
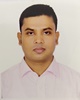
- Open Access
- Authors : Engr. Md. Arifujjaman , Engr. Indrajit Kumar Paul , Engr. Asif Raihan
- Paper ID : IJERTV10IS040023
- Volume & Issue : Volume 10, Issue 04 (April 2021)
- Published (First Online): 13-04-2021
- ISSN (Online) : 2278-0181
- Publisher Name : IJERT
- License:
This work is licensed under a Creative Commons Attribution 4.0 International License
In-Situ Load Test of Two storied Ferrocement Experimental House at HBRI Campus
Engr. Md. Arifujjaman
Senior Research Engineer
Structural Engineering & Construction Division Housing and Building Research Institute.
Engr. Indrajit Kumar Paul
Research Engineer
Soil Mechanics and Foundation Engineering Division
Engr. Asif Raihan
Research Fellow
Application of Ferrocement Technology in Rural Housing
Abstract:- Ferrocement is a versatile construction material and it is used widely in developing countries for housing, sanitation, agriculture, fisheries, water resources, water transportation (both in freshwater and marine environment), biogas structure, etc. The uniform distribution and high surface area to volume ratio of its reinforcement results in better crack arrest mechanism. This property makes ferrocement as an ideal tool of repairing and re-strengthening of older and deteriorated structure/structural members. This research work is focused on nondestructive evaluation of a two storied ferrocement experimental house inside HBRI premise. The in-situ load test technique for concrete systems mentioned in the ACI 318 Building Code Requirements for Structural Concrete, namely the 24h load test method and its evaluation criteria has been in use for several years. The primary goal of load testing is to demonstrate the safety of a structure. Load test do not determine the design strength or load carrying limit. In general, load tests can be used to determine the ability of a structure to support additional loads, to establish the safety of structures. The method to be followed is by providing gravity load with water because of its relatively low specific weight and also water is typically feasible when the test loads do not exceed 200 psf. The water was filled up to 2.06 ft height (calculated from load intensity) to impose desired load. The first floor and roof floor were filled with water step wise and the deflection at suitable points was measured. The total test load was maintained for a period of 24 hour. Then, the test load was removed and a set of final readings were taken 24 h after the removal of the test load. Test results showed that the structure had adequate strength for future use. Further studies could confirm the findings of previous researchers by a combination of tests, instead of performing just one type of test. It would provide more suitable results to confidently accept or reject the structure as a whole or its component for future use.
-
FOREWORD:
CHAPTER-1 (INTRODUCTION)
In-situ load testing is commonly used to assess the safety or the serviceability of an existing or new structural system or part of its elements for a particular external load condition. The most frequently used load testing procedure includes the in-situ load test method described in Chapter 20 of the ACI 318 – Building Code Requirements for Structural Concrete. While it is usually possible to demonstrate the safety of an existing structure through calculations based on general accepted engineering principles, this is not always the case. Sometimes there are structures for which calculations alone may not be sufficient to demonstrate fitness for intended occupancy or use. In such situations, in-situ load testing can provide valuable information about the performance of existing structures, and can take advantage of beneficial structural behavior not readily apparent with conventional computational methods.
The primary goal of load testing is to demonstrate the safety of a structure. Load tests do not determine the design strength or load-carrying limit. In general, load tests can be used to determine the ability of a structure to support additional loads, to establish the safety of structures with design or construction deficiencies or structures with damage or deterioration, to validate design approaches for and effectiveness of strengthening schemes, to gain knowledge on the behavior of a structure by accounting on the beneficial effects of hidden load paths, and to supplement, validate or tune-up analytical work aimed at understanding the behavior of a structure.
-
LOAD TESTING IN THE EVALUATION OF A STRUCTURE
The objectives of any structural evaluation are to establish the existing condition of the structure, identify issues affecting the structural performance, and develop and implement any remedial actions required. Very often, the evaluation of an existing structure requires performing structural analysis to investigate the ability of the structure and components to resist
the load demands prescribed by the governing building code.
Frequently, the evaluation of existing structures requires a field condition appraisal to determine the condition of the structure. The information collected in the field is used to supplement the structural analysis conducted to establish the adequacy of the structure or components of the structure in question. However, in some situations, the results of the structural analysis may not be conclusive and a high level of confidence in the results cannot be achieved. In situations like these, in-situ load testing may be required to gain further knowledge about the behavior of the structure to conclusively establish its adequacy.
Load test programs can be expensive and, as such, they should only be used when analytical resources have been exhausted and when there is high confidence that the results of the load test can help with answering questions about the structural behavior not evident from other analyses.
-
PROBLEM IDENTIFICATION:
As structures are aging, the assessment of buildings, bridges, tunnels, dams and industrial structures is becoming increasingly important. Structural codes have been developed for new design, but they often are not appropriate for assessment since there are significant differences between design and assessment. Design uncertainties arise from the prediction of load and resistance parameters of a new structure. These uncertainties represent the variability of a large population of structures caused by the unequal qualities of material, the different construction practices, and the variability of site specific live loads. Also a conservative design does not result in a significant increase in structural cost while a conservative assessment may result in unnecessary and costly repairs or replacement. Therefore, there is a clear need for technical rules for the assessment of existing structures.
A common problem facing design professionals involved in the modification, repair and reuse of existing structures is how to assess the capacity of an existing structure when the original design plans and specifications are not available. The assessment requires an understanding of the future use, structure type and dimensions, material properties used in the original construction, the extent of deterioration present and current building code status of the structure. This report describes methods for assessment of existing structures with a focus on the assessment of the existing ferrocement material properties and structural conditions.
-
OBJECTIVES OF THE RESEARCH:
There are two main objectives to conduct assessment of existing structures, the assurance of structural safety and serviceability and the minimization of costs. The objectives, benefits and risks associated with the load test program should be clear to all the parties involved (i.e. owners and building officials). Load tests typically have the following objectives:
-
It demonstrates that the structure or structural element ca safely resist the design loads with an adequate factor of safety against failure,
-
It demonstrates that the service loads do not cause deflections or crack widths that exceed industry standard limits, or preset values established for operation of a given structure,
So the objective of this research is to develop and verify the ferrocement design criteria. The test will give a estimation of the safety margin for ferrocement structure.
-
STRUCTURAL SAFETY AND SERVICEABILITY
The main task of assessment is to ensure that the structure or parts of the structure do not fail under loading. The assessment is carried out for ultimate limit states, which are:
-
loss of equilibrium of the structure or parts of it as a rigid body (e.g. overturning)
-
attainment of the maximum resistance capacity
-
transformation of the structure or part of it into a mechanism
-
instability of the structure of part of it
-
sudden change of the assumed structural system to a new system (e.g. snap through)
A reduction of serviceability may lead to a limitation of use and therefore serviceability assessment might become necessary. Serviceability limit states include:
-
local damage which may reduce the working life of the structure
-
unacceptable deformations which affect the efficient use
-
excessive vibrations which cause discomfort to people
Safety and serviceability can be evaluated for a variety of reasons, among others for changes in use or increase of loads, effects of deterioration, damage as result of extreme loading events and concern about design and construction errors and about the quality of building material and workmanship.
