
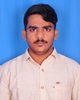
- Open Access
- Authors : N R N Prem Kumar
- Paper ID : IJERTV11IS040228
- Volume & Issue : Volume 11, Issue 04 (April 2022)
- Published (First Online): 03-05-2022
- ISSN (Online) : 2278-0181
- Publisher Name : IJERT
- License:
This work is licensed under a Creative Commons Attribution 4.0 International License
Improvement of Black Cotton Soil for Pavement Sub Grade Using Calcium Carbide Residue and Flyash
N R N Prem Kumar
Assistant professor Department of Civil Engineering
Rajiv Gandhi University of Knowledge Technologies-AP
Abstract: Locally available soils are readily available and comparatively cheap, but theyre often problematic and dont satisfy the specified geotechnical properties. In developing countries like India, needs for infrastructural and industrial projects are increasing rapidly. These require soils which have high bearing capacity and strength properties and also are available locally. Black cotton soil is one of such locally available soils which constitute about one-fifth part in India. In case of black cotton soil, their volume change and bearing capacity which is low when compared to other soils are the major problems faced by a geotechnical engineer. In such situations, soil improvement or modification plays a major role. Stabilization is the common method implemented to improve these properties. Stabilization of soils using industrial wastes is widely recommended for countries that are developing in the construction of pavements. Rapid industrialization also causes production of giant amount of solid waste materials whose disposal creating lot of environmental problems. Calcium carbide residue (CCR) comes from acetylene gas production and Fly Ash (FA) comes from power plant production. Both CCR and FA are abundantly available as waste materials. The application of CCR is very limited in civil engineering works, especially in soil stabilization. Thus, the current work is carried
out to estimate the optimal C C R c o n t e n t a n d CCRFA ratio from different binder contents for improvement of black
cotton soil as pavement subgrade material.
In order to obtain the optimum content of CCR and CCR:FA blender Standard Proctor compaction, unconfined compression test (UCC), California bearing ratio (CBR) test, Mineralogical and Morphological studies are conducted. The UCC samples are tested under different curing periods (3, 7, 14 and 28 days) and CBR test is carried out for a curing period of 1 day. Mineralogical and morphological studies are carried on samples cured for 28 days.
From this study, its revealed that for mixes of BC Soil + 8% CCR and BC Soil + 10% CCR + 6% FA are considered as optimum to enhance the black cotton soil for pavement subgrade.
Keywords: Soil stabilization, Expansive soils, Calcium carbide residue, Fly ash.
INTRODUCTION:
Expansive soils are posing huge problems and many challenges to civil engineers, construction firms and owners. The black cotton soils in India are well known for their expansive nature. In India, the black cotton soil covers 7 lakh square kilometres approximately 20-25 % land area and are found in the states of Maharashtra, Gujarat, Rajasthan, Madhya Pradesh, Uttar Pradesh, Karnataka, Andhra Pradesh and Tamil Nadu. These are derived from the weathering action of Basalts and traps of Deccan plateau. However, their occurrence on granite gneiss, shales, sandstones, slates and limestone is additionally recognised (Uppal, 1965; Mohan, 1973; Katti, 1979; Desai, 1985).
They are extremely fertile for agricultural functions however create severe issues to the pavements, embankments and light-weight to medium loaded residential buildings resting on because of cyclic volumetric changes caused by moisture fluctuation. Cracks appear in the overlying structures due to this volume change behaviour of black cotton soils. The reason for this behaviour is due to presence of clay mineral such as montmorillonite that has an expanding lattice structure. During monsoons, soils containing this mineral will imbibe water, swell, become soft and their capacity to bear water is reduced, while in drier seasons, these soils shrinks and become harder due to evaporation of water. These soils are majorly found in semi-arid and arid regions.
These types of soils are to be stabilized in order to rectify its deficiencies in engineering properties especially to use as pavement material. Pavement design is based on the preface that minimum specified structural tone will be achieved for each layer of material in the pavement system. Each layer should be able to resist shearing, avoid excessive deformations that cause fatigue cracking within the same layer or in overlying layers, and stop excessive permanent deflection through densification. As the quality of a soil layer is enhanced, the ability of that layer to spread the load over a greater area is generally increased so that a reduction in the required thickness of the soil sub-grade and surface layers may be allowed.
-
Calcium Carbide Residue:
Figure.1: Regions covered by Black cotton soil in India
It is a by-product from the acetylene gas production. It is used around the world for welding, metal cutting, lighting and to fruit ripen. This calcium carbide residue is obtained from a reaction between Calcium carbide and water which produce acetylene gas and calcium hydroxide in a slurry form which mainly consists of calcium hydroxide Ca(OH)2 along with SiO2, CaCO3 and other metal oxides. In India, there are many Acetylene Gas factories and PVC Chemical Plants which produces CCR in huge quantities mainly dumped in the landfills causing environmental pollutions because of its alkalinity. CCR production is described in the following equation:
CaC2+ 2H2O C2H2+ Ca (OH)2
For large content of natural pozzolanic materials in clayey soils, calcium hydroxide [Ca(OH)2] can be used to produce high strength geo-material. For the environmental and economic impact such waste rich materials can be utilized collectively with natural pozzolanic material in clay to invent a cementitious material.
-
Fly Ash:
Fly ash is a major concern to the remediation experts because fly ash contains trace levels of heavy metals and other toxic substances which will pose human health hazard. According to Fly Ash Utilization (FAU) total fly ash generated in India during the period of 2010-12 is estimated to be 131.09 million-tonne out of which 73.13 million-tonne (approximately 55.79% of total fly ash) is utilized for various purposes. Although usage of fly ash is continuing to grow day by day, but still lot of efforts are required to consume the remaining quantity of fly ash which is being left over.
Objectives of the Project:
-
To study the influence of calcium carbide residue and fly ash on Black cotton soil.
-
To compare unconfined compressive strength and California bearing ratio values of soil treated with CCR + FA and untreated black cotton soil.
-
To understand the mineralogical and morphological variations of soil treated with CCR and fly ash binder.
-
To formulate a model for optimum utilization of CCR and FA with 10% CCR to obtain particular value of CBR for BC soil using Multi-Linear Regression Analysis and SOLVER an add-in of Microsoft Excel.
LITERATURE:
Since past, mankind has used the natural earth surface for transportation. These road surfaces were transformed in to masses of mud by the spring and rain, where as in the summer season the carts created clouds of dust. Many attempts were made to take care of these problems. Some researchers tried to modify the engineering properties of soil using certain stabilization technique. At an equivalent time various waste products are created by several industrial plants. These waste products might be utilized in the construction projects after following certain treatment procedure. By treatig natural soil or fly-ash, or by addition of certain materials thereto, new construction materials are often developed. One such natural soil that covers many parts of India is black cotton soil. The main problem with this soil is their expansive nature.
