
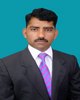
- Open Access
- Authors : Muhammad Arshad Javid, Muhammad Imran
- Paper ID : IJERTV9IS060965
- Volume & Issue : Volume 09, Issue 06 (June 2020)
- Published (First Online): 14-07-2020
- ISSN (Online) : 2278-0181
- Publisher Name : IJERT
- License:
This work is licensed under a Creative Commons Attribution 4.0 International License
In Vitro Haematological Effects and Attenuation of Ultrasound Waves in Human Blood
Muhammad Arshad Javid1*, Muhammad Imran1
-
Department of Basic Sciences (Physics), University of Engineering & Technology, Taxila, 47050, Pakistan.
-
Research Scholar, Department of Basic Sciences (Physics), UET, Taxila, 47050, Pakistan.
Abstract:- In vitro hematological effects of ultrasound have been reported in many studies to understand the absorption and attenuation mechanisms of ultrasonic waves in the human blood. The objective of this study is to assess the in vitro hematological effect and attenuation coefficient of the ultrasound in human blood at different frequencies. Pathological parameters of complete blood count (CBC) test such as hemoglobin, erythrocytes, leucocytes and thrombocytes cells were counted after the exposure of ultrasound. Blood samples of different groups (A+, A-, B+, B-, AB +, AB , O+, O-) were collected from 23 healthy subjects having mean age (34.56±14.20) years from BVH, Bahawalpur of Pakistan. Informed consent from subjects was taken to study the CBC test at 370C. The attenuation of ultrasound in blood was measured using Linear Transducer Probe-0805AT for 15 minutes. One way analysis of variance (ANOVA) test was used to assess the variability of blood cells in samples. A p-value (P 0.05) was deemed to be statistically significant in data. In this study, the attenuation coefficient () of ultrasound waves was measured at frequencies (3.5-11 MHz) using the power law. Statistical analysis revealed that in vitro exposure of ultrasound in human blood exposed a reduced amount of blood cells in CBC test of ultrasound samples (US) as compared to CBC test of control samples (CS).
Keywords: CBC, Ultrasound attenuation, Human blood, Frequencies, In vitro.
INTRODUCTION
Ultrasonography is a non-invasive diagnostic modality which is commonly used in the intracranial radiotherapy. In dental practice, it has been executed for cleaning the tooth and to kill the germs. It has been practiced in urology to remove kidney stones, to see blood flow irregularities and the position of the fetus in gynaecology [1]. In engineering sciences, ultrasonic testers are commonly applied to detect the porosity, flaws, uniformities, dimensional measurements and the characterization of materials [2-3]. Furthermore, ultrasound waves (USW) are employed in industrial processes such as plastic welding, material cleaning and widely used in sonochemistry [4].
Ultrasonic waves have been influenced by the mechanism of scattering and absorption when transmitted through the biologic tissues [5]. Resultantly, the energy and intensity of ultrasonic waves lose due to the heat and molecular exchange of energy in tissues [6]. The mechanism of scattering and absorption lead to classical attenuation coefficient of sound waves that depend on the frequency [7].When the sound waves pass through a medium i.e. air, soft tissues, cartilages, bones and blood, its amplitude and intensity are changed as an exponential function of penetration depth or distance. This reduction of amplitude and intensity is known as attenuation. The attenuation of US waves becomes larger in hard tissues and cartilages as compared to soft tissues [8]. However the temperature effect, cavitations streaming and free radicals effects have been reported in the previous literature [9-10]. Ultrasound absorption has been quantified directly by measuring the amount of acoustic energy converted into thermal energy or total acoustic attenuation [11]. Published literature has demonstrated that the ultrasound attenuation in blood shows nominal changes below 10 MHz frequencies due to its scattering of sound waves. Normally, the frequency of US waves is used from 1 MHz to 20 MHz in the medical field [12-13]. The scattering of ultrasound waves in human blood depends on the frequency approximately very close to Rayleigh scattering below 30 MHz and changes sharply at higher frequencies [14]. Previous studies have demonstrated that the attenuation of ultrasound in the blood depends upon the frequency, absorption mechanism and the concentration of blood cells [15,16,17]. Limited work has been explored about the attenuation of sound waves in the human blood. In this study, we have made an effort to assess the in vitro ultrasound exposure in blood cells such as hemoglobin, erythrocytes, leucocytes, thrombocytes and the attenuation coefficient in blood at different frequencies.