Increases of the maximum live load limits and changes of use are probably the main reasons for structural assessment. For buildings such changes could result in the need to support higher floor loadings.
Any structure is undergoing some degree of deterioration. The effects of deterioration are structure and site specific. Concerning structural strength, corrosion and fatigue are the main deterioration processes. Spalling, cracking, and degraded surface conditions are typical indications of deterioration.
Impact, earthquake or wind storms can result in structural damage. The remaining load carrying capacity needs to be analyzed after such events.
It may be necessary to assess an existing structure, after concerns about the correct design and constructions arise, including low quality building material or workmanship.
-
-
-
METHODOLOGY:
The load test protocol includes the loading procedures and the means to evaluate the performance of the structure during the load test and at completion of the load test. The load test protocol has two components that are dependent on each other: the loading procedure and the acceptance criteria.
The loading procedure describes how the structure will be loaded: load steps required to reach the ultimate test load magnitude, duration of each load step, loading and unloading requirements, hold period for the test load magnitude, etc.
The acceptance criteria describe the parameters to evaluate the performance and acceptability of the structure. These parameters can be qualitative or quantitative. Qualitative parameters are those that can be assessed visually, including formation of new cracks, widening of existing cracks, or other damage caused by the applied loads. Quantitative parameters are those that can be physically measured and can include deflections, strains, angles of slope, and crack widths.
The ACI Committees 318 prescribes protocol for load testing of concrete structures: Monotonic Load Testing.
1.6 ORGANIZATION OF THE REPORT:
The remaining portion of this thesis is divided into four chapters.
-
Chapter 2 covers the literature review and background related to load testing code provisions, application of load testing, reliability-based design, reliability-based calibration of design codes, reliability-based load testing concepts, and current load testing practices in bridge evaluation.
-
Chapter 3 contains the detailed experimental program done during the study.
-
Chapter 4 discusses the experimental results obtained during the experimental program.
-
Finally in chapter 5, a conclusion and recommendation of further research are made by the author.
-
INTRODUCTION:
CHAPTER-2 (LITERATURE REVIEW)
Ferro-cement material has long been in the area of interest among the researchers. Now it became necessary to implement it in the practical field and evaluate its performance. As a outcome the in-situ load takes into place.
Among the investigators Gustavo Tumialan in his paper wrote mentioned, the primary goal of load testing is to demonstrate the safety of a structure. Load tests do not determine the design strength or load-carrying limit. In general, load tests can be used to determine the ability of a structure to support additional loads, to establish the safety of structures with design or construction deficiencies or structures with damage or deterioration, to validate design approaches for and effectiveness of strengthening schemes, to gain knowledge on the behavior of a structure by accounting on the beneficial effects of hidden load paths, and to supplement, validate or tune-up analytical work aimed at understanding the behavior of a structure. There are different methods for load testing like:
-
Loading using Water and Dead Weights
-
Loading using Hydraulic Jacks
In this study loading with water weight will be used. In the United States, there are two protocols for load testing of concrete structures: Monotonic and Cyclic. These load test protocols are standardized by the American Concrete Institute (ACI) codes in ACI 318, Chapter 20 Strength Evaluation of Existing Structures and ACI 437 Code Requirements for Load Testing of Existing Concrete Structures and Commentary. The latter is an ACI standard recently developed by ACI
Committee 437 which includes a protocol for monotonic load testing with some modifications to the protocol currently specified by ACI 318, and a protocol for cyclic load testing (not included in ACI 318). The selection of a load test protocol typically depends on different parameters such as the objectives of the load test, site conditions, time constraints, costs, and familiarity with a load test protocol. Thus, ACI 437 permits the use of either the monotonic or cyclic load testing, at the discretion of the engineer. Monotonic load test protocol has been used for several decades for the structural evaluation of concrete structures. The procedure basically involves loading the structure in a monotonic manner by gradually applying the load until reaching the test load magnitude, which is maintained for 24 hours. Measurements are recorded before any load is applied, after each load increment, when the maximum load is achieved, after 24 hours of sustained loading, and 24 hours subsequent to the removal of the test load. The structure is evaluated based on the maximum recorded deflection and the amount of deflection recovery. Monotonic loading can be achieved using dead weights or hydraulic jacks.
-
-
FERROCEMENT:
Ferrocement is a composite material consisting of rich cement mortar matrix uniformly reinforced with one or more layers of very thin wire mesh with or without supporting skeletal steel. Ferrocement is also written as ferrociment, ferrocemento, ferrocimento, and ferrozement. Literally meaning is much steel rather than much concrete. Ferrocement is sometimes referred to as thin-shell concrete.
American Concrete Institute Committee 549 has defined ferrocement in broader sense as a type of thin wall reinforced concrete commonly constructed of hydraulic cement mortar, reinforced with closely space layers of continuous & relatively small diameter mesh. The mesh may be made of metal or other suitable materials. Ferrocement possesses a degree of toughness, ductility, durability, strength & crack resistance which is considerably greater than that found in other forms of concrete construction. These properties are achieved in the structures with a thickness that is generally less than 25 mm, a dimension that is nearly unthinkable in other forms of construction & a clear improvement over conventional reinforced concrete.
-
CONSTITUENT MATERIALS:
-
CEMENT:
The cement used should confirm to ASTM specifications. There are several types of cements which are available commercially in the market of which Ordinary Portland cement, Portland composite cement are the two most commonly used.
-
AGGREGATES:
The most common aggregate used in ferrocement is sand .Sand should comply with ACI 549.1R-93 and ASTM C33 standard fine aggregate. Aggregate is the term given to the inert material & it occupies 60 to 80 % of the volume of mortar. Aggregates to be used for the production of high quality mortar for ferrocement structure must be strong enough, impermeable & capable of producing a sufficiently workable mix with minimum water / cement ratio to achieve proper penetration of wire mesh. The sand cement ratio is kept from 2 to 4.
-
REINFORCING MESH:
One of the essential components of ferrocement is wire mesh. Different types of wire meshes are available almost everywhere, they generally consist of thin wires, either woven or welded into the mesh but main requirement is that it must be easily handled and if necessary, flexible enough to be bent around sharp corners. The function of wire mesh and reinforcing rod is to provide the form and to support the mortar in its green state. In the hardened state, its function is to absorb the tensile stresses on the structure which the mortar on its own would not be able to withstand.
-
MIXING WATER:
The quality of mixing water for mortar has a visual effect on the resulting hardened ferrocement. Impurities in water may interfere with setting of cement & will adversely affect the strength of cause staining of its surface & may also lead to its corrosion of ferrocement. Usually water that is piped from the public supplies is regarded as satisfactory. The water cement ratio is generally kept from 0.3 to 0.5.