Expansive soils, which usually contain the clay mineral montmorillonite and also include sedimentary and residual soils, clay stones and shales. The volume change can exert enough force on a building or other structure to cause damage to them. Expansive soils also will shrink once they dry out. This shrinkage can remove support from buildings or other structures and end in damaging subsidence and fissures. Geotechnical engineers did not recognize the damages related to buildings on expansive soils until late 1930s. The U.S. Bureau of reclamation made the primary observation which is recorded about soil heaving in 1938 (Chen, 1975). Since then variety of researchers have pioneered researches into expansive soils. The expansive nature of a soil is most obvious near the ground surface where the profile is subjected to seasonal and environmental changes (Terzaghi, Peck and Mesri, 1996; Fredlund and Rahardjo, 1993).
The term clay can refer both to a size and to a category of minerals. As a size term, it refers to all or any constituents of a soil smaller than a specific size, usually 0.002 mm in engineering classifications. As a mineral, it refers to specific clay minerals which are distinguished by (1) small particle size, (2) net electrical charge, (3) plasticity of soil when mixed with water and (4) high weathering resistance (Mitchell and Soga, 2005).
Criteria to identify and characterize Expansive Soils:
Table 1: Soil expansivity predicted by plasticity index
Swelling Potential |
Plasticity Index (%) |
||
Chen |
IS:1498 |
Holtz and Gibbs |
|
Low |
0-15 |
<12 |
<20 |
Medium |
10-35 |
12-23 |
12-34 |
High |
20-55 |
23-32 |
23-45 |
Very high |
>35 |
>32 |
>32 |
Table 2: Soil expansivity prediction by liquid limit
Degree of expansion |
Liquid Limit WL (%) |
|
Chen |
IS: 1498 |
|
Low |
<30 |
20-35 |
Medium |
30-40 |
35-50 |
High |
40-60 |
50-70 |
Very High |
>60 |
70-90 |
Table 3: Soil expansivity prediction by Shrinkage Limit
Degree of Expansion |
Shrinkage Limit WL (%) |
|
Holtz and Gibbs |
IS:1498 |
|
Low |
>15 |
>13 |
Medium |
10-16 |
8-18 |
High |
7-12 |
6-12 |
Very high |
<11 |
<10 |
Factors affecting volume change:
Table 4: Soil factors that influence shrink-swell potential (Nelson and Miller.1992)
Factor |
Description |
Clay mineralogy |
Montmorillonites, Vermiculites, and some mixed layer minerals undergo considerable soil volume changes. |
Soil water chemistry |
Swelling is repressed by increased cation concentration and increased cation valence. |
Soil suction |
Soil suction is an independent effective stress variable, represented by the negative pore pressure in unsaturated soils. Soil suction depends on saturation, gravity, pore size and shape, surface tension, electrical and chemical characteristics of the soil particles and water. |
Plasticity |
Soils that exhibit plastic behaviour over wide ranges of moisture content and have high liquid limits exhibit greater potential for swelling and shrinkage. |
Soil structure and fabric |
Flocculated clays tend to be more expansive than dispersed clays. Cemented particles reduce swell. Fabric and structure are altered by compaction. |
Dry density |
Higher densities usually indicate closer particle spacing, which may mean greater repulsive forces between particles and larger swelling potential. |
Table 5: Environmental factors that influence shrink and swell potentials of soils (Nelson and Miller.1992)
Factor |
Description |
Moisture variations |
Changes in moisture in the active zone near the upper part of the soil profile primarily define heave. It is in those layers that the widest variation in moisture and volume change will occur. |
Climate |
Amount and variation of precipitation and evapotranspiration greatly influence the moisture availability and depth of seasonal moisture fluctuation. Greatest seasonal heave occurs in semiarid climates that have pronounced short wet periods. |
Groundwater |
Shallow water tables provide a source of moisture and fluctuating water tables contribute to moisture. |
Drainage and manmade water sources |
Surface drainage features provide sources of water at the surface; leaky plumbing can give the soil access to water at greater depth. |
Vegetation |
Trees, shrubs, and grasses deplete moisture from the soil through transpiration and cause the soil to be differentially wetted in areas of varying vegetation. |
Permeability |
Soils with higher permeability allow faster migration of water and promote faster rates of swell. |
Temperature |
Increasing temperature cause moisture to diffuse to cooler areas beneath pavements and buildings |
Stress history |
An over-consolidated soil is more expansive than the same soil at the same void ratio, but normally consolidated. |
Loading |
Magnitude of surcharge load determines the amount of volume change that will occur for a given moisture content and density. An externally applied load acts to balance inter- particle repulsive forces and reduces swell. |
Remedial Measures to Expansive Soil for Pavement Subgrade:
-
Soil Replacement: It consists of removing the expansive soil completely or to a considerable depth and backfilling it with granular materials ( Zeitlen, 1969; Chaturvedi, 1977; Ordemir et al., 1977; Snethen et al., 1979; Chen, 1988). This meth od is practicable only if expansive soil extends to a shallow depth and granular material is obtainable in abundance within the vicinity of construction. If partial replacement is to be done, care should be taken to restrict the entry of surface drainage into the underlying expansive soil.
-
Application of Surcharge Pressure: In application of surcharge pressure, the expansive soil is loaded with a pressure equal to or greater than the swell pressure to counteract swelling. However, this method is often adopted in low swelling clays and in structures where adequate superimposed loads are exerted on the foundation. Chen (1988) claims that at a comparatively shallow depth beneath the foundation, the intensity of added stress is very small and swelling may occur beneath this level.
-
Pre-wetting: The principle of pre-wetting relies on the concept that if soil is allowed to swell by wetting before construction and if the high soil moisture content is maintained, the soil volume will remain essentially constant, achieving a non- heave state; therefore, structural damage won't occur. Pre-wetting is generally done by method of ponding. Some of the applications of this method which are successful and unsuccessful are reported (Mc Dowell, 1965; Blight and Wet, 1965; Williams, 1965; Bara, 1969; Gromco, 1974; Datye, 1977; Subba Rao and Satyadas, 1980) and it is generally felt that a great deal of time is required to stabilise the specified thickness of highly impermeable expansive soil bed (Snethen, 1979; Chen, 1988). To quicken the process of pre-wetting and to facilitate wetting with depth, a grid of boreholes are often installed before ponding (Blight and Wet, 1965; Subba Rao, 1986).
It has also been felt that it's possible to vary the swelling characteristics of expansive soils by means of flooding a given site with proper choice of electrolyte solution (Katti, 1966; Ho, 1968; Frydman, 1977; Subba Rao, 1994).
