days curing age. Although it sharply increased to 497.9 kPa at 28 days. The peak strength attained for cured specimens is 497.9 kPa, obtained at 28 days of curing. Meanwhile, the highest percentage strength increase of 138.33 % was recorded at the 3 days of curing age in comparison with the control specimen CON of the same age. The increase in percentage of UCS
values as result of treatment with Bacillus mycoides_MTO47265 is in line with the findings of [20],[3].>
Figure 3: Unconfined Compressive Strength (kPa) for uncured and cured specimen over Age (days)
Table 2: Percentage Increase in Unconfined Compressive Strength (kPa) for Uncured and Cured samples over Age (days)
Age / Specimen |
% increase of cured specimen |
% increase of uncured specimen |
3 |
138.33 |
39.49 |
7 |
129.59 |
95.23 |
14 |
64.71 |
40.62 |
21 |
96.00 |
28.57 |
28 |
77.82 |
31.21 |
The Coefficients of permeability of Uncured and Cured Specimens
For uncured treated specimens, continual decrease in permeability coefficient from 0.000000136 cm/s to 0.000000112 cm/s was observed over the first consecutive 28 days of age. The highest percentage reduction in permeability coefficient of 89.43% was obtained 28 days when juxtaposed with the control specimen of the same age figure 4. A significant decrease in the coefficient of permeability was recorded from 0.000000617 cm/s to 0.000000558 cm/s from the curing age of 3 days and 7 days respectively but a sharp increase to 0.000000612 cm/s was attained at curing age 14 days figure. This remained constant till 21 days after which the value steeply dropped to 0.000000605 cm/s at the curing age 28 days. The maximum percentage decrease in the coefficient of permeability of 42.92 % was achieved on 28
days of curing age in measure with the value obtained for control specimen of the same curing age of 28 days
Figure 4: Coefficient of Permeability (cm/s) for Uncured and Cured samples over
Table 3: Percentage Decreasee in Coefficient of Permeability (cm/s) for both Cured and Uncured Treated specimen over Age (days)
Age /Specimen |
% decrease of cured specimen |
% decrease of uncurred specimen |
3 |
-35.46 |
-87.70 |
7 |
-40.30 |
-40.30 |
14 |
-30.14 |
-30.14 |
21 |
-38.80 |
-38.80 |
28 |
-42.92 |
-42.92 |
The SEM micrographs of the lateritc soil treated with Bacillus mycoides_MTO47265 and untreated (CON) is shown in plate 1 and 2 respectively. The bio-cemented (the specimen treated with specimen Bacillus mycoides_MTO47265) shows fully clustered crystals morphology of calcite cementing larger portion of the pores of the soil matrix when compared with that of non-bio- cemented specimen. This is in line with the claim of [21], [22] [3]. The formation of cementitious calcite precipitate coating the soil particles and clogging the inter-grain pores be could responsible for the improved strength and reduction in permeability of the Bacillus mycoides_MTO47265 cemented lateritic soil.
Plate 1a & 1b: SEM of treated and untreated lateritic soil specimen
Energy Dispersive Spectroscopy (EDS)
The EDS tests were conducted on the control specimens (untreated) and on the Bacillus mycoides_MTO47265 treated specimens as to determine the elemental compositions in term of atomic concentration. The atomic concentrations are presented in the table 4 below. From table below,the increase in atomic concentration of calcium and carbon,after MICP treatment of the soil, was found to be 0.32 and 1.02 respectively.When expressed in percentage,the increase in calcium and carbon was 64 % and 174 % respectively.This increase was due to formation of calcium carbonate in the inter-particle pores and on the surface of the soil matrix.
Table 4: Atomic Concentration of the Elements in the lateritic soil treated with Bacillus mycoides_MTO47265 |
|||
Element |
Untreated |
Treated |
Percentage Increase/Decrease |
Ca |
0.50 |
0.82 |
64.00 |
C |
0.60 |
1.62 |
170.00 |
O |
15.55 |
14.38 |
-7.50 |
Si |
35.58 |
41.55 |
16.77 |
Al |
25.91 |
24.38 |
-5.91 |
Fe |
15.55 |
13.29 |
-14.53 |
CONCLUSION
The lateritic soil treated with Bacillus mycoides_MTO47265, increased strength and reduction in permeability compared with that of untreated lateritic soil. Hence Bacillus mycoides_MTO47265 has the capacity of producing calcite.
REFERENCES
[1] A., Smith, M. Pritchard, A. Edmondson and Shafakat Bashir,. The reduction of the permeability of lateritic soil through the application of microbially induced calcite precipitate. Natural Resources, 2017, 8: 337-352. [2] J.,De Jong, K., Soga,, S. Burns, L. Van Paassen,, A. Qabany, A. Aydilek,, Biogeochemical Processes and Geotechnical Applications: Progress, Opportunities and Challenges.Géotechnique, 2013, 63.4:287301
[3] A. Eberemu. O., Kolawole J. Osinubi,, Stephen T. Ijimdiya, S. E. Yakubu., John E. Sani. Potential use of B. Pumilus in Microbioal Induced Calcite Precipitation Improvement of Lateritic Soil Proceedings of the 2nd Symposium on Coupled Phenomena in Environmental Geotechnics (CPEG2), Leeds, UK , 2017 [4] Junhui Zhang, Xiuzhi Shi, Xin Chen, Xiaofeng Huo, and Zhi Yu.. Microbial-Induced Carbonate Precipitation: A Review on Influencing Factors and Applications. Advances in Civil Engineering.Volume, 2021 |ArticleID 9974027 | https://doi.org/10.1155/2021/9974027
[5] DeJong, J. T., Mortensen, B. M., Martinez, B. C., and Nelson, D.C. Bio-mediated soil improvement. Ecol. Eng., 2011, 36(2), 197210.