-
ADMIXTURES:
-
Admixtures are used to alter or improve one or more properties of cement mortar or concrete. Most of the admixtures are used to improve the workability, to lesson water demand & to prolong mortar setting. Admixtures can be classified into groups according to the effect they are expected to achieve. The commonly used admixtures
-
Accelerating admixtures
-
Retarding admixtures
-
Water reducing admixtures
-
Air entraining admixtures.
A new class of water reducing admixtures has emerged during last two decades, known as "Super plasticizer". There are the high range water reducers.
-
HISTORICAL BACKGROUND:
The credit of using ferrocement in the present day goes to Joseph Louis Lambot who in 1848 constructed several rowing boats, plant pots, seats & other items from a material he called ferciment. Lambots construction consisted of a mesh or a grid reinforcement made of two layers of small diameter on bars at right angle & plastered with cement mortar with a thin cover to reinforcement Lambots rowboats were 3.66 m long ,1.22 m wide & 25 mm to 38 mm thick .These were reinforced with grid & wire netting . One of the boat build by him, still in remarkably good condition, is on display in the museum at Brignoles, France.
There was very little application of true ferrocement construction between 1888 & 1942 when Pier Luigi Nervi began a series of experiments on ferrocement. He observed that reinforcing concrete with layers of wire mesh produced a material possessing the mechanical characteristics of an approximately homogenous material capable of resisting high impact. After the Second World War, Nervi demonstrated the utility of ferrocement as a boat building material.
In 1945, Nervi built the 165 ton Motor Yatch Prune on a supporting frame of 6.35 mm dia rods spaced 106 mm apart with 4 layers of wire mesh on each side of rods with total thickness of 35 mm. It weighed 5% less than a comparable wooden hull & cost 40% less at that time.
In 1947, Nervi built first terrestrial ferrocement structure, a storage warehouse of about 10.7 m × 21.3 m .size .The strength of the structure was due to the corrugations of the wall & the roof which were 44.45 mm thick.
In 1948 Nervi used ferrocement in first public structure, the Tutrin Exhibition building. The central hall of the building which spans 91.4 m was built of prefabricated elements connected by reinforced concrete arches at the top & bottom of the undulations.
In 1958, the first ferrocement structure a vaulted roof over shopping centre was built in Leningrad in Soviet Union.
In 1970, a prototype prefabricated ferrocement home was constructed in U.S.A. The house was found much lighter in weight & higher in resistance to dynamic load than the conventionally built brick or block house.
In 1971 a ferrocement trowler named Rosy in I was built in Hong Kong. It had an overall length of 26 m & is claimed to be the worlds longest ferrocement fishing boat. In 1972, the US National Academy of sciences through its board on sciences & technology for international Development established an adhoc panel on the utilization of ferrocement in developing countries. In 1974, the American Concrete Institute formed committee 549 on ferrocement. In 1975, two ferrocement aqueducts were designed & built for rural irrigation in China.
In 1976, the International Ferrocement Information Centre (IFIC) was founded at Asian institute of Technology, Bangkok, Thailand). The centre is financed by the United States Agency for International development, the Government of New Zealand & the International Development Research Centre of Canada.
In 1978 an elevated metrostation of 43.5 m × 1.6 m in size with continous ferrocement roofing was erected in Leningrad.
In 1979 RILEM (International Union of Testing & research Laboratries of materials & structures) established a Committee (48-FC) to evaluate testing methods for ferrocement.
-
POTENTIAL APPLICATIONS:
Ferrocement has found wide spread applications in housing particularly in roofs, floors, slabs, & walls. Some researchers were also made on the use of ferrocement in beams & columns. Ferrocement roofs investigated included shell roofs, folded plates & the channel shaped roofs, box girders & secondary roofing.
(Kaushik et al 1987) investigated the behaviour of ferrocement cylindrical shell units as roofing elements and found that they can be used as roofing elements for low cost housing & satisfy Indian requirements of loading deflections & crack width with economy.
(Jagdish & Radhakrishna. 1977) investigated the suitability & effectiveness of using the ferrocement hyperbolic paraboloid shall roofing units for short spans of 4 m. they recommend that the ferrocement hyperbolic paraboloid shells with two layers of chicken mesh are quite adequated.
-
OTHER APPLICATIONS:
Ferrocement applications to water resources structures are numerous. Ferrocement has been used for:
-
Water tanks
-
Canal linings
-
Aqueducts
-
Pipes
-
Ferrocement gates
-
Culverts
Ferrocement has been widely accepted as a suitable building material for Biogas structures & marine applications such as boats, ships, bazges, pontoons, treatment plnts, & floating docks etc.
-
ACI 318-14 CHAPTER 27:
ACI 318-14 is the Building Code Requirements for Structural Concrete; this is the model code adopted by many building codes in the US and elsewhere. Chapter 27 of ACI 318 (hereafter referred to as ACI 318 Ch. 27) presents code provisions for the strength evaluation of existing structures through the use of a 24-hour monotonic load test. ACI 318 Ch. 27 Commentary states that a strength evaluation could be performed for various reasons including, but not limited to:
-
-
the quality of materials is considered to be deficient,
-
there is evidence of faulty or erroneous construction,
-
there exists noticeable deterioration that may affect structural performance,
-
if the building is to be repurposed for a new function, or
-
for any other reasons where the structure does not appear to meet the requirements of the code (ACI 318-14, 2014).
-
ACI 318 CH. 27: TEST LOAD MAGNITUDE:
The testing required for the 24-hour monotonic load test (24-h LT) is based on arranging the TLM to generate maximum, critical deflections over a 24-hour period. The TLM for the 24-h LT should be at least the greatest of (ACI 318-14, 2014):
= 1.3
= 1.15 + 1.5 + 0.4 ( )
= 1.15 + 0.9 + 1.5 ( )
where D = dead load; L = live load; LR = roof live load; S = snow load; and R = rain load.
-
MONOTONIC LOADING PROTOCOL:
The TLM is to be applied in not less than four approximately equal increments. Once 100% of the applied test load is achieved, the test load is sustained for 24 hours. Once the 24 hours have passed, the response measurements are made then the load is removed. Then, 24 hours after the test load has been removed, another set of response measurements may be collected to measure the residual deflection, if necessary. Figure 2.1 illustrates the test load application of the monotonic load test; the monotonic loading protocol is the same for ACI 437, ACI 318 Ch. 27, and Chapter 20 of CSA A23.3.