-
CNS-Layer Technique: This method is developed by Katti (1969). Field observations by Katti and his colleagues indicated that within the case of swelling soils, the swelling phenomenon is confined only to a small depth, 1.0 to 1.5 m below the surface of the expansive soil and by its own can develop cohesion that is sufficient to counteract swelling pressure. Upon observation, it's found that if an environment almost like that existing at 1 m depth within the black cotton soil with equivalent cohesion is produced and at an adequate time the system utilized in producing this environment doesn't swell or exert swelling pressure, then it's possible to counteract the swelling pressure and heave of a swelling soil mass.
Hence, it has been proposed to interpose adequate thickness of cohesive non-swelling layer between the structures and so the swelling soil base. When such a soil layer comes into contact with black cotton soil before saturation, it develops an electrical environment at the interface and below. This environment and thus the load of the CNS together helps in developing absorbed water bonds during saturation within the black cotton soil system. Further, due to development of cohesive forces by CNS and to some extent its weight, it reduces the void ratio of the expansive soil at the interface, thereby increasing the expansive soil cohesion, although the cohesion of CNS which developed in swelling soil at the interface needn't necessarily be the same. Under the influence of particular minimum thickness of CNS layer, the void ratio and therefore the consequent increase within the expansive soil cohesion reach the requisite limits needed for counteracting the swelling pressure of the soil.
-
Geosynthetics: Das (2006) believes that geosynthetics (including geofabrics, geotextiles and geomembranes) play a task in separating materials, reinforcing, filtering, draining and/or providing a moisture barrier. To be more descript – keeping a clay subgrade and sub-base material separate, increasing load bearing capacity, protection of fine-grained soils from transportation and channelling undesirable water away. Zornberg and Gupta (2009) support this and state that geosynthetics reinforce the subgrade or base materials by providing lateral restraint (minimising spread), tensile membrane support and increasing the bearing capacity. These benefits are supported by field observations of the traffic benefit ratio (TBR) and so the base course reduction (BCR).
Crone (2009) demonstrates that an enormous cost saving can be made by replacing an unbound layer with a geogrid. Zornberg and Gupta (2009) have concluded that the absence of geofabric incorporation in design manuals are due to lack of understanding and actual testing on the contribution that these fabrics are delivering to improved performance of pavements. Kwon, Tutumluer and Al-Qadi (2009) present a similar argument, stating cost benefit analyses haven't yet been realised and an adequate design procedure is unavailable.
In 2008, Zornberg et al. (2008) evaluated and reported in their findings of 35 projects over swelling clays within the USA (Forth Worth to Dallas) which were experiencing cracking. They found that 26 out of the 35 projects had been constructed using geosynthetics (with bi-axial grids the preference) which do not have well-defined design procedures.
-
Moisture Barriers: As entitled, moisture barriers around a foundation or pavement can assist in controlling the movement of water causing differential heave. This may also include a geosynthetic material or plastic layer that lines the pavement box to contain the pavement materials. Of all the research carried out to do this review, there was little mention about moisture barriers and no elaboration on successful methods.
-
Stabilization Methods: As expansive soil movements cause significant damage to the infrastructure built above them. Hence, to mitigate expansive soil movements, several methods were investigated. These methods are divided into three groups (Ingles and Metcalf, 1972; Mitchell and Katti, 1981).
-
Physical stabilization: Modification of soil properties by heat and electricity.
-
Mechanical stabilization: Improvement of soil by mixing materials that do not alter any soil properties.
-
Chemical stabilization: Modification of soil properties by using solid or liquid chemical additives.
Materials:
EXPERIMENTATION AND METHODOLOGY:
-
Black-cotton soil: Black-cotton soil (BC soil) has been obtained from the site besides the sewage tank located in the staff quarters. The soil has been collected from a depth of 1.0m below ground level. According to IS soil classification system, this soil was classified as high plasticity clay (CH). The elemental chemical composition and physical properties of Black cotton soil are shown in table 6, 7 respectively. The results of standard proctor test shows that optimum moisture content was 16.6% corresponding to a maximum dry density of 1.70g/cc.
Figure 2: SEM image of BC soil
Table 6: Elemental chemical composition of BC soil (%)
Constituent
CCR
Silica (SiO2) Alumina (Al2O3) Ferrous (Fe2O3) Calcium (CaO) Titanium (TiO2) Magnesium (MgO) Sodium (Na) Potassium (K)
79.93%
9.59%
5.07%
1.05%
0.54%
2.11%
0.60%
1.11%
Table 7: Properties of Black cotton soil
S.NO
Properties
BC Soil
1
Specific gravity
2.65
2
Grain size analysis Gravel (%) Sand (%)
Silt (%) Clay (%)
4
29
40
27
3
Liquid limit
59
4
Plastic limit
18
5
Plasticity index
41
6
IS Soil classification
CH
7
Compaction properties Optimum moisture content (%) Maximum dry density (g/cm3)
16.6
1.68
8
Unconfined compressive strength (kg/cm2)
2.01
-
Calcium Carbide Residue: Calcium carbide residue is a waste material that is collected from gas welding shop in Nuzvid. The specific gravity of Calcium carbide residue is 2.23. The elemental properties of CCR are shown in the table 8.
Figure 3: SEM image of Calcium carbide residue
Table 8: Elemental chemical composition of CCR (%)
Constituent
CCR
Silica (SiO2) Alumina (Al2O3) Ferrous (Fe2O3) Calcium (CaO) Titanium (TiO2) Magnesium (MgO)
40.7%
10.17%
8.04%
40%
0.65%
0.44%
-
Fly Ash: As per ASTM C 618 Fly ash was classified into CLASS F. Class F Fly ash is produced from combustion of bituminous coals or anthracite coals. It has low calcium (Cao) percentage about 20 % and silica + alumina + iron oxide more than 70%.
To find whether the fly ash brought from Ramagundam is CLASS F, Scanning Electroscope Microscope (SEM) and EDAX analysis were done and the results are as shown below.
Figure 4: SEM image of Fly Ash.
Table 9: Elemental chemical composition of Fly ash (%)
Constituent
Fly ash
Silica (SiO2) Alumina (Al2O3) Ferrous (Fe2O3) Calcium (CaO) Titanium (TiO2) Magnesium (MgO)
62%
10.17%
3.04%
0.92%
0.99%
0.44%
From the table 9, it is clear that percentage of calcium (CaO) is less than 20% and silica + alumina + iron oxide more than 70%. From this, Fly Ash is classified as class F.