[6] Gat,D., Tsesarky , M., Shamir, D., and Ronen, Z. Accelerated microbial induced CaCO3 precipitate I a defined coculture of ureoltic and non-ureolytic bacteria. Biogeosciences , 2014, 11: 2561-2569 [7] Fauriel, S. and Laloui, L.. A Bio-hemo-hydro-mechanical Model for Microbially Induced Calcite Precipitation in Soils. Q17 Computer. Geotechonology. 2012, (46) 104120 [8] Lai Hanjiang, Shifan Wu, Mingjuan Cui, Jian Chu. Recent development in biogeotechnology and its engineering applications.Front. Struct. Civ. Eng. 2021,15(5): 10731096 https://doi.org/10.1007/s11709-021-0758-0) [9] Harkes, M.P., van Paassen, L.A., Booster, J.L., Whiffi, V.S. and van Loosdrecht, M.C.M . Fixation and distribution of bacterial activity in sand to induce carbonate precipitation for ground reinforcement, Ecol. Eng., 2010. 36, 112-117 [10] Bernardi, D., DeJong, J. T., Montoya, B. M. and Martinez, B. C.. Bio-bricks: Biologically Cemented Sandstone Bricks. Construction Building Material 2014, 55.31:462469. [11] Van Paassen, L. A., Ghose, R., van der Linden, T. J. M., van der Star, W. R. L. and van Loosdrecht, M. C. M.. Quantifying Biomediated Ground Improvement by Ureolysis: Large Scale Biogrout Experiment. Journal of Geotechnology and Geoenvironmental Engineering 2010, 136.12:17211728. [12] Cheng, L. and Cord-Ruwisch, R.. Upscaling Effects of Soil Improvement by Microbially Induced Calcite Precipitation by Surface Percolation. Geomicrobiology Journal, 2014, 31.5:396406.
[13] ASTM Annual Book of Standards Vol. 04.08, American Society for Testing and Materials, Philadelphia. 1992. [14] AASHTO Standard Specifications for Transport Materials and Methods of Sampling and Testing.14th Edition, American Association of State Highway and Transport Officials (AASHTO), Washington,D.C, 1986. [15] Arora, K.R. (2009) Soil mechanics and Foundation Engineering (Geotechnical Engineerng) 7th edition page 58-60. 2009, New Delhi. . [16] Rammurthy and Sitharam, 2014 Geotechnical Engineering (Soil Mechanics) S. Chand and Company PVT. LTD. Revised Edition New Delhi Page, 2014. [17] Al Rawi Orabi, Assaf, N Mohammed and Rawashdeh Taiseer. Effect of Organic Content on the Engineering Properties of Jordanian Clayey Soils. International Journal of civil Engineering and Technology: 2017, 8 (11) 1018-1026. [18] Tsozue,D., Bitom, D. and Yogue-Fouateu, R.. Morphology, mineralogy and geochemistry of a lateritic sequence developed on micaschrist in the Abong-Bang region, Southeast Cameroon.Geological Soc S. Afr, 2012, 115:103-116. [19] Brice T Kamtchueng, Vincent L Onana, Wilson Y. Fantong, Akira Ueda, Roger F.D. Ntouala , Michel H.D. Wongolo, Ghislain B. Ndongo, Arnaud Ngoo Ze, Véronique K.B. Kamgang and Joseph M. Ondoa, Geotechnical, chemical and mineralogical evaluation of lateritic soils in humid tropical area (Mfou, Central-Cameroon): Implications for road construction International Journal of Geo-Engineering 2015, 6(1):1-21. [20] Putra H, Yasuhara H, Kinoshita, N. Neupane, D and Lu C-W. Effect of Magnesium as Substitute Material in Enyzme-Mediated Calcite Precipitation for Soil- improvement Technique. Front Bioeng Biotechnol. 2016, 4:37. [21] Wei-Soon Ng, Min-Lee L., and Siew-Ling H .An Overview of the Factors Affecting Microbial-Induced Calcite Precipitation and its Potential Application in Soil Improvement. International Journal of Civil, Environmental, Structural, Construction and Architectural Engineering 2012, .Vol: 6, No: 2, Pp. 188. [22] Hanifi, C., Waleed, S. and Ibrahim, H. K.Bacterial Calcium Carbonate Precipitation in Peat. Arab J Sci Eng, 2015, 40:2251 2260DOI10.1007/s13369-015-1760-4.