Figure 2.1. Test Load Application for the Monotonic Load Test (ACI 437.2-13, 2014)
-
ACI 318 CH. 27: ACCEPTANCE CRITERIA FOR THE MONOTONIC LOAD TEST:
The success or failure of the 24-h LT is based on two acceptance criteria. The first criterion involves visual observation of the tested element; the practitioner must ensure no excessive cracking, spalling, or deflection is observed during the duration of the test. The second criterion is a quantitative assessment of the maximum deflection and residual deflection values after each load increment is applied, after the TLM is sustained for 24 hours, and 24 hours after the sustained load is removed (ACI 318-14, 2014). Given that the residual deflection is measured 24 hours after the sustained load is removed, the total duration of the 24-h LT is approximately 48 hours. The quantitative criterion considers the maximum deflection limits satisfying one of the following equations (ACI 318-14, 2014).
If the test satisfies the maximum deflection criterion (max) then the residual deflection criterion (r max) does not need to be satisfied (Ziehl et al., 2008). Additionally, if the test does not meet the equations for maximum and residual deflection, it is permitted to repeat the test 72 hours following the completion of the initial test.
-
ACI 318 CH. 27: ADVANTAGES AND DISADVANTAGES OF THE MONOTONIC LOAD TEST:
The advantages associated with the 24-h LT are:
-
The test uses simple acceptance criteria that are easy to measure, quantify, and assess.
-
Having been in practice for more than 90 years, the 24-h LT is a seemingly reliable method that has been validated through its application over the period of its existence.
The work of ACI Committee 437 to develop provisions for the CLT included carefully investigating the validity of the existing 24-h LT. Within that work, the following observations regarding the 24-h LT were made:
-
Acceptance criteria for the 24-h LT were developed for simply-supported members based on working stress design limits using material properties and technology available in the 1920s. The acceptance criteria does not provide any flexibility to accommodate for end fixity or material properties (ACI 437.2-13, 2014).
-
The cost and time associated with the 24-h LT are considerable given that the tested area has to be cleared and out of service for at least 48 hours, as the residual deflection is measured 24 hours after the initial 24 hour test is completed (Galati et al., 2008).
-
The result of the 24-h LT is a binary pass-fail as it was designed as a proof load test. The success of the test only indicates that the member can successfully withstand the TLM; no additional information beyond that about the ultimate resistance is provided.
2.2. APPLICATION OF CYCLIC AND 24-HR MONOTONIC LOAD TESTS:
This section of the literature review provides a review of existing in-situ and experimental applications for the CLT and 24- hr LT. Table 2.1 summarizes the concepts discussed in each application paper included in this section. Reinforced concrete (RC) slabs and beams were the primary type of elements investigated in these studies with one application study investigating a two-way, post-tensioned slab.
Table 2.1. Concept Summary: Application of 24-h LT and CLT; Ultimate Capacity Margin
Proof Load Testing
To Failure
Author (Year)
Geometry
24-h LT
CLT
in-situ
FEM
UCM
Casadei et al. (2005)
RC slab
Galati et al. (2008) Part 1
Ziehl et al. (2008) Part 2
PT slab RC slab
Liu and Ziehl (2009)
14, RC
beam samples
De Luca et al. (2013)
RC slab
-
IN SITU LOAD TESTING OF PARKING GARAGE REINFORCED CONCRETE SLABS: COMPARISON BETWEEN 24 H AND CYCLIC LOAD TESTING:
Casadei et al. (2005) use in-situ application of both the CLT and the 24-hr LT on a parking garage with one-way reinforced concrete slabs. This is one of the earliest application papers reporting on the comparison between the CLT and the 24-hr LT. The tests were conducted on identical slabs in the parking structure.
It was determined that both slabs did not pass each respective load test which showcased a consistent outcome regardless of load testing protocol. For this specific case study, the CLT and 24-hr LT yielded the same final outcome considering slabs that were subjected to the same test load magnitude.
Since the structure was scheduled for demolition, the slabs were loaded to failure following each load test. This allowed for the calculation of the Ultimate Collapse or Ultimate Capacity Margin (UCM) to be determined as per the following equation:
(%) = ( 1 /) × 100% ; where, Ptest-load is the load at which the structure exceeded an acceptance criterion and Pultfailure is the load at which the structure was deemed to have reached its ultimate capacity.
It was determined that the remaining strength reserve of the system beyond the test load magnitude was 18% for the CLT and 20% for the 24-hr LT. The similarity of the outcome between the CLT and 24-hr LT showcased promise that there is a likelihood of consistency between th two load tests. It was demonstrated that quantifying the UCM may provide greater insight into the resistance of a structure beyond proof loading which may be helpful to stakeholders and practitioners (Casadei et al., 2005).
-
IN-SITU EVALUATION OF CONCRETE SLAB SYSTEMS:
This two-part research paper focused on conducting the CLT and the 24-hr LT in two experimental scenarios: a two-way post-tensioned (PT) concrete slab system and a two-way reinforced concrete (RC) slab system. The two-way PT concrete slab was investigated as it was believed that the system was inadequate in both flexure and shear resistance due to many areas being characterized by tendon and reinforcement misplacement. The two-way RC slab system was investigated as the system exhibited distributed cracking at the positive and negative moment regions (Galati et al., 2008).
Using commercial Finite Element (FE) software, SAP 2000, the maximum theoretical deflection was generated in comparison to the maximum experimental deflection collected on site. Using uncracked and cracked assumptions in the FE model and comparing to the experimental results, it was evident that the first two CLT cycles exhibited uncracked behavior and the last two CLT cycles exhibited cracked behavior. The two middle CLT cycles exhibited uncracked behavior in the two-way post-tensioned concrete slab and cracked behavior in the two-way RC concrete slab. The outcomes of the CLT acceptance criteria are highly sensitive to the status of the section, whether cracked or uncracked, prior to load testing (Galati et al., 2008).
In Part II of this paper, the use of Acoustic Emission (AE) in both experimental procedures was discussed. Ziehl et al. state that AE used in conjunction with CLT creates more informative, complimentary testing outcomes. The acceptance criteria used to assess the structures in this research are presented in Table 2.2 (2008).
Table 2.2. Acceptance Criteria Proposed by Ziehl et al. (2008)
CLT Test
24-Hour Load
AE
Repeatability
Maximum Deflection
Calm Ratio vs. Load Ratio
Permanency
Residual Deflection
Cumulative Signal Strength Ratio
Deviation from Linearity
The two-way PT concrete slab was deemed satisfactory under both the CLT and the 24-hr LT with AE results confirming cracking and deflection phenomena throughout testing. Only CLT and AE experimentation was conducted for the two-way RC slab. Under the CLT, the slab failed the permanency and deviation from linearity criteria at load set 3 and beyond. Under AE, the slab exceeded the Cumulative Signal Strength Ratio limit at load set 4 and beyond.
As both experimental structures were still in service, the slabs were not taken to failure. However, the UCM was obtained using the calibrated FE model which was developed previously to estimate deflections. Ptest-load was the load at which an acceptance criteria failed while Pult. failure was the theoretical ultimate load or moment capacity of the slab. The UCM for each acceptance criteria was calculated based on the findings from the FE model; UCM results ranged from 19 33% with one data set at 54%. UCM values of 19 33% from the FE model were comparable to the UCM values of 18% and 20% from the experimental results presented by Casadei et al (2005).
