
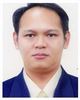
- Open Access
- Authors : Kittikom Nontprasat , Ganwarich Pluphrach , Surachate Chutima
- Paper ID : IJERTV9IS060021
- Volume & Issue : Volume 09, Issue 06 (June 2020)
- Published (First Online): 18-06-2020
- ISSN (Online) : 2278-0181
- Publisher Name : IJERT
- License:
This work is licensed under a Creative Commons Attribution 4.0 International License
Improvement the Bar Bow of Al2O3-TiC Thinner Bar in High Precision of Grinding and Cutting Processes
Kittikom Nontprasat1, Ganwarich Pluphracp
1,2Mechanical Engineering Department, Srinakharinwirot University, Ong-Kharak, Thailand
Surachate Chutima3
3Mechanical Engineering Department, King Mongkut University of Technology Thonburi, Tungkru, Bangkok, Thailand
Abstract The bar bow is directly of quality to manufacturing, the bar bending on thin material in the mechanic processes. In this work, to study the residual stress on surface of Al2O3-TiC component used as substrate for rear-write head in hard disk drive. In manufacture, the both side on thin material is machined by cutting and grinding process, as cutting tool is used the thin diamond blade of fine grain size and grinding wheel of fine grain size each of side, the high precision machine is carried out according to the method of experiments. The results were experimented by the bar bending, bar parallelism, surface roughness of grinding size and surface roughness of cutting side, and compared the results by analysis of variance (ANOVA). The results revealed that the grinding level and blade type affected the bar bending, blade type and blade thickness affected the bar parallelism, and surface roughness of grinding side is improved by the grinding level, while cutting side is main affected by blade type.
Keywords Ceramic Al2O3-TiC, Cutting and grinding process, Thinner bar, Bar Bending, Air Bearing Surface
-
INTRODUCTION
The machining process in industrials are a one of importance process, grinding and cutting processes are worked by removing material of workpiece or surface of the part to chips [1, 2]. The performance of the mechanical system is effected by compact factors such as heat generation, wear, and creep life and fatigue strength [3], it received by the several factors into operation such as cutting tool and workpiece materials, feed rate, spindle speed, depth of cut, coolant, tool construction, vibration and surface finish, etc. Therefore, it is essential to provide a sufficient relationship between tool type and cutting conditions, workpiece and geometrical parameters and tool material properties, which was the complexity of the machining process too difficult indications [4]. The blade thickness was not directly affected by the slider bar defection [5]. The dicing speeds depend on the diamond size and abrasive grains size that was affecting the cutting surface [6]. The material removal rate (MRR) is one quality of machining [7]. The roughness of the finished part, surface quality, process accuracy, and others are more important output parameters of the grinding process [8]. The surface roughness was affected by the combination of cutting parameters such as the cutting speed and feed rate [9]. The surface finish improved by increasing the grinding depth which the critical parameter during grinding is 10-15 m/sec for velocity range of grinding process and grinding depth of 30 m and the better grinding
wheel is CBN wheel which matched with the high grinding depth and good thermal properties [10], but the depth of cut was affected by cutting force [2]. The high sensitive indicators for thermal or mechanical influences on surface integrity were residual stresses. The mechanical effects of the grits lead to plastic deformations and the strong negative effective cutting angle in grinding induces plastic deformation on the surface. The material compression and deformation causing surface compressive residual stresses and the tensile residual stresses were affected by temperature in the grinding or cutting process. The main stress direction is oriented perpendicular to the direction of cut. The directional dependence of stresses into the surface layer correlates with the mechanical effects of the cutting edges. The more plastic deformation found perpendicular to the cutting direction. A bending distortion was produced as a result of the residual stresses generated by the scribing operation. An analytical model for the bending effect based on the residual stresses due to line-force dipoles was developed [11]. The interrelation between subsurface damage and residual stress introduced by the grinding and diamond polishing, the layer