Table 10: Properties of Fly ash
S.NO
Properties
Fly ash
1
Specific gravity
2.32
2
Grain size analysis Gravel (%) Sand (%) Fines (%)
0
6
94
3
Liquid limit
NP
4
Plastic limit
NP
5
Plasticity index
NP
6
IS Soil classification
CH
7
Compaction properties Optimum moisture content (%) Maximum dry density (g/cm3)
27.4
1.23
Tests used in this Investigation:
-
Specific gravity
-
Grain size analysis
-
Atterbergs limits
-
Standard Proctor compaction
-
Unconfined compressive strength
-
California bearing ratio
-
X-ray diffraction analysis
-
Scanning electron microscopy
-
-
-
Specific Gravity Test: It is the ratio of mass of unit volume of soil to the mass of same volume of distilled water which is gas- free at a particular temperature. This property of soil is used for deriving a relationship between air, solids and water in a given volume of soil. This test was carried out by using a Pycnometer as per IS 2720 Part 3 (1980).
-
Grain Size Analysis Test: This test provides the grain size distribution which is used to classify the soil. Grain size analysis tests are still some of the most valuable guides to the engineering behaviour of soils in the context of geotechnical engineering. Fine particle and coarse particle distributions are found out in this test using IS 2720 Part 4 (1985). It is followed for particles whose size are more than 75 micron only. Hydrometer analysis was carried out for particles of size less than 75micron.
-
Atterberg Limits: Liquid limit and plastic limit are termed as Atterberg limits. Liquid Limit (LL) is defined as that moisture content at which soil starts to behave as a liquid and starts to flow. Plastic Limit (PL) is defined as that moisture content at which soils starts to behave as a plastic material. Liquid limit and Plastic limit tests were done as per IS 2720 Part 5 (1985). Liquid limit tests were conducted using two different apparatus namely Casagrande apparatus and cone penetration apparatus.
Casagrande Method: Liquid limit of soil was determined with the help of standard apparatus designed by Casagrande. Take about 120g of soil sample which passes through 425-micron IS sieve and mix it thoroughly with distilled water in an evaporating dish or on a flat glass plate to form a uniform paste. The paste placed in the brass cup of Casagrande apparatus and the groove is cut with the standard grooving tool. The handle of the apparatus is rotated at a rate of 2 revolutions per second and number of blows were counted till bottom of the groove joins along a distance of 10 mm due to the flow and not by sliding. Take about 10 gms of this soil for determining water content. The process is repeated by taking different water contents.
Liquid Limit: A flow curve is plotted between water content and number of drops using a semi logarithmic curve taking water content on the arithmetical scale and number of drops on logarithmic scale. This flow curve is nearly a straight line which passes through four or more plotted points. The water content which corresponds to 25 drops which is read from the curve will be rounded off to the nearest number and taken as the liquid limit of the soil.
Figure 5: Casagrande apparatus for determination of consistency limit.
Plastic limit test: Plastic limit of clay was determined by taking the soil sample passing through 425 sieve which is mixed with water until the soil mass becomes plastic enough to be easily remoulded. A ball is formed using about 8 g of paste and rolled between fingers up to 3mm diameter and the sample is again remoulded into a ball. This process of rolling and remoulding is repeated until the thread starts just crumbling at a diameter of 3mm.The crumbling threads are kept for water content determination. The test is repeated two or three times and the average water content are taken which is treated as the plastic limit.
-
Standard Proctor Test: Compaction tests are still some of the most valuable guides to the engineering behaviour of soils in the context of road engineering. Standard Compaction Test was used to determine the OMC (optimum moisture content) and the maximum dry density to which various mixes can be compacted at this moisture content. Although these tests form a part of the procedures of other tests used, they were performed primarily to determine whether or not there was an increase in density upon the addition of various additives to the soil. Improvement of grading and/or compaction of soil to higher density results in reduction in settlement, reduction in permeability and an increase in shear strength.
Standard proctor compaction tests were conducted as per IS: 2720 Part 7 (1980) on various mixes prepared on basis of dry weight. The mould of standard volume equal to 1000cc is used. The mould is filled up with the material to be compacted by 25 blows in three layers. Standard hammer of 2.45kg weight falling from a height of 30cm is used for compaction. Test is repeated at different water contents. Dry density is calculated at all water contents so as to obtain the compaction curve between moisture content and dry unit weight. The water content which corresponds to maximum value of dry density is taken as the optimum moisture content.
-
California Bearing Ratio Test: It is a penetration test which measures the load spreading ability of the pavement and is only justified in the case of pavements that are flexible. The CBR tests were performed as per IS : 2720 Part 16.To prepare the samples for CBR test, different mixes chosen were compacted statically in standard moulds at optimum moisture content and maximum dry density. The dimension of the soil sample for CBR test is taken as 150mm diameter and 125mm height. Surcharge weight of 25N was used during the testing. A metal penetration plunger of diameter 50 mm and 100 mm long was used to penetrate the samples at the rate of 1.25 mm/minute using computerized CBR testing machine. After soaking for 96 hours, the soaked CBR tests were conducted. For soaking samples were placed in a tank maintaining constant water level throughout the period.
Figure 6: CBR testing machine
-
Unconfined Compressive Strength Test: The effect of the various additives on the strength of stabilized soil has little direct application to pavement design. Comressive strength test has been used to determine the relative response of materials to cement and lime stabilization and to give an overall picture of the quality of stabilized materials. It is generally assumed that the higher the compressive strength the better the quality of stabilized mixes. In this study the unconfined compressive strength tests were performed in accordance with test method IS 2720 Part10 (1991) to determine the effect of adding various additives, of different proportions and at various rates of application, to different soils. These tests were conducted on the reference mixes that are obtained from standard compaction test. The sizes of the samples prepared were of aspect ratio 2 i.e., 38 mm diameter and 76 mm length and the strain rate of 0.60 mm/minute is used for testing. The samples were prepared by compacted sample with the help of a tamping rod in three layers at optimum moisture content and maximum dry density in the UCS mould of standard dimension.
Figure 7: Unconfined compressive strength on sample
-
Powder X-ray Diffraction: Powder X-ray diffraction is a method widely used in the analysis of solid solution, crystallinity and, particularly, with small angle scattering, the size and, to some extent, the shape of small particles. The hydration products of lime-RHA and lime-GBFS stabilized materials were identified in this study using the diffraction technique.