MATERIALS AND METHODS
To assess the ultrasound properties, five blood samples of each group (A +ve, A-ve, B+ve, B-ve,
AB +ve, AB ve, O +ve, O-ve) were collected from 23 healthy subjects having mean age with standard deviation (34.56±14.20) years in which gender was 15 male and 8 female from Bahawalpur Victoria Hospital of Pakistan. For this purpose, evacuated tube of polypropylene (3ml) containing K3-EDTA (ethylenediaminetetraaceticacidtri-potassium) size 13mm x 75mm and diameter 10.8 mm (Becton, Dickinson and Company, Franklin Lakes, NJ, USA) was used to perform the complete blood count (CBC) test at 370C. The complete blood count test was performed in the pathological lab under normal condition. In this study, ultrasound exposure was done in collected blood samples of each group for attenuation purposes. Pathological parameters of blood such as hemoglobin, erythrocytes, leucocytes and thrombocytes were considered to see the ultrasonic effects. Ultrasound waves were passed through K3EDTA tube for 15 minutes using Linear Transducer Probe PLM 805AT (3.5-11 MHz) of Toshiba Nemio XG iStyle. Blood samples were exposed by ultrasonic waves at different frequencies (3.5 MHz, 5 MHz, 6 MHz, 7 MHz,
9 MHz and 11 MHz). The pathological indicators i.e hemoglobin (HGB), erythrocytes, leucocytes, thrombocytes, mean corpuscular volume (MCV), hematocrit (HCT), mean platelet volume (MPV), mean corpuscular hemoglobin (MCH),
lymphocytes percentage (LYM%) in each blood sample were assessed by CBC reports as mentioned in Table-1. However, in this study we focused on blood cells such as hemoglobin, erythrocytes, leucocytes, hematocrit and thrombocytes to see mean count of blood cells after the exposure of ultrasound. The CBC reports of control samples were compared with CBC of ultrasound samples (US). Statistical analysis was performed using software (OrgionPro.8, www.origionLab.com). One way analysis of variance (ANOVA) test was used to assess the variability of pathological parameters of blood samples. A p-value (P
0.05) was deemed to be statistically significant in samples. In this work, power-law was used to assess the attenuation coefficient () as this power-law was referred in many studies by Bradley E. et al. (2010), Marcos M. et al.(2006), Shung et al( 1976), Gopala G.K et al.(1990) and Saleem MS. et al.(2011) to measure the attenuation coefficient as mentioned in equation-1.
= ——————– 1 [18]
Theoretically, in the case of blood, the power of frequency was taken (n =1) so it was taken linear to frequency and can be calculated using equation-2.
= 0.571 ——————– 2 [19-20]
The attenuation coefficient () of this experimental was measured using values mentioned in Table-2. The speed of ultrasound and density of human blood was calculated using equation-3 & 4 adding HCT (%), as mentioned in appendix.
c = 0.9 x HCT (%) + 1545 3
= 0.78 x HCT (%) + 1017 ————– 4 [12]
The comparative study of attenuation coefficient () of ultrasound of this in vitro study was displayed by Figure 2.
RESULTS
CBC test of blood samples was performed to assss the in vito haematological effects of ultrasound and the attenuation coefficient in blood cells. In this experiment, five samples of each blood group were studied at different frequencies (3.5 MHz, 5 MHz, 6 MHz, 7 MHz, 9 MHz and 11 MHz). CBC test of each blood sample was averaged before and after applying US waves. The effect of ultrasound waves in the human blood at different frequencies was mentioned in Table-1. One sample t-test induced a significant difference (P=0.001) when hemoglobin, erythrocytes, leucocytes and thrombocytes of blood samples were compared with and without ultrasound at different frequencies. A less amount of WBCs was seen as compared to normal profile of CBC test. The platelets near about 14.4-20 % decreased than normal profile and the hematocrit (HCT) i.e. percentage of RBCs in blood was also decreased [21]. HGB and mean corpuscular volume (average volume of RBCs) decreased and packed cell volume was also seen decreased. The number of platelets was also found about 5.5-10 % decreased as compared to normal profile. One way analysis of variance (ANOVA) test was used to assess the inconsistency of pathological parameters such as (RBC, WBC, HGB) of blood cells. Mean values with standard deviation of control samples CBC (CS) and CBC of ultrasound (US) at different frequencies (MHz) were displayed in Fig-1. A p-value found (p < 0.05) remained significant in CBC (CS) and CBC (US) samples. Furthermore the attenuation coefficient () was measured using power-law formula as mentioned in text references 12, 18 and 29. The attenuation coefficient () of blood samples was calculated in vitro at different frequencies. Elementary parameters such as 1 = density of blood, C1= speed of ultrasound in blood, C2=speed of ultrasound in polypropylene, Z1= Impedance in first media i.e blood, Z2= Impedance in second media i.e. Polypropylene, X= K3EDTA Tube diameter, = attenuation coefficient at 1MHz) were taken to calculate the attenuation coefficient as shown in Table-2 and Table 3. Figure-2 displayed the attenuation coefficient using power-law by prominent authors. In this study the results found the higher attenuation coefficient at frequencies (3.5 MHz to 11 MHz) as compared to previous studies. This higher attenuation coefficient trend may be due to K3EDTA Tube material structure in which the absorption, attenuation, relaxation time and the thermal mechanism of ultrasound vary as depicted in Figure-2.