of high dislocation density was found near the surface [12]. Grinding depth and feed rate impacted to the hardened layer depth [13]. The grinding process with coarse diamond grains results in a deep residual stress layer and higher residual stress at a certain depth be a lower strength. The higher residual compressive stresses at the surface are assumed to be the result of higher tensile stresses near grain boundaries beneath the compressive stress layer and this will encourage the development of a crack along grain boundaries [14]. The abrasive of grinding was influences the strength of machined ceramics. One feature was that the grinding introduces residual stresses into the surface region. The residual stresses were affected by the compressive and hence beneficial to the strength. Some feature was that large cracks are frequently formed in the surface region during processing. These large cracks can be removed or reduced in size by a suitable grinding process. Therefore, both the magnitude and variation in strength can be significantly improved by applying abrasive grinding [15]. The optimization of the cutting condition can reduce costs or production time and enhance surface [16]. The researchers are studied the minimizing of surface roughness and reducing the vibration during the machining process [17]. They have presented the prediction of multiple degrees of freedom model, they investigated the relationship between the cutting tool and workpiece in the turning process. The orthogonal turning process operation was significantly influenced by the tool overhang and work cross-section on the
stable by [18], the surface finish was improved by a passive vibration damping into the boring process [19]. The internal finish turning was performed by [9], investigated on the influence of the geometry, material of the tool and workpiece on the process stability, the indication of the ratio of boring bar overhang to bar external diameter on the stability of the process was eminently affected. The tool position of dynamic adjustment during the turning process was the accuracy control of the mechanical adaptive system introduced by [20]. The analysis of variance (ANOVA) was a statistic tool for analysis more parametric combined, can find the relationship of two groups or more groups of data [1] [2], the investigations operated by [21], [22] showed the surface roughness affected by feed rate more than cutting speed with using Taguchi method [23] [24]. The machinability of Al/SiC MMC investigated by the better surface finish at high speed with low depth of cut and feed rate [25] [26].
This present paper is studying the systematic investigation on cutting machine of ceramic using diamond blade thickness 45, 48, and 71 µm and blade type SD1000, SD1200 and SD1500, with grinding wheel types is SD2500, and grinding level is 0, 12.7, and 23.5 mm refer center of grinding wheel. The experiments are assessed in terms of bar bending changes that were investigated and characterized in measure bar bending, bar parallelism, the surface roughness of the grinding side and cutting side with comparison results by analysis of variance (ANOVA).
-
METHODS AND EXPERIMENTAL PROCEDURE
A. Materials
The dimension of specimen is 15.24 (w) x 46.07 (L) x 1.25
(T) mm for mini section of experiment. The Al2O3-TiC mini section was bonded to the fixture by thermal adesive wax, the holding mini section of wafer fixed to the chuck table by vacuum and the bars location was overhang fix from the fixture at 2.54 mm. This experiment used the ceramic type Al2O3-TiC, the 65 % of Al2O3 with 35 % of TiC as the percentage of volume, and 1m of grain size particle of the polycrystalline microstructure. The physical properties are density 4,300 kg/m3, Youngs modulus 390 GPa, Poissons
Table 1. Parameters of experiment
Parameters
Tier1
Tier 2
Tier 3
Bt
SD1000
SD1200
SD1500
BTh (µm)
45T
48T
71T
Gl (mm)
0
12.7
23.5
Bt=Blade type, BTh=Blade thickness, Gl=Grinding level
-
Bar Bending Measurements
The bar bending measurements are performed using a high accuracy machine (Machine design, 1/1,000,000 inch accuracy). A non-contact photography technique is capture cross mark the deposition side of slider no. 2 and 52 then the machine will be created the reference line. The machine will be moving to slider no. 9, 19, 27, 35, and 45 represent. The machine calculated the distance of each point to the reference line and report to displays. The positive bar bending is more extended of grinding side from cutting side, the equal of extending both sides is zero bending, and the negative bar bending is lower extended of grinding side from cutting side.