The following observations summarize the different facets of load testing that were taken into consideration during the development of this thesis. The thesis investigated load testing code provisions, application of load testing, reliability-based design, reliability-based calibration of design codes, reliability-based load testing concepts, and current load testing practices in bridge evaluation.
-
-
Two load testing code provisions exist under the authority of ACI: ACI 437 and ACI 318
Chapter 27. ACI 437 provides recommendations on structural load testing for both a monotonic load test and a cyclic load. ACI 318 Ch. 27 provides guidelines on structural load testing using a monotonic load test. The primary differences between
the two code provisions are the loading protocols (cyclic versus monotonic), the acceptance criteria, and the dead and live factors used for the test load magnitudes load combinations.
-
Application studies of load testing provided insight into the similarities and differences of
cyclic load testing versus monotonic load testing. However, the scope of these studies was usually limited to specific structures (or test experiments) and there was no consideration given to the differing test load magnitude levels between the two tests; generally, the application studies of load testing focused on the loading protocols and assessment of acceptance criteria.
-
Recent load testing application literature by De Luca et al. (2013) emphasized the need to
transform load testing from a binary, pass-or-fail-outcome testing method into an informative, diagnostic testing method. It was concluded that a better understanding of the probability of failure and remaining strength of an element following a load test would provide valuable insight to practitioners tackling load testing projects.
-
The concept of reliability-based load testing was developed based on existing literature
which uses conditional probability theory to model proof load testing on structural elements (Hall, 1988). Probabilistically, the concept of conditional probability theory was
determined to be an acceptable method to evaluate the probability of failure of an element at a given proof load level.
Masetti et al. (2006) studied the behavior of one-way reinforced concrete slab. The hydraulics jack was used for loading on the slab and the Linear Variable Displacement Transducers (LVDT) was used to collect the slab deformations. The results were obtained from the analysis of the graph relationship between load and deflection. The maximum deflection should be not more than the allowable deflection from ACI 318 and the rebound (residual) deflection should be not less than the standard residual deflection that has followed ACI 318 as well.
Casadei et al. (2005) studied the structure response of two way slab. The hydraulics jack was used for loading on the slab and the LVDT was used to collect the slab deformations. The results were obtained from the analysis of the graph relationship between statics load and deflection and between cyclic loading and deflection. The conclusion was shown that the statics load gave the clearly results (deflection) than the cyclic load.
Ramana (2013) studied the concrete strength of a two way slab by Schmidt Hammer. The results were obtained from the graph relationship between rebound number and concrete strength.
The dimensions of the tested deck slab is 7.32 x 4.74 m. (length x width) and its thickness is 15 cm. The nine dial gages are installed at the points G1 to G9 and the dial gage no.5 (G5) is located at the middle of the slab as shown in Figure 1.
CHAPTER-3
(EXPERIMENTAL PROGRAM)
-
INTRODUCTION:
While testing on the ferrocement model house it is imperative to ensure the safety of the people who are involved with this research work. Therefore some ferrocement element such as ferrocement beam, ferrocement column were tested in order to determine its load capacity. So the test was conducted in three phases(flexure test of ferrocement laminated masonry beam, axial load test of ferrocement laminated masonry column and in-situ load test of two storied ferrocement experimental house) described below.
-
TEST PROGRAM:
In three phases, the test was conducted. In the first phase three beam specimens of same cross section and materials as ferrocement experimental house were tested for flexure under three point loading. In the second phase four frrocement laminated masonry short column and a rc column were tested under axial load to determine its load carrying capacity and compared with RC column. In the final phase, in situ load test was conducted by providing gravity water up to 2.06 ft height (128.75 lb) on ground floor and first floor.
-
TESTING PROCEDURE AND INSTRUMENTATION:
-
FLEXURE TEST OF BEAM:
The experimental programme consisted of three beam specimens. All beams had the same size and reinforced as same as the beam of ferrocement model house. The cross sectional area was 11.5×7 and the length was 6-8 which is similar as the ferrocement experimental house. Bricks of 9.5×4.5×2.75 sizes, 2-layer BWG wire mesh and 6-12 mm dia rod was used for the specimens as shown in the figure 3.2. Then a third point loading was conducted as shown in the figure 3.1 and the load test setup was shown in figure 3.3.
Figure 3.1. 3-Point Flexure Test Figure 3.2. Cross Section of FC Beam
Figure 3.3. Load Test Setup
-
AXIAL LOAD TEST OF COLUMN:
Columns are the most authoritative structural element in any structure that transfers the entire loads to the foundation. Slenderness ratio is an important aspect that determines the load carrying capacity of the columns. In general, the load carrying capacity of the concrete columns decreases with increase in slenderness ratio. Therefore, the strengthening of deficient columns is necessary to increase the load carrying capacity, ductility and energy absorption capacity that can be achieved by external confinement of column. External confinement can be done by using different materials such as ferrocement, fibre reinforced polymers (FRP), steel jacketing.
To determine the axial capacity, four short columns were casted. The cross sectional area was 12×7 and the height was 20. Bricks of 9.5×4.5×2.75 sizes, 2-layer 20 BWG wire mesh and 4-12 mm dia rod was used for the specimens as shown in the figure 3.5. Then an axial loading test was conducted. The arrangement of dial gauge to determine the axial deformation and lateral deformation on both sides of the column is shown in the figure 3.4.
Figure 3.4. Axial Load Test Setup
Figure 3.5. Cross Section of FC Brick Column
-
IN-SITU LOAD TEST:
In-situ load testing is commonly used to assess the safety or the serviceability of an existing or new structural system or part of its elements for a particular external load condition. The most frequently used load testing procedure includes the in- situ load test method described in Chapter 20 of the ACI 318 – Building Code Requirements for Structural Concrete. The load testing procedure is described below,
-
The method to be followed is by providing gravity load with water. The water to be filled up to 2.06 ft height(calculated from load intensity) to impose desired load .
-
The first floor and roof were filled with water step wise and the deflection at suitable points to be measured.
-
The deflection gauges to arranged in such a way that the deflection in one story did not affect the deflection measurement in other story.
-
A pump to be used to lift water to height. The water should be clean from any objectionable substance.
-
No leakage of water would not be allowed and when using water, water meters was used to measure the amount of water introduced in the containers. The water to be filled gradually and a measument of deflection was taken at every 6 in (According to ACI 318, test load shall be applied in not less than four approximately equal increments) fill of water.
-
Once the structure was adequately instrumented, at the locations where the maximum response was expected, initial values of each instrument was recorded not more than one hour before the application of the first load increment.