Table-1. In vitro ultrasound effect of frequencies in blood cells.
f |
Blood Content |
RBC |
MCV |
HCT |
PLT |
MPV |
PDW |
WBC |
HGB |
MCH |
MCHC |
LYM |
(MHz) |
(10¹²/L) (Mean±S.d ) |
(L/L) (Mean±S. d) |
(%) |
(109/ L) (Mean± S.d) |
(f L) (Mean±S. d) |
(f L) (Mean± S.d) |
(109/L) (Mean ±S.d) |
(g/dL) (Mean± S.d) |
(pg) (Mean±S .d) |
(g/dL) (Mean±S. d) |
(%) |
|
3.5 |
CBC(CS) |
3.47± 0.28 |
85.75±2.3 |
32.85 |
217.5±7 0 |
8.35±1.06 |
10.65±0. 21 |
5.46±1 .02 |
8.61±0. 6 |
27.65±3.6 |
32.3±3.5 |
34.7 |
CBC(US) |
3.262±0.39 |
85.6±2.12 |
32.05 |
223±83. 4 |
8.4±0.84 |
10.95±0. 21 |
5.35±1 .2 |
8.26±0. 8 |
28.25±3.7 |
33±3.5 |
37.83 |
|
5 |
CBC(CS) |
3.83±0.66 |
83.1±4.4 |
28.9 |
194±82 |
8.33±0.7 |
11.66±1. 4 |
6.13±0 .61 |
8.46±1. 2 |
24.3±1.9 |
29.16±1.0 7 |
43.5 |
CBC(US) |
3.73±0.61 |
84±4.0 |
30.5 |
187.33± 81.1 |
9.16±0.56 |
11.26±.0 98 |
5.76±0 .4 |
10.7±3. 2 |
24.06±2.1 |
28.6±1.3 |
46.3 |
|
6 |
CBC(CS) |
3.6±0.36 |
75.86±9.0 |
40.43 |
250.3±7 1 |
7.26±1.1 |
9.16±2.1 1 |
6.9±2. 2 |
10.65±3 .18 |
24.7±3.4 |
32.3±0.65 |
33.96 |
CBC(US) |
3.52±0.37 |
75.7±8.51 |
39.26 |
238±67. 1 |
7.23±0.89 |
9.6±1.44 |
6.86±1 .1 |
10.83±3 .2 |
24.33±4.5 |
32.53±3.3 3 |
33.4 |
|
7 |
CBC(CS) |
4.98±0.69 |
81.95±6.7 |
29.31 |
220±96 |
8.4±1.2 |
11.8±1.3 |
7.4±1. 4 |
12.83±4 .2 |
24.3±2.5 |
29.36±1.6 |
38.66 |
CBC(US) |
4.83±0.69 |
82.36±7.4 |
29.48 |
226.83± 93 |
8.5±1.3 |
11.43±2. 08 |
7.06±1 .6 |
12.96±4 .8 |
24.2±2.3 |
29.41±0.9 |
39.18 |
9 |
CBC(CS) |
5.06±0.17 |
81.15±12 |
40.05 |
204.5±7 0 |
5.45±1.9 |
6.87±1.3 |
7.65±0 .6 |
13.95±2 .6 |
18.17±3.2 |
34.9±2.1 |
37.62 |
|
CBC(US) |
5.06±0.3 |
83.9±14.0 3 |
40 |
185.75± 72.96 |
5.4±1.2 |
7.2±2.1 |
7.05±0 .9 |
13.6±1. 4 |
29.72±6.6 |
35.1±2.2 |
38.92 |
||
11 |
CBC(CS) |
5.29±0.51 |
83.66±2.9 |
42.36 |
314±57 |
5.5±1.5 |
9.5±0.03 |
9.9±2. 2 |
14.18±3 .1 |
28.04±4.0 2 |
33.5±7.4 |
32.74 |
|
CBC(US) |
5.16±0.42 |
84.48±4.4 0 |
42.78 |
290.6±7 2 |
5.76±1.04 |
5.76±2.1 |
9.12±1 .9 |
13.98±0 .2 |
27.64±1.7 |
32.72± 1.01 |
32.96 |
f; frequency, MHz; Mega Hertz, CBC(CS); complete blood count (control sample), CBC(US); complete blood count (ultrasound samples ), fL = femto litre MCV; mean corpuscular hemoglobin, HCT; hematocrit, PLT; platelet count, MPV; Mean Platelet Volume, MCH: Mean Corpuscular Hemoglobin, MCHC; Mean corpuscular hemoglobin Concentration, LYM; Lymphocytes percentage, PWD; Platelet distribution Width, Pg = pico gram g/dL = gram per deciliter
Table-2: Attenuation Coefficient () in vitro
No |
(kg/m) |
(m/s) |