Theory of X-Ray Diffraction: When a beam of monochromatic X-rays is directed onto a crystalline surface, diffraction occurs, the diffracted beam being built up of rays scattered by the atoms in the crystal lying in its path. The reinforcement of scattered rays occurs when Bragg's law is satisfied.
n = 2d sin , Where
= wave length of the X-ray
d = crystal spacing characteristic of each mineral component = angle of incidence of the X-ray
n = integral number
By using X-rays of known wave length, and measuring the set of 6 which produced diffracted beams, d spacings of the various planes in a crystal can be calculated. In the powder diffraction method, the sample is reduced to a very fine powder and placed in the beam of X-rays. Each particle is therefore a crystal oriented randomly with respect to the beam. However, some particles must be oriented so that a particular set of lattice planes makes the correct Bragg angle for the beam diffraction. The presence of a large number of particles having all possible orientations ensures that the diffracted beams represent every set of lattice planes in the crystal. The diffracted beams are detected and their intensities and associated angles determined and recorded by a movable counter. The d spacings and their associated intensities form the pattern which is characteristic of the substance. Identification of a particular substance is made with the aid of standard tables of crystal reflections and their intensities.
-
Scanning Electron Microscope: Morphological studies are carried out using scanning electron microscope (VEGA 3 series) with tungsten heated filament having live stereoscopic imaging using 3D beam technology. Scanning electron microscopy (SEM) is conducted to understand the morphological changes occurring in the soil due to CCR and CCR+FA binder. The soil specimens were mounted on to the tape glued to the flat surface of SEM stub and sputter coated with gold prior to scanning.
Formulating a model: The objective function is formulated as follows:
Y is directly proportional to the variables X1, X2, X3 & X4. The equation created as given below. Y = b0 + b1X1 + b2X2 + b3X3 + b4X4 + b5X5
Where,
Y = California Bearing Ratio (%), b0, b1, b2, b3, b4 and b5 = constants, X1 = CCR or Fly Ash Fraction (%), X2 = Value of Liquid Limit (%),
X3 = Value of Plasticity Index (%),
X4 = Value of Optimum Moisture Content (%), & X5= Value of Maximum dry density (g/cc).
-
The values of above constants will be solved by using the Multi-Linear Regression Analysis in the Data Analysis Toolpak an add-in for Microsoft Excel.
-
After obtaining the objective function, using SOLVER an add-in for Microsoft Excel which is used to predict the amount of CCR, CCR+FA required to obtain the target value of CBR.
-
To check the reliability of equation, experimental values are compared with predicted values.
-
Input data required for SOLVER is experimental data, changing variables and constraints.
Figure 8: Setting target value, giving constraints and changing variable cells in SOLVER
Methodology: The experimentation program of the present work was conducted in three phases. Phase 1:
-
The first phase dealt with finding the optimum content of calcium carbide residue from Atterbergs limits and unconfined compressive strength, which involved addition of different percentages (4%, 6%, 8%, 10%, and 12%) of Calcium carbide residue to black cotton soil.
-
The CCR was thoroughly mixed by hand until homogeneity was reached, and the mixture was quickly stored in a large plastic bag to prevent losing of moisture content. All CCR-treated soil specimens were tested for unconfined compressive strength after curing time of 3, 7, 14 and 28 days.
-
Atterbergs limits test and soaked CBR test were carried out after 1 day curing period.
-
Although the optimum content of CCR obtained from experimental results is 8%, for phase 2 the percentage of CCR is fixed as 10% in order to get pozzolanic reaction between free lime in CCR and fly ash.
Phase 2:
-
-
The strength of the blended CCR and FA-stabilized black cotton soil was investigated with fixed CCR content of 10% with varying fly ash contents (2%, 4%, 6%, 8%, and 10%).
-
UCS test is carried out for curing periods of 3, 7, 14and 28 days, CBR tests are carried out for curing period of 1 day. Phase 3:
-
Mineralogical and morphological studies were carried out to understand the mechanism that is responsible for obtaining strength in CCR and CCR+FA stabilized soil.
-
Formulating a model based on regression and using SOLVER an Add-In for Microsoft Excel which can be used to predict the amount of CCR and CCR+FA added to BC soil for obtaining the target values of CBR.
-
RESULTS AND DISCUSSIONS:
-
Atterbergs Limits: The test results from figure 9 shows that LL and PI decreased whereas PL increased for BC Soil mixed with CCR. It is known by addition of CCR to BC Soil can, (1) reduce the thickness of the diffuse double layer, (2) cause flocculation of clay particles, and (3) increase the content of coarser particles by substituting finer soil particles with coarser particles. All these reasons together cause the decrease in LL and PI, and the increase in PL. Mitchell (1993) indicated that PI is a good indicator of swell potential, the lower PI is, and the lower swell potential will be. Addition of CCR to BC soil decreased the plasticity index of expansive soil significantly. This implies there is a significant reduction in swell potential by addition CCR to BC Soil.
Liquid limit
70
60
50
40
30
20
10
0
Plastic limit
Plasticity index
0 2
Calcium carbide residue (%)
10
12
4
6
8
Water content (%)
Figure 9: Variations of liquid limit, plastic limit and plasticity index with addition of calcium carbide residue to black cotton soil
Table 11: Variations of liquid limit, plastic limit and plasticity index with addition of 10%calcium carbide residue and different percentages of Fly ash to black cotton soil.
Composition
Liquid limit
Plastic limit
Plasticity index
BC+10 %CCR+2%FA
32
21
11
BC+10 %CCR+4%FA
31
21
10
BC+10 %CCR+6%FA
30
21
9
BC+10 %CCR+8%FA
30
22
8
BC+10 %CCR+10%FA
30
22
8
Even with the addition of fly ash to the CCR stabilized Black cotton soil, Liquid limit and plasticity index is decreasing while plastic limit is increasing but to the lesser extent. CCR itself is giving significant reduction to the expansive nature of black cotton soil.
-
Standard Proctor Test: The standard proctor tests were conducted by mixing different percentages of CCR and CCR- Fly ash to the Black cotton soil. BC soil is mixed with CCR by varying its percentage from 4% to 12% in increments of 2% and standard proctor tests were conducted on the mixes. After selecting suitable proportion of calcium carbide residue, fly ash content was mixed from 2% to 10% in increments of 2% and standard proctor tests were conducted on these mixes.
1.70
Dry density (g/cc)
1.65
1.60
1.55
1.50
1.45
1.40
1.35
13 16 19Water Con2t2ent (%)
BC SOIL BC+ 4%CCR BC+6%CCR BC+8%CCR BC+10%CCR BC+12%CCR
25 28
Figure 10: showing changes in OMC and MDD with addition of CCR to BC soil.
From figure 10, it observed that there is an increase in the optimum water content and decrease in maximum dry unit weight when CCR is added to BC Soil, this can be explained as follows: The decrease of the maximum dry unit weight by adding CCR to BC Soil is mainly due to its lower specific gravity and the immediate formation of cemented products. The pozzolanic reaction between BC soil and CCR is responsible for increase in OMC. As CCR content increases, the water sensitivity of CCR stabilized clay decreases i.e., causing only a minor change in dry density with large increase in water content.