-
The total test load was maintained for a period of 24 h. The test load was then removed and a set of final readings
was made 24 h after the removal of the test load.
-
Pumps and hoses was used to convey the water to distant drains but the unloading process was significantly slow down.
-
The evaluation of the structure is based on two different sets of acceptance criteria to certify whether the member tested has passed the load test or not. First, a set of visual parameters (e.g., no spalling or crushing of compressed concrete
and evidence of excessive deflections) that would obviously be incompatible with the safety requirements of the structure should be evaluated. Secondly, the measured maximum deflections shall satisfy one of the following two equations:
Where,
lt=Span length of member h=Thickness of member
1=Deflection
r=Residual deflection
-
LOAD INTENSITY(ACI 318) :
If the measured maximum and residual deflections do not satisfy the Equations, the load test may be repeated, but not earlier than 72 hours after the removal of the first test load as specified in ACI 318-05 Section 20.5.2.
The total test load (including dead load already in place) shall not be less than the larger of (a), (b) and (c): a) 1.15D+1.5L+0.4(Lr or S or R)
b) 1.15D+0.9L+1.5(Lr or S or R)
c) 1.3D
Where, D= Dead load
L= Live load
Lr = Roof live load
-
LOAD CALCULATION:
-
-
-
Dead load of Channel=25 psf Dead load of Floor finish=15 psf
Dead load of Beam, column and partition wall(from 1st floor)=88 psf Load from Beam, column and partition wall (Ground floor) = 79 psf Total dead load=207 psf
15% of Dead load= 31.05 psf
Load intensity= 128.86 psf(maximum) Water height=2.06 ft
According to ACI 318, upto minimum 2.06 ft height level water should be given.
-
EQUIPMENT SETUP:
In order to obtain good and reliable results, the instrumentation to monitor the response of the structure during the load test should be carefully selected. When using electronic devices, all the data is typically collected with an automated data acquisition system to monitor the response of the structure during the load test. However, when using dead weights, water or dial gauges, data can also be manually recorded at the completion of each load step. The setup of deflection transducers and cameras are shown in figure 3.6.
Figure 3.6. Location of cameras and deflection transducer
Cameras were set at the locations where the maximum response is expected. Beam column joint and the bottom of beam and rib are the most critical location for a structure. At ground floor, camera 1 was set to observe the beam column joint and camera 2 will be set to observe the bottom of the beam and rib as shown in the figure 3.7 (a) & (b). Only one camera was set on the first floor to observe the beam column joint as shown in figure 3.7(c). To observe the overall structure camera 4 will be set outside the building as shown in figure 3.7(d).
-
(b)
-
(c) (d)
Figure 3.7. Location of Cameras (a) Camera 1, (b) Camera 2, (c) Camera 3, (d) Camera 4
To measure the deflection of beam and rib on both floor, three deflection transducers was set under the beam bottom and rib bottom. Deflection transducer 1 was set at the bottom of the beam and deflection transducer 2 was set at the bottom of the rib of the ground floor as shown in figure 3.8. (a). Similarly deflection transducer 3 was set at the bottom of the beam of first floor as shown in figure 3.8 (b). then results were obtained from data logger and the behavior of the structure was observed with CCTV monitor as shown in figure 3.8 (c).
(b)
(a)
(c)
Figure 3.8. (a) Deflection Transducer 1 & 2, (b) Deflection Transducer 3,
(c) Data Logger and CCTV monitor
CHAPTER-4 (RESULTS AND DISCUSSIONs)
-
INTRODUCTION:
Three ferrocement laminated masonry beams and four ferrocement jacketed masonr columns and one RC column were tested in two phases of this study to evaluate the performance. In the first phase, all beams were tested under three point loading. On the other hand, columns were tested under monotonically increasing concentric compression. Finally, two storied ferrocement experimental house was tested under gravity load. The results of experimental program, including a discussion of the observed specimen behavior are presented in this chapter.
-
FLEXURE TEST OF BEAM:
Ferrocement can be applied to increase the flexural strength of a concrete member by bonding with the concrete matrix. Investigation has done by brick matrix instead of concrete and found an increase in tensile and compressive strength. Three samples of same cross sections and reinforcement are introduced in this investigation. Flexural capacity of ferrocement jacketed brick beam is evaluated and comparing with plain concrete specimens. Effect due to ferrocement on flexure was found to be significant in increasing ductility which is an important property during seismic loading or earthquake.
Figure 4.1. Load vs Displacement Curve for Three Specimens
From figure 4.1, it can be observed that the average bending moment capacity of the ferrocement jacketed brick beam is 29.45 k-ft but the bending moment from existing structure is 12.2 k-ft. That means the ferrocement jacketed brick beam is safe for in situ load test.
3.1.1. FAILURE PATTERN:
Failure pattern of beam specimens are observed during experiment. Brittle failure is successfully resisted by using ferrocement and ductility is increased in a significant amount. Experimental results showed that use of ferrocement increase load carrying capacity, prevented spalling of brick as well as ductility of brick beam. Only flexure cracks is observed in the ferrocement laminated brick beams. Failure pattern is shown in figure 4.2.
Figure 4.2. Flexural Crack in FC Beam
Table 4.1. Test Results Of Ferrocement Jacketex Brick Beam
Specimen |
Cross sectional area() |
Load (Kips) |
Average load(Kips) |
Average bending moment capacity (k- ft) |
Bending moment from existing structure (k-ft) |
FCBB1 |
80.5 |
19 |
17.67 |
29.45 |
12.2 |
FCBB2 |
80.5 |
16 |
|||
FCBB3 |
80.5 |
18 |
-
AXIAL LOAD TEST OF COLUMN:
As the purpose of this study is to investigate the axial capacity of ferrocement jacketed brick columns and RC columns. The columns are cast with only longitudinal bars and no tie bar is used. Ferrocement jacketed brick column shows better post cracking response in uniaxial compression. A number of square brick columns jackted with ferrocement are tested and improved ductility and toughness are found. These properties are very important during seismic loading or earthquake.
-
LOAD VS AXIAL DEFORMATION:
Figure 4.3. Load vs Axial Deformation Curve of FCBC1, FCBC2, FCBC3, FCBC4 and RC samples
From figure 4.3, it can be observed that the average load bearing capacity of the ferrocement jacketed brick column is lower than the reinforced concrete column but the ferrocement laminated brick columns are more ductile than reinforced concrete column. From the graph, it can be seen that the reinforced concrete column collapse suddenly but in case of FC brick column it can sustain load for a long time.
-
LOAD VS LATERAL DEFORMATION(IN LONG DIRECTION) :
Figure 4.4. Load vs Lateral Deformation (in long direction) Curve of FCBC1, FCBC2, FCBC3, FCBC4 and RC samples
Lateral deflection in long direction with increasing load has also been measured for ferrocement jacketed brick column and reinforced column as shown in figure 4.4. For sample FCBC2 there was an error occurred during the test. Therefore, the data obtained from the graph is not satisfactory.