= MRayl |
(kg/m) |
(m/s) |
= MRayl |
= [ ] + |
X
(cm) |
= dB/cm |
Publish ed study |
1060 [19] |
1570 [24] |
1.7 |
913 [12, 22] |
2740 [12, 23] |
2.5 |
0.04 |
1.22 |
0.5711 |
Experi mental value |
1044 |
1577 |
1.65 |
913 |
2740 |
2.5 |
0.4250 |
1.22 |
0.562 |
1 = density of Blood C1= speed of US in blood, C2=speed of US in polypropylene, Z1= Impedance in first media i.e blood, Z2= Impedance in second media i.e Polypropylene, X= K3EDTA Tube diameter, = attenuation coefficient at 1MHz
Figure-1: In vitro ultrasound exposure in blood (CS; control sample CBC without ultrasound, mean values with standard deviation.
Table-3: In vitro measurement of attenuation coefficient ()
Frequency |
Experimental |
[24] | [29] | [12] | [30] |
(MHz) |
= 0.562 |
=0.18 |
=0.14¹·²¹ |
=0.0596¹·56 |
=0.087¹·²5 |
3.5 |
1.967 |
0.63 |
0.638 |
0.421 |
0.416 |
5 |
2.81 |
0.9 |
0.982 |
0.734 |
0.651 |
6 |
3.372 |
1.08 |
1.224 |
0.975 |
0.817 |
7 |
3.934 |
1.26 |
1.475 |
1.241 |
0.991 |
9 |
5.058 |
1.62 |
1.999 |
1.836 |
1.356 |
11 |
6.182 |
1.98 |
2.548 |
2.511 |
1.743 |
Figure 2: (a) Experiemtnal value (b) Hendee et al.[24] (c) Szabo et al. [ 29] (d) Bradly et al. [12] (e) Johnston et al. [30]
DISCUSSION
Human blood assumed to be consisting of blood cells 45% in volume and suspended in plasma under normal haematology analysis. Blood cells were made up of red blood cells (erythrocytes), platelets (thrombocytes) and white blood cells (leukocytes). The CBC test might be varied slightly owing to temperature effect under normal conditions. Previous studies had been used the power law to assess the attenuation coefficient () in human blood at different frequencies as mentioned in references 12, 18, 24, 29, and 30. It was well reported that attenuation mechanism in human blood depends on many factors at the cellular level owing to structure of molecule, intermolecular forces, viscosity and the thermal molecular properties [21]. Krishna et al. presented the acoustic impedance of haemoglobin, plasma and erythrocytes and reported higher acoustic impedance in erythrocytes as compared to whole blood at 3 MHz. In this study, the attenuation coefficient of blood samples was very close to Krishna et al. work [19]. The absorption in the human blood depends upon the viscosity due to a change in density of red blood cells and the surrounding plasma [24]. This mechanism leads to reduce the cell size of blood cells by increasing the tonicity of suspension and the osmosis process across the cell membrane.