-
Bar Parallelism Measurements
The bar parallelism calculated by difference of the bar thickness value of top and bottom side, as measured by a z- scope machine, with 3 zones separated such inlet cutting zone, middle zone, and exit zone.
-
Surface Roughness Measurements
The surface roughness measured both sides for the grinding side and cutting side by Atomic force measurement (AFM) machine model AFM5300E, the measured surface roughness (2D and 3D), with a sample square length of 10 x 10 µm, calculated the surface roughness (Ra) by averaging ten roughness values obtained 512 per point of both sides. Figure 1 shown 2D and 3D of surface roughness.
ratio 0.22, specific heat 880 J/kg , thermal conductivity 6,302
W/m , and Resistivity 20,000 -m.
B. Experimental setup
This study was using a high precision cutting machine with a maximum power of 10kW single spindle and a maximum spindle speed of 20,000 rpm by Dover precision machine. The special diamond blade three types used the SD1000, 1200, SD1500 by grit side, with the three thicknesses were the dimension of 45, 48, 71 µm, with 114 mm of outside's diameter, as the cutting process, the rotating of blade wheel 9,000 rpm and the feed rate 180 mm/min see in Table I. The special grinding wheel type was SD2500 of grit side, the dimension was diameter 69 mm and 2 mm Thickness, the rotation of grinding wheel was 9,000 rpm and the feed rate of
250 mm/min. Experiments were performed under wet conditions, as 7.571 liters/minute of constant coolant flow rate at 2% solution of rustic water-based, and 1.5 mm cutting of depth for both of the cutting and grinding process used.
Fig. 1 2D and 3D Surface roughness measurements
-
-
RESULTS AND DISCUSSIONS
In this study, the bar bending, the bar parallelism, the surface roughness (Ra) of grinding side, and the surface roughness of cutting side were shown the results in Table 2. The analysis of results used by the analysis of variance (ANOVA) method.
Table 2 Experimental results of grinding
Bt
BTh
Gl
BB
BP
RaG
RaC
SD1000
45T
0
-14.030
3.668
20.683
19.365
SD1000
48T
12.7
-19.240
3.447
19.957
15.120
SD1000
71T
23.5
-3.405
3.268
13.399
13.582
SD1200
45T
12.7
-24.781
3.468
18.740
12.651
SD1200
48T
23.5
-10.511
3.247
14.767
10.263
SD1200
71T
0
-26.073
3.168
22.814
10.741
SD1500
45T
23.5
-15.082
3.268
12.870
9.896
SD1500
48T
0
-33.690
3.047
21.143
7.039
SD1500
71T
12.7
-30.608
2.827
16.505
8.533
Bt
BTh
Gl
BB
BP
RaG
RaC
SD1000
45T
0
-14.030
3.668
20.683
19.365
SD1000
48T
12.7
-19.240
3.447
19.957
15.120
SD1000
71T
23.5
-3.405
3.268
13.399
13.582
SD1200
45T
12.7
-24.781
3.468
18.740
12.651
SD1200
48T
23.5
-10.511
3.247
14.767
10.263
SD1200
71T
0
-26.073
3.168
22.814
10.741
SD1500
45T
23.5
-15.082
3.268
12.870
9.896
SD1500
48T
0
-33.690
3.047
21.143
7.039
SD1500
71T
12.7
-30.608
2.827
16.505
8.533
Bt=Blade type, BTh=Blade Thickness, Gl=Grinding level, BB=Bar Bending, BP=Bar Parallelism, RaG=Roughness of Grinding side, RaC=Roughness of Cutting side
-
Results of Bar Bending and Analysis
Fig. 2, shows experiment results of bar bending trend, the 45T thickness blade increased the blade type SD1000 to SD1500, while the 48T and 71T are irregular trend. The grinding level of 12.7 mm shows the blade type SD1000 to SD1500 is increase bended, while 0 mm and 23.5 mm of grinding level show irregular.