-
LOAD VS LATERAL DEFORMATION(IN SHORT DIRECTION) :
Figure 4.5. Load vs Lateral Deformation (in short direction) Curve of FCBC1, , FCBC3, FCBC4 and RC samples
Lateral deflection in short direction with increasing load has also been measured for ferrocement jacketed brick column and reinforced column as shown in figure 4.5. For sample FCBC2 there was an error occurred during the test. Therefore, the data obtained from the graph is not satisfactory.
-
TIME VS AXIAL DEFORMATION:
Figure 4.6. Time vs Axial Deformation Curve of FCBC1, FCBC2, FCBC3, FCBC4 and RC samples
Time is an important parameter as it can be observed that how far the column can bear the load. Therefore axial deformation is measured with time for ferrocement jacketed brick column and reinforced column. It can be observed from figure 4.6 that ferrocement jacketed brick column can bear the load for 6-10 minutes whether the reinforced concrte column lasts for 3.5 minutes. It proves ductile behavior of ferrocement.
Table 4.2. Test Result of FC Brick Beam
Specimen
Cross Sectional area(sqin)
Load (Kips)
Maximum load capacity (Kips)
Load from existing structure(kips)
FCBC1
84
80
80
25
FCBC2
84
80
FCBC3
84
58
FCBC4
84
65
-
FAILURE PATTERN:
For control specimen of compressive strength test some spalling effect is and after jacketing ferrocement the failure patters are transformed from brittle failure to ductile failure. Figure 4.7 show experimental failure pattern of ferrocement jacketed brick specimens and rc specimens respectively. While reinforced concrete columns are completely splited out, ferrocement jacketed brick columns are failed but not splited out, the brick materials are held by the ferrocement which is ductile behaviour. If this property is developed in structural member, during earthquake axial members will not fail instantly and this will lessen the risk of injury and also allow movement of people to safe location.
-
(b)
-
-
(c) (d)
(e)
Figure 4.7. Failure Patterns of (a) FCBC1, (b) FCBC2, (c) FCBC3, (d) FCBC4,
(e) RC Specimens
-
IN-SITU LOAD TEST:
Load tests were performed based on the guidelines of ACI 318-05. The number and arrangement of spans or panels to be tested were selected to maximize the deflection and stresses in the critical regions of the structural elements. In all, two beams on ground and 1st floor and one rib in the ground floor were tested. The number and arrangement of beams and rib to be tested were selected to maximize the deflection and stresses in the critical regions of the structural elements. More than one test load arrangements were used if a single arrangement was not resulting in maximum values of the effects necessary to demonstrate the adequacy of the structure. As per ACI 318 the test load (including dead and already in place load) should not be less than 0.85(1.4DL + 1.7LL). The test load was applied in five equal increments compared with the minimum figure of four, as recommended by the ACI. The test load was applied by providing gravity load with water of 128.86 psf for loading the ground floor and first floor. A pump was used to lift water to height. However, there was leakage problem while loading water. Figure show the arrangement and actual placement of deflection gauges, and loading arrangement by using water.
All the selected beams and ribs of both ground and 1st floor behaved well during the load test and could be considered for using in superstructure. Some cracks were visible during the loading phase and it was observed that these cracks did not widen during and after testing. Hence, these are non-structural cracks and may be repaired during finishing phase.
-
ACCEPTANCE CRITERIA:
-
ACI 318 suggests that concrete in loaded panels should not show any sign of failure including spalling and crushing. The measured deflections should satisfy one of the following conditions:
where lt, h, 1 and r are the shorter span of slab panel, slab thickness, maximum and residual deflections respectively. The remaining details can be found in article 20.5.2 of ACI 318. Table 4.3 presents the summary of all the load tests. For all cases the maximum deflection (1) was found to happen at the mid-span. All the panels except panel 2 on first floor qualified the ACI criteria. Although in the ribs of the first floor cracking were visible.
Table 4.3. Summary of Load Test Result
Panel |
1(ft) |
A=lt^2/20000h |
Result |
r |
1/4 |
Result |
Final Result |
Ch 01(Ground Floor Rib) |
0.003886208 |
0.005217391 |
Ok |
0.001682753 |
0.000972 |
Not Ok |
Adequate |
Ch 02 (Ground Floor Beam) |
0.001679108 |
0.00675 |
Ok |
0 |
0.00042 |
Ok |
Adequate |
Ch 04(1st Floor Beam) |
0.000533872 |
0.005217391 |
Ok |
6.6734e-05 |
0.000133 |
Ok |
Adequate |
It is worth mentioning that both the beams on the ground floor and 1st floor exhibited best performance. 1st floor beam showed lowest maximum deflection and ground floor beam showed lowest residual deflection.
From the load deflection curve, it is observed that beams of both ground and first floor show elasticity where rib of the ground floor show a small deformation which is in allowable limit (BNBC).
Figure 4.8. Load vs Deflection Curve for Beams and Rib on Ground Floor and 1st Floor
-
FERROCEMENT CHANNEL:
The building system uses pre-cast ferrocement roofing channels which are placed adjacent to each other and spanning over two supports. After partly filling the valley between channels with concrete, the channels form an idealized T-beam and are able to carry the load of a roof / floor. Ferrocement comprises of a uniform distribution of reinforcement by use of chicken wire mesh and welded mesh encapsulated in rich cement mortar, thereby achieving significant reduction in both steel reinforcement and dead weight of roof. This composition provides a more uniform distribution of strength as compared to RCC. In this test a ferrocement channel(span 12-3 and width 2-0) of 5 thickness was used. The cross section and the joint detail of the ferrocement channel are given below.
Figure 4.9 Cross Section of Ferrocement Channel Figure 4.10 Joint Detail of Ferrocement Channel
During testing, cracks were appeared in several ribs. The cracks were visible in the mortar between the joint of two ribs which is not structural. The cracks appeared in the bottom of the ribs are shown in figure 4.11.
Figure 4.11. Crack Appeared in the Bottom of Ribs
-
CONCLUSION:
CHAPTER-5 (CONCLUSION AND RECOMMENDATION)
Load testing is a method of strength evaluation that is more representative of the performance of the strengthened structural member than analytical approaches and can provide valuable information regarding the health and performance of a strengthened structural element. Based on the experimental investigation, the following conclusion can be made:
In the absence of a reliable model for predicting the compressive strength, tensile strength, and flexural strength of beams and column with the easily available ferrocement in context of Bangladesh, the current research successfully investigated the
capacity enhancement and stress field of the ferrocement from experimental viewpoint to introduce this new engineering material in the Bangladesh construction industry.
-
FLEXURE TEST OF BEAM:
According to the results obtained from experimental studies of beams under flexure loading, following conclusions can be drawn:
Ferrocement jacketing technique could be used effectively, if proper jacketing scheme is introduced.