Table 12: Variation of Optimum Moisture content and Maximum Dry Density with addition of 10% Calcium carbide residue and different percentages of Fly ash to BC soil
Composition
Optimum moisture content (%)
Maximum dry density (g/cc)
BC+10 %CCR+2%FA
22.28
1.5
BC+10 %CCR+4%FA
22.67
1.49
BC+10 %CCR+6%FA
23.44
1.47
BC+10 %CCR+8%FA
24.2
1.44
BC+10 %CCR+10%FA
24.5
1.4
The maximum dry density of CCR+FA blended BC soil showed slightly higher values than blended CCR-stabilized BC soil in the initial FA increments up to 6%. This is because FA has a slightly higher specific gravity than CCR and therefore causes an increase in the specific gravity of the binder (CCR+FA). The spherical shape of the FA is also the main contributor to the compaction efficiency. It helps soil particles and CCR particles to slip over each other and into a densely packed state. However, all the blended CCR-stabilized BC soil samples possess a lower maximum dry unit weight than the compacted Black cotton soil because of its lower specific gravity. With the addition of fly ash to the CCR stabilized BC soil the maximum dry density decreased. This is attributed to the low specific gravity and spherical shaped particles (which occupy more volume) of fly ash. The increase in optimum moisture is due to the fineness or more surface area of fly ash particles compared to the BC soil.
-
Unconfined Compression Test: The unconfined compressive strength tests were conducted on the optimum mixes which are selected from standard proctors compaction test. The stress-strain behaviour of different composites with corresponding curing periods is shown in the following figures.
9.00
8.00
STRESS (kg/cm2)
7.00
6.00
5.00
4.00
3.00
2.00
1.00
0.00
3 DAYS
BC
BC+ 4% CCR BC + 6% CCR BC+ 8% CCR BC+10 %CCR BC+12% CCR
0 1 ST
2 %) 3 4
RAIN(
Figure 11: Stress-Strain behaviour of BC soil treated with CCR for a curing period of 3 days
7 DAYS
14
12
STRESS (kg/cm2)
10
8
6
4
BC
BC+ 4% CCR BC + 6% CCR BC+ 8% CCR BC+10 %CCR BC+ 12% CCR
2
0
0.0 1.0 2.0 3.0 4.0 5.0
STRAIN(%)
Figure 12: Stress-Strain behaviour of BC soil treated with CCR for a curing period of 7 days
25 14 DAYS
STRESS (kg/cm2)
20
15
10
BC
BC+ 4% CCR BC+6% CCR BC+ 8% CCR BC+10 %CCR BC+ 12% CCR
5
0
0.0 1.0 2.0 3.0 4.0 5.0
STRAIN(%)
Figure 13: Stress-Strain behaviour of BC soil treated with CCR for a curing period of 14 days
35 28 DAYS
30
STRESS (kg/cm2)
BC
25 BC+ 4% CCR
20 BC+6% CCR
BC+ 8% CCR
15
BC+10 %CCR
10 BC+ 12%CCR
5
0
0.0 1.0 2.0 3.0 4.0 5.0
STRAIN(%)
10
12
16
10
30.9
19
13
31.1
20
31.4
20
12
14
11
13
8
28.3
18
12
UCS (kg/cm2)
Figure 14: Stress-Strain behaviour of BC soil treated with CCR for a curing period of 28 days
40
30
20
10
0
7
25.5
19
12
23.9
10%CCR+Fly ash
0
2
4
6
8
10
3 days
7 days
14 days
28 days
Figure 15: Unconfined compressive strength of BC soil treated with 10%CCR with different percentages of Fly ash for curing periods of 3,7,14 and 28 days respectively
Further the unconfined compressive strength tests were carried out with CCR+FA stabilized BC soil. The stress-strain behaviour of the stabilized soil samples for 10% CCR with different FA contents at the OMC are shown in Figs. 11,12,13 and 14
for 3, 7, 14 and 28 days curing periods respectively. The strength development in inert zone is completely dependent on the FA content. The strength increases sharply as the FA content increases up to the optimal value and then decreases. In the deterioration zone i.e., after optimum CCR content the strength of CCR stabilized clay is lower than that of the inert zone. The reduction in strength with increasing CCR content is caused by unsoundness due to the free lime content. Thus FA can improve this detrimental effect as indicated by the increase in strength with increasing FA content. However, the strength increase is gradual up to an optimal FA content at about 6%. The maximum unconfined compressive strength obtained for 10% CCR is about 25.45kg/cm2 while the BC soil stabilized with 10% CCR and 6% FA blender is 31.36 kg/cm2. Thus, the main objective to improve the strength of BC soil for utilizing it as subgrade material is achieved.
-
California Bearing Ratio Test:
CBR tests were conducted for Black cotton soil stabilized with different percentages of Calcium carbide residue. All the specimens are compacted at optimum moisture content and cured for a period of 1 day. The soaked CBR test was carried out after soaking the specimens for 4 days. A graph was plotted between load vs penetration in the figure 16, and table 13, showing the CBR% obtained for different percentages of calcium carbide residue by applying corrections according to IS: 2720 part 16.
From the results it was observed that the CBR value of soil-CCR mix increased significantly with increase in CCR content. The strength of CCR stabilized soil is attributed to the pozzolanic reaction between silica and alumina of BC soil and lime of CCR to form various types of cementing agents.
CBR
700
600
500
Load (kg)
>
400
300
200
100
BC SOIL
BC + 4% CCR BC + 6%CCR BC + 8% CCR BC + 10% CCR BC+ 12 % CCR
0
0 2.5 5 7.5 10 12.5
Penetration (mm)
CBR (%)
Figure 16: Load Vs Penetration graph for BC soil stabilized with different percentages of CCR
25
20
BC soil +CCR
21.27
18.52
22.56
23.25
15
11.58
0
Calcium carbide residue (%)
0 4 6 8 10
12
10
5
2.15
Figure 17: Variation of soaked CBR values for BC soil stabilized with different percentages.
Based on the results as shown in the above Fig 16 and Fig 17, BC soil was initially poor subgrade material with CBR of 2.15%, improved substantially with the addition of calcium carbide residue and became suitable for laying pavements. Even the mix having BC Soil+8%CCR, BC Soil+10%CCR and BC Soil+12%CCR are giving a soaked CBR value more than 20%, which is desirable for sub base material as per IRC specifications.
26.61
28.6
27.64
25.67
22.56
CBR (%)
In second phase, CBR tests were carried out for Black cotton soil stabilized with 10%CCR and different percentages of Fly ash. From the figure 18, it was observed that CBR value increasing up to 6% fly ash content thereafter it started decreasing. The improved CBR may be attributed to the presence of the free lime content in CCR which will react with silica and alumina of fly ash to cause pozzolanic reactions. With further increase in fly ash, CBR is decreasing because there is no free lime content to react with fly ash.