As Table 2 of average of the bar bending was analyzed by ANOVA statistical technical. The technique was based on partitioning the variable of data by as assumed, however suitable, statistical model. Considering the absolute of evaluation data reading as the response variable, the contributing linear statistical model was used to explain the data for bar bending:
(1)
When, yij is the ijth observation, µ us the mean of the ith factor level or treatment, i is a parameter unique to the ith treatment, ij is a random error component. P-value approach is the probability of test statistic. A confidence level of 95% is performed analysis. Accordingly, the P-value for parameters is indicated the significance of bigger of = 0.5.
(a)
(b)
Fig. 2 Results of the bar bending with, (a) Bar types vs blade thicknesses, and (b) Bar type of vs grinding level.
The average of bending results were used the ANOVA by Minitab 14 software, the output shows in Table 3 was obtained. It can be seen the R2 value is 98.94% for average bending, the R2 value represents the percentage of data variability accounted by the model. It can be concluded that the effects of blade type and grinding level factors on bending are significant.
Table 3 ANOVA of bar bending for grinding
Source
DOF
SS
MS
F
P
%
Bt
2
306.43
153.21
36.79
0.026
39.47
BTh
2
15.64
7.82
1.88
0.347
2.02
Gl
2
454.39
227.19
54.55
0.018
58.52
Err
2
8.33
4.16
Total
8
784.79
100
DOF=Degree of freedom, SS= Sum of square, MS= Mean of square, F= F-ratio, P= P-Value, %=% contribution
-
Results of Bar Parallelism and Analysis
As Fig. 3 showed the variation of bar parallelism results on blade type – blade thickness and blade type – grinding level. It can see be, at 45T and 48T of blade shown the blade type reduce grain size of blade, the bar parallelism reduced, while 71T thickness blade was irregular tendency. As the all of them for grinding level show irregular.
The calculated of bar parallelism for ANOVA shown that the blade type and blade thickness are significant with 53.89% and 45.38% contribution affected at the R2 was 99.28% by Minitab software shown in Table 4.
(a)
(b)
(a)
(b)
(a)
(b)
(a)
(b)
Fig. 3 Results of the bar parallelism with, (a) Bar types vs blade thicknesses, and (b) Bar type of vs grinding level.
Table 4 ANOVA of bar parallelism for grinding
Source
DOF
SS
ME
F
P
%
Bt
2
0.2599
0.1300
74.11
0.013
53.89
BTh
2
0.2189
0.1094
62.41
0.016
45.38
Gl
2
0.0035
0.0018
1.00
0.500
0.73
Error
2
0.0035
0.0018
Total
8
0.4858
100
Fig. 4 Analysis of the surface roughness of grinding process with (a) Ra of grinding level, (b) Ra of ANOVA.
-
Results of Surface Roughness of Cutting Side and Analysis
Fig. 5 (a), (b), shows the graph of surface roughness for the cutting side, the blade type SD1000 was highest of surface roughness value all of blade thickness, while the blade type SD1500 was lowest of surface roughness value all of blade thickness. Similar, the ANOVA shows significant for blade type, p-value was 0.044 at 80.40% of % contribution, and the R2 is 96.46%, with 95% of confidential shown in Fig, 5 (b).
Interval Plot of Ra of cutting vs Blade Type, Blade Thickness
95% CI for the Mean
-
Results of Surface Roughness of Grinding Side and Analysis
The trend of graph shown the surface roughness decreased the grinding level increased see in Fig. 4 (a). The surface roughness results of the grinding side was significant by ANOVA with Minitab, it shows p-value < 0.05 of grinding level at 91.97% of % contribution, while blade type and blade thickness were showed not significant on p-value is 0.290 and 0.478, respect. The R2 is 97.78% in Fig. 4 (b).