In brick beams jacketed with ferrocement are found not so efficient in enhancing the bending moment capacity of brick. The other reason may be the low strength brick with this ferrocement is not enough to arrest the hoop tension.
Experimental results showed that use of ferrocement increase load carrying capacity, prevented spalling of brick as well as ductility of brick beam. Only flexure cracks is observed in the ferrocement laminated brick beams
-
AXIAL LOAD TEST OF COLUMN:
In case of eccentrically loaded columns, following conclusions can be drawn according to experimental results:
Ferrocement jacketing could be used an effective re-strengthening material for column element of building subjected to concentric loading.
Confinement with the ferrocement encasement improves the ultimate load carrying capacity and increases the axial and lateral deflection of brick column.
-
IN SITU LOAD TEST:
The load test was conducted without disrupting the normal operation of the structures.
The results demonstrated that newly constructed ferrocement experimental house is safe and serviceable. This types of structure can be developed in rural areas.
Both the beams on the ground floor and 1st floor exhibited best performance. 1st floor beam showed lowest maximum deflection and ground floor beam showed lowest residual deflection.
From the load deflection curve, it is observed that beams of both ground and first floor show elasticity where rib of the ground floor show a small deformation which is in allowable limit(BNBC).
Cracks were appeared in several ribs during testing. The cracks were visible in the mortar between the joint of two ribs which is not structural.
By this research we can develop a new type of ferrocement structure which is safe and serviceable and due to low cost housing we can spread this ferrocement laminated masonry structure in the rural area.
The results obtained are going to help in the project work to investigate the behavior of ferrocement house for various parameters and loading. This is useful to find solutions by searching new design techniques and method of constructions.
5.2 LIMITATIONS AND RECOMMENDATION:
In the continuation of this work, following recommendation can be proposed for the future research:
There are no clear guidelines regarding the quantitative level of safety following the successful application of a load test. Further study is required to overcome this problem.
The number of deflection transducer for measuring deflection and CCTV cameras for observing the behavior of the structure was moderate. To get accurate results the number of deflection transducer and cameras need to increase.
The loading and unloading procedure using water was time consuming and critical. Water speed was too high while loading as well as it was critically low while unloading. And also vision problem was occurred while measuring water height using water meter. Thats why the results obtained from the test were not so accurate. A system should be upgraded to overcome this problem.
ACKNOWLEDGEMENT
The research reported herein was carried out in the Structural Engineering & Construction Division, Housing and Building Research Institute underthe project of Application of Ferrocement Technology in Rural Housing
The acknowledgement for the work that follows is due to my very far-sighted & intelligent co-supervisor Engr. M. A. Wahed, Principal Research Engineer for his encouragement, guidance and support from the initial to the final level. He constantly shored me up, enabling me to develop an understanding of the subject. Especially thanks to Engr. Sadia Mannan Mitu for
assisting me as a research fellow of this project.
I express my sincere thanks to Engr. Mohammad Abu Sadeque, PEng, Director, Housing and Building Research Institute for providing every facility to work in the laboratory.
I would also like to thank all the staff members & lab assistants who were always there when I needed them in my experimental works.
Last but not least, my special thanks to my family members for their constant motivation. Without their unconditional support and constant inspiration it would not been possible for me to complete this research.
(REFERENCES)
-
Huang, Q., Gardoni, P. and Hurlebaus, S., 2011, Predicting Concrete Compressive Strength Using Ultrasonic Pulse Velocity and Rebound Number,
ACI Material Journal, V. 108, No. 4, pp. 403-412
-
Hertlein, B.H., 1992, Role of Nondestructive Testing in Assessing the Infrastructure Crisis, Proceedings, Materials Engineering Congress on Performance and Prevention of Deficiencies and Failures, ASCE, pp. 8091.
-
Mirmiran, A., 2001, Integration of Non- Destructive Testing In Concrete Education, Journal of Engineering Education, V. 90, No. 2,pp. 219-222.
-
Bray, D.E., 1993, The Role of NDE in Engineering Education, Materials Evaluation, [5] vol. 51, no. 6, pp. 651652.
-
ACI Committee 228, 2003, In-Place Methods to Estimate Concrete Strength (ACI 228.1R-03), American Concrete Institute, Farmington Hills, MI.
-
Samarin, A., and Meynink, P., 1981, Use of Combined Ultrasonic and Rebound Hammer
-
Method for Determining Strength of Concrete Structural Members, Concrete International, [9] V. 3, No. 3, pp. 25-29.
-
Miretti, R., Carrasco, M. F., Grether, R. O. and Passerino, C. R., 2004, Combined Non-Destructive Methods Applied to Normal-Weight and Lightweight Concrete, Insight, V. 46, No. 12, pp. 748-753.
-
Hola, J., and Schabowicz, K., 2005, New Technique of Nondestructive Assessment of
-
Concrete Strength Using Artificial Intelligence, NDT&E International, V. 38, pp. 251-259.
-
ASTM C42, 2003, Standard Test Method for Obtaining and Testing Drilled Cores and Sawed Beams of Concrete ASTM International, West Conshohocken, PA.
-
Nanni, A. and M. Mettemeyer, 2001, "Diagnostic Load Testing of a Two-Way Postensioned Concrete Slab," ASCE Practice Periodical on Structural Design and Construction, V. 6, No. 2, pp. 73-82.
-
Mettemeyer, M., 1999, In Situ Rapid Load Testing of Concrete Structures, Masters Thesis, University of Missouri Rolla, Rolla, Missouri, pp. 113.
-
Mettemeyer, M. and Nanni, A., 1999, Guidelines for Rapid Load Testing of Concrete
-
Structural Members, CIES 99-5, Report, University of Missouri Rolla, Rolla, Missouri.
-
ACI Committee 318, 2008, Building Code Requirements for Structural Concrete (ACI 318- 08).American Concrete Institute, Farmington Hills, MI.
-
Casadei, P., Parretti, R., Nanni, A. and Heinze, T., 2005, In Situ Load Testing of Parking
-
Garage Reinforced Concrete Slabs: Comparison between 24 h and Cyclic Load Testing,
-
Practice Periodical on Structural Design and Construction, V. 10, No. 1, pp. 40-48.
-
Bungey, J. H., 1989, The Testing of Concrete in Structures, 2nd Ed., Chapman and Hall, New York.
-
Fling, R. S., McCrate, T. E., and Doncaster, C. W., 1989, Load test compared to earlier
-
structure failure, Concrete International, American Concrete Institute, V. 18, No. 11, pp. 2227.
-
Hall, W. B., and Tsai, M. (1989). Load testing, structural reliability and test evaluation.
-
Structural safety, Elsevier Science, New York, Vol. 6, 285302.
-
"Minimum Design Loads for Buildings and Other Structures (7-05)," American Society of
-
Civil Engineers, Reston, VA., USA., 2006.