BC soil+10% CCR+ FA increments
31.5
35
30
25
20
15
10
5
0
0 2
4 Fly ash (%) 6
8
10
Figure 18: Soaked CBR values for BC soil stabilized with 10%CCR and fly ash of different percentages i.e., 2%, 4%, 6%, 8% and 10%
-
Mineralogical Studies: Mineralogical studies are conducted using PAN analytical X-pert powder diffractometer. Samples were scan from 6º (2) and 70º (2) angle using copper K alpha radiation at a scanning rate of 2 degrees per minute. The data obtained is analysed using X-pert high score plus software, to identify minerals by comparing the standard diffraction patterns of minerals.
Figure 19: X-ray diffraction patterns of BC soil and BC soil treated with different percentages of CCR
Figure 20: X-ray diffraction patterns of BC soil treated with 10% CCR and Fly ash of different percentages
The X-ray diffraction analysis is carried out on samples cured for a period of 28 days. Prior to testing all the samples were oven dried for a period of 24 hours. The oven dried samples were powdered and passed through 75 micron sieve. This powder is used for X-ray diffraction analysis. In X-ray diffraction pattern of soil with respect to water shows that the natural black cotton soil contains Volkonskoite (Peaks at 4.49, 2.56 and 1.50 [Ã…]) along with Quartz (Peaks at 3.34, 2.45, 1.81 and 1 .67 [Ã…]) and microcline (Peaks at 3.24, 2.90 and 1.98 [Ã…]) as their major minerals. The black cotton soil treated with 4% CCR showed peaks pertaining to Rankinite (peaks at 3.20, 3.03 and 2.13[Ã…]), a new mineral, which is a calcium silicate mineral. For BC soils
treated with 6% CCR and 8% CCR and showed peaks pertaining to Anorthite (peaks at 3.47, 3.18 and 2.90 [Ã…]),which is a calcium aluminium silicate mineral and the soil treated with 10% CCR showed peaks to Calcite (calcium carbonate) at 3.03, 2.28 and 1.87[Ã…] in addition to Anorthite. The BC soil treated with 10% CCR and fly ash samples showed Anorthite (peaks at 3.47, 3.18 and 2.90 [Ã…]) in addition to minerals that present in natural soil.
21.1 BC Soil
21.2 BC Soil+4% CCR
21.3 BC Soil+6% CCR
21.4 BC Soil+8% CCR
21.5 BC Soil+10% CCR
21.6 BC Soil+10% CCR+2% FA
21.7 BC Soil+10% CCR+4% FA 21.8 B
C Soil+10% CCR+6% FA
-
Morphological Studies: Scanning electron microscope images of the BC soil and soil treated with CCR CCR+FA blender are shown in fig 21. Natural BC soil exhibits a fairly flocculated type of microstructure. This is due to the fact that individual volkonskoite particles are delicately thin and curved flakes, which exist as domains bonded by high valence inter layer cations to act as one thicker unit.
21.9 BC Soil+10% CCR+8% FA
21.10 BC Soil+10% CCR+10% FA
Figure 21: SEM images of BC soil and BC soil stabilized with CCR and CCR+FA blender
In the presence of iron oxides might have added the flocculants nature to the BC soil. It can be observed from the figure 21 that the soil treated with CCR showed more flocculated structure and formation of some cementing agents around the soil particles after 28 days curing time. The flocculation of soil particles increased with increase in concentrations of CCR due to pozzolanic reaction. However, at 10% CCR the formation of needle like structures indicates the unreacted content of CCR added to the soil. The SEM images of soil treated with CCR+FA mix showed significant changes in morphology. At lower fly ash contents the formation of needle shaped particles indicates the unreacted CCR content which signifies the requirement of additional fly ash content for chemical reaction. From SEM images of soil treated with 10% CCR+6% FA it is observed the absence of needle like structure which confirms the complete utilization of CCR and fly ash. Thereafter the presence of spherical shaped particles highlights the excess amount of fly ash which leads to reduction in strength.
-
Formulating a model for optimum utilization of CCR and FA with 10% CCR to obtain the target value of CBR for BC soil:
The objective of this modelling is to obtain the amount of CCR and CCR+FA binder added to soil for obtaining particular target value of CBR. For this, an equation is formulated in terms of CBR using multi-linear regression analysis a built-in of Microsoft excel. The values of coefficients are obtained from regression analysis. Then using SOLVER (an add-in of excel) the amount of CCR and CCR+FA value are calculated for target CBR values. The parameters required for SOLVER are changing variables, constraints and target value. Tables 13 and 14, 15 and 16 are showing the comparisons between experimental values and predicted values.
Table 13: Data obtained from experiments conducted on CCR stabilized soil
CBR (%)
CCR (%)
Liquid limit (%)
Plasticity index (%)
Optimum moisture content (%)
Maximum dry density (%)
2.15
0
59
41
16.6
1.68
11.58
4
42
22
18.8
1.58
18.52
6
39
18
19.6
1.54
21.27
8
37
16
20.6
1.51
22.56
10
35
14
21.5
1.45
23.25
12
32
12
22.3
1.42
The values of coefficients using regression are obtained by giving input data as Table 14. The Objective function is
Y = 1330.702+ 69.95636X1 -32.7514X2 + 25.054767X3 118.044X4 + 914.3204X5
Table 15: Predicted values of CCR stabilized soil from Solver
CBR (%)
CCR (%)
Liquid limit (%)
Plasticity index (%)
Otimum moisture content (%)
Maximum dry density (%)
2.15
0
59.25
40.91
16.46
1.67
11.58
3.99
42.06
20.98
18.54
1.58
18.52
5.99
39.13
17.98
19.51
1.53
21.27
7.99
37.09
15.99
20.59
1.51
22.56
9.95
35.24
13.97
21.31
1.43
23.25
11.96
34.12
11.98
22.19
1.41
Table 16: Data obtained from experiments conducted on 10% CCR+ FA stabilized soil
CBR (%)
FA (%)
Liquid limit (%)
Plasticity index (%)
Optimum moisture content (%)
Maximum dry density (%)
22.56
0
35
12
21.5
1.45
26.61
2
32
11
22.28
1.5
28.6
4
31
10
22.67
1.49
31.5
6
30
9
23.44
1.47
27.64
8
30
8
24.2
1.44
25.67
10
30
8
24.5
1.4
The Objective function is
Y = 826.322 – 5.32892X1 9.28365X2 2.49556X3 4.13757X4 248.2285X5
Table 17: Predicted values of 10% CCR+ FA stabilized soil from Solver
CBR (%)
FA (%)
Liquid limit (%)
Plasticity index (%)
Optimum moisture content (%)
Maximum dry density (%)
22.56
0
35.15
11.5
21.02
1.46
26.61
2
32.04
11.01
22.01
1.50
28.6
4
31.02
10.01
22.51
1.49
31.5
6.01
30.35
9.01
23.09
1.46
27.64
8
30.03
8
24.01
1.44
25.67
10.01
30.08
8.01
24.02
1.41
CONCLUSIONS:
The following conclusions are drawn based on the laboratory studies carried out in this work.