20
Ra of cutting
Ra of cutting
15
10
5
0
Blade Thickness Blade Type
45T
48T SD1000
71T
45T
48T SD1200
71T
45T
48T SD1500
71T
(a)
(b)
Fig. 5 Show the surface roughness of cutting process with (a) Ra value of blade type with blade thickness, (b) Surface roughness analysis of blade type by ANOVA.
-
-
-
CONCLUSIONS
The grinding and cutting process for the slider bar was controlled by the slider bar defection or bar bending by the machining process. The residue stress induced in the grinding surface contributed force into the angle line direction. The cutting process-induced force into horizontal line direction, both directions of forces were significantly affected by slider bar bending. Three grinding level and three cutting blade are investigated. This experiment shows as below:
-
The bar bending result is affected 58.52% and 39.47% by grinding level and blade type, it show significantly,
-
The bar parallelism result is showed significant of blade type and blade thickness at 53.89% and 45.38% of the % contribution affected.
-
The surface roughness trend of grinding side is improved by grinding level.
-
The blade type is affected to surface roughness of cutting side.
ACKNOWLEDGMENT
The authors would like to thank you to the Industry/University Cooperative Research Center (I/UCRC) in HDD Advanced Manufacturing, Institute of FIeld roBOtics, King Mongkuts University of Technology Thonburi and National Electronics and Computer Technology Center, National Science and Technology Development Agency, Western Digital Company (Thailand), Srinakharinwirote University, Bangkok, Thailand.
REFERENCES
-
J. Ribeiro, H. Lopes, L. Queijo, and D. Figueiredo, Optimization of Cutting Parameters to Minimize the Surface Roughness in the End Milling Process Using the Taguchi Method, Periodica Polytechnica Mechanical Engineering, 61(1), 2017, 30-35. Doi: 10.3311/PPme.9114.
-
H. Tebassi, M.A. Yallese, T. Meddour, F. Girardin, and T. Mabrouki, On the Modeling of Surface Roughness and Cutting Force when Turning of Inconel 718 Using Artificial Neural Network and Response Surface Methodology: Accuracy and Benefit, Periodica Polytechnica Mechanical Engineering, 61(1), 2017, 1-11.
https://Doi.org/10.3311/PPme.8742.
-
M. Dogra, V.S. Sharma, and J. Dureja, Effect of tool geometry variation on finish turning A Review, Journal of Engineering Science and Technology Review, 4(1), 2011, 113.
-
G. Mustafa, and Y.Y. Emre, Application of Taguchi method for determining optimum surface roughness in turning of high-alloy white cast iron, Measurement, 46, 2013, 913919.
-
A. Nontprasat, V. Buakheaw, and C. Thanakhan, Reduce defection of slider bar from machining process, ME-NETT23, 2009, 112.
-
T. Adachi, K. Matsumaru, and K. Ishizaki, Diamond grain size effects on grinding-ability of dicing blades for cutting AlTiC (Al2O3-TiC composite), Journal of Austaria Ceramic Society, 43(1), 2007, 39-43.
-
S. Madival, M.R. Ahmed, M.L. Halappa, and L. Marulaiah, Application Potential of Fuzzy and Regression in Optimization of MRR and Surface Roughness during Machining of C45 Steel, Periodica Polytechnia Mechanical Engineering, 63(2), 2019, 132-139. https://Doi.org/10.3311/PPme.13171.
-
L.V. Shipulin, Complex Model of Surface Grinding, Proceedings of the International Multi Conference of Engineers and Computer Scientists, 2, 2012.
-
M. Sortino, G. Totis, and F. Prosperi, Development of a practical model for selection of stable tooling system configurations in internal turning, International Journal of Machine Tools & Manufacture, 61, 2012, 58 70.
-
H. Yunus, and J.F. ahman, Parametric Influence on Cutting Parameters Characteristics in Precision Machining of Ceramic Coating Materials, International Journal of Scientific & Engineering Research, 3(1), 2012. ISSN 229-5518.