-
The unconfined compressive strength and California bearing ratio of CCR treated BC soil improved substantially with increasing percentages of CCR content. The unconfined compressive strength and CBR of natural soil enhanced 10 – 15 times after CCR stabilization.
-
The natural black cotton soil stabilized with calcium carbide residue and fly ash mix also obtained 15-16 times strength enhancement in both UCS and CBR.
-
The X-ray diffraction analysis identified the formation of calcium silicate hydrates and calcium aluminium silicates which are cementing agents. The Scanning electron microscopy images evidenced the flocculation of particles and pozzolanic compounds.
-
The amount of CCR, FA for target value of CBR predicted from SOLVER is in good correlation with experimental data.
-
From this study, it is suggested to use 8% CCR or 10% CCR + 6% FA blender to improve the black cotton soil for pavement subgrade.
REFERENCES:
[1] Suksun Horpibulsuk, et al. (2013): Strength development in silty clay stabilized with calcium carbide residue and fly ash, Soils and Foundations 2013;53(4):477-486. [2] Bell F.G. (1996): Lime stabilization of clay minerals and soils, Eng Geol 42:223 237. [3] Jaturapitakkul, C., Roongreung, B., (2003): Cementing material from calcium carbide residue-rice husk ash, Journal of Materials in Civil Engineering, ASCE 15(5),470475. [4] Kampala, A., Horpibulsuk, S., (2013): Engineering properties of calcium carbide residue stabilized silty clay, Journal of Materials in Civil Engineering, ASCE 25(5), MT.1943-5533.0000618. [5] IS: 1498 (1970) Classification and Identification of soils for engineering purposes. [6] IS: 2720 part III/Sec I (1980) Determination of Specific gravity, Fine grained soils. [7] IS: 2720 part IV (1985) Grain Size analysis. [8] IS: 2720 part V (1985) Determination of Liquid & Plastic Limit. [9] IS: 2720 part XL (1977) Determination of Free Swell Index of Soils. [10] IS: 4332 part-III (1967) Test for determination of moisture content dry density relation for stabilized soil mixtures. [11] Consoli, N.C., Marques Prietto, P.D., Harb Carraro, J.A., and Heineck, K.S. (2001): Behavior of compacted soil-fly ash-carbide lime mixtures, J. Geotech. Geoenviron. Eng. 2001.127:774-782. [12] Fusheng Zha, Songyu Liu, Yanjun Du and Kerui Cui (2008): Behavior of expansive soils stabilized with fly ash, Nat Hazards (2008) 47:509- 523. [13] Songsuda Vichan, Runglawan Rachan (2013): Chemical stabilization of soft Bangkok clay using the blend of calcium carbide residue and biomass ash, Soils and Foundations 2013;53(2):272-281. [14] Du, Y.J., Zhang, Y.Y., Liu, S.Y. (2011): Investigation of strength and California bearing ratio properties of natural soils treated by calcium carbide residue, Geo-Frontiers 2011, ASCE. [15] Makaratat, N., Jaturapitakkul, C., and Laosamathikul, T. (2010): Effects of calcium carbide residue-Fly ash binder on mechanical properties of concrete, J.Mater.Civ.Eng.2010.22:1164-1170. [16] Jyoti S.Trivedi, et al. (2013): Optimum Utilization of Fly ash for stabilization of sub-grade soil using Genetic algorithm, Procedia Engineering 51 (2013) 250_258. [17] Kampala, A., Horpibulsuk, S., (2013): Engineering properties of recycled calcium carbide residue stabilized clay as fill and pavement materials, Construction and Building materials 46 (2013) 203-210. [18] Kumrawat, N., Ahirwar, S.K. (2014): Experimental study and analysis of Black cotton soil with CCR & BA, International journal of Engineering Sciences & Management, ISSN: 2277-5528. [19] Hongfang Sun, et al. (2015): Properties of chemically combusted calcium carbide residue and its influence on cement properties, Materials 2015, 8, 638-651. [20] Phetchuay, C., Horpibulsuk, S. (2014): Calcium carbide residue: Alkaline activator for clay-fly ash geopolymer, Construction and buildingmaterials 69 (2014) 285-294.
[21] Joel, Manasseh, and Edeh. (2013): Soil modification and stabilization potential of calcium carbide waste, Advanced materials research Vol 824 (2013) pp 29-36. [22] Neeraj Kumar Sharma, S.K. Swain, Umesh C. Sahoo. (2012): Stabilization of a Clayey soil with fly ash and lime, Geotech Geol Eng (20120 30:1197-1205. [23] Kumrawat, N., Ahirwar, S.K. (2014): Performance analysis of Black cotton soil treated with calcium carbide residue and stone dust, International Journal of Engineering Research and Science & Technology, ISSN 2319-5991, Vol. 3, No. 4. [24] Laxmikant Yadu, et al. (2011): Comparision of fly ash and rice husk ash stabilized black cotton soil, International Journal of Earth Sciences and Engineering, ISSN 0974-5904, Volum 04, No 06 SPL, October 2011, pp.42-45. [25] Mitchell, J.K. (1993): Fundamental of soil behaviour, 2nd. Edition. John Wiley and Sons, Inc., New York. [26] Mohan, D., Jain, G.S. and Sharma, D.(1973): Foundations practice in Expansive soils of India, Proc. Of 3rd Int. Conf. on Expansive soils, Haifa, Israel, Vol.1,pp.319-324. [27] Katti, , R. K.(1979): Search for solutions to problems in black cotton soils, First Indian Geotechn. Soc. Annu. Lect., Indian Geotechn. J., 9(1), 188. [28] Chen F. H. (1975): Foundations on expansive soils, Elsevier Scientific Publishing Co., Amsterdam. [29] Desai, I.D. and Oza, B.N. (1997): Influence of anhydrous calcium chloride on shear strength of expansive soils, Proc.1st Int. Symp. On expansive soils, HBTI, Kanpur, India. [30] Nelson and Miller, J.D. (1992): Expansive soils: Problems and Practice in Foundation and Pavement Engineering, John Wiley Publication. [31] Snethen, D.R. (1979): An evaluation of methodology for prediction and minimization of detrimental volume change of expansive soils inhighway subgrades, Administration, Washington, D.C.