-
Handbook of Ceramic Grinding and Polishing. Noyes Publications Park Ridge, New Jersey, U.S.A, and William Andrew Publishing, LLC Norwich, New York, U.S.A.
-
H. Yasui, R. Sakamoto, Y. Tanana, and T. Yoshimura, Influence of Normal Feed on Surface Roughness in Newly Developed Ultra- Smoothness Grinding Method, Thesis of Graduate School of Science and Technology Kumamoto University, Kumamoto city, Kumamoto, 2008.
-
J. Li, S. Liu, and C. Du, Experimental Research and Computer Simulation of Face Grind-hardening Technology, Journal of Mechanical Engineering, 59, 2013, 80-88.
-
P.H.J. Berg, and V.D. G.D. With, Strength and residual stress of Mg- PSZ after grinding, Elsevier Sequoia, Wear, 160, 1993, 301-308.
-
W.H. Tuan, and J.C. Kuo, Contribution of Residual Stress to the Strength of Abrasive Ground Alumina, Journal of the European Ceramic Society 19 © 1999 Elsevier Science Limited, 1998, 1593-1597.
-
K. Samir, M.H. Adel, and G. Amro, Investigation into the turning parameters effect on the surface roughness of flame hardened medium carbon steel with TiN-Al2O3-TiCN coated inserts based on Taguchi technique, World Academy of Science, Engineering and Technology, 59, 2011, 21372141.
-
L.V. Martinez, J.C. Jauregui-Correa, and E. Rubio-Cerda, Analysis of compliance between the cutting tool and the workpiece on the stability of a turning process, International Journal of Machine Tools and Manufacture, 48, 2008, 10541062.
-
K.K. Rama, and J. Srinivas, Study of tool dynamics with a discrete model of workpiece in orthogonal turning, International Journal of Machining and Machinability of Materials, 10(1-2), 2011, 7185.
-
S. Kanaseand, and V. Jadhav, Enhancement of surface finish of boring operation using passive damper, Indian Journal of Applied Research, 2(3), 2012, 6870.
-
A.A. Tareq, Extending the technological capability of turning operation, International Journal of Engineering, Science and Technology, 2(1), 2009, 192201.
-
R.P. Pragnesh, and V.A. Patel, Effect of machining parameters on surface roughness and power consumption for 6063 Al alloy TiC composites (MMCs), International journal engineering research and applications, 2(4), 2013, 295300.
-
U.D. Gulhane, S.P. Ayare, V.S. Chandorkare, and M.M. Jadhav, Investigation of turning process to improve productivity (MMR) for better surface finish of AL-7075-T6 using DOE, International journal of design and manufacturing technology, 4(1), 2013, 5967.
-
A. Mannaa, and B. Bhattacharya, A study on machinability of Al/SiCMMC, Journal of Materials Processing Technology, 140, 2013, 711-716.
-
B.D. Narayana, and S.B. Chenata, Optimization of cutting parameters for turning aluminum alloy using Taguchi method, International Journal of Engineering Research and Technology, 2(7), 2013, 1399 1407.
-
T. Sasimurugan, and K. Palanikumar, Analysis of the machining characteristics on surface roughness of a hybrid aluminum metal matrix
composite (Al6061-SiC-Al2O3), Journal of Minerals & Materials Characterization & Engineering, 10(13), 2011, 12131224.
-
N.B. Doddapattar, and S.N. Lakhmana, An optimization of machinability of aluminum allows 7075 and cutting tool parameters by using Taguchi technique, International Journal of Mechanical Engineering and Technology, 3(2), 2012, 480493.
-
C.M. Douglas, Design and Analysis of Experiments, John Wiley & Sons, Inc. 8th edition, 2013, pp. 69. A. Cichocki and R. Unbehaven, Neural Networks for Optimization and Signal Processing, 1st ed. Chichester, U.K.: Wiley, 2, 1993, 45-47.