The scattering mechanism in the human blood was strongly related to red blood cells including shear rate and the concentration of the plasma protein. That is why the concentration of blood protein was considered a biomarker of scattering and the absorption process at higher frequency of ultrasound. This study has explained the attenuation coefficient of red blood cell at different frequencies. The red blood cell lost its concentration due to application of ultrasound waves and due to the viscosity of relative motion at frequencies lower than 15 MHz [25, 26]. Therefore the less concentration of red blood cells was assessed after exposure of ultrasound as compared to the control samples. In this study we reported the haematocrit versus speed of sound and the density of human blood. The referred density of human blood was taken in 1060 (kg/m3) but density =1044 (kg/m3) was used to assess the absorption impedance (Z) and the attenuation coefficient () at different frequencies. Several experimental studies demonstrated that the attenuation coefficient changes due to the haemoglobin oxygenation as well as temperature [27-28-29]. Schneider et al. (1969) explained that a 4% increase in the attenuation coefficient at 10 MHz in deoxygenated haemoglobin solution as compared with oxygenated haemoglobin solutions. Similarly the attenuation coefficient decreased 8 % at higher frequencies for example 500 MHz. However, Shung and Ried et al. (1977) elucidated an increase of 60
% attenuation coefficient at 10 MHz. In this study the attenuation coefficient of HGB (g/dl) was increased at the rate of 1% at 3 MHz to 11 MHz. It was reported that the attenuation coefficient of ultrasound waves depends upon the concentration of red blood cells as well as blood proteins. This minimal increase in HGB might be ignored at physiologically level [12]. However, the attenuation coefficient of HGB rose directly at higher frequencies as shown in Table -2 and Figure-1. Williams A.R. et al. (1983) studied the interaction of ultrasound with platelets and found the adverse effect of ultrasound effects on platelets structure [30]. Another aspect of this study was to find decreased counts of platelets under ultrasound exposure. Treeby BE et al. (2010) observed the damaged morphology of platelet during exposure of ultrasound therapy [31]. Similarly, Lunan K.D et al.
also found reduced platelets during the application of ultrasound at different frequencies [32]. Meharpour M. et al (2016) studied the bioeffect of low intensity effects in the blood in vivo and found reduced number of platelets, HGB and HCT as explained by this report [33]. Ultrasound exposure on the leukocytes cells indicated a minimal effect approximate less than 1 %. Kasmawan et al. studied the ultrasound effect on leukocytes and erythrocytes in blood cell of mice and reported a decline of both blood cells [34]. Oleshkevich et al. (2017) investigated the effects of continuous and modulated ultrasound in blood cells of different animal species and reported the leukopenia, cytolysis, destruction, the aggregation of cells, foaming of the cytoplasm of granulocytes, rupture of the cytoplasmic membrane, bursting of nuclei, and their deformation and disturbance of their borders [36]. In this study, the leukocytes cell counts reduced in very small amount due to its morphology or high immune susceptibility of cell structure. This in vitro study has made an effort to assess the hematological outcome. Furthermore, the attenuation coefficient () at higher frequencies in human blood might be helpful to understand the physical aspects of ultrasound in medical sciences as well as physical sciences. In this study, ultrasound exposure was not conducted more than 11 MHz frequency. Ultrasound waves at higher frequencies may present noticeable change in blood cells concentration than low frequencies. The limitation of this study was a small data set of blood samples and gender based interpretation of ultrasound.
CONCLUSION
In vitro study of ultrasound exposure in human blood presented that hemoglobin, erythrocytes, leucocytes and thrombocytes cells count dropped than normal profile of CBC. The reduced blood cell during the ultrasound exposure was the outcomes of several biophysical mechanisms for example shear rate, temperature and frequency of ultrasound waves. This study also indicated that the attenuation coefficient of ultrasound vary at different frequencies (3.5 MHz to 11 MHz) in the human blood. Sheer power-law manipulation i previous and current study is not enough to explain the exposure of ultrasound waves in blood cells. Further improvements are requested to estimate the concentration of blood cells during the execution of ultrasound. Therefore this work may assist to biophysicists to evaluate the ultrasound exposure in human blood as well as to comprehend the attenuation coefficient of ultrasound at different frequencies.
ACKNOWLEDGMENT
We are thankful to Professor Dr. Mustansar Waraich who helped us to perform ultrasound experiments in BVH Hospital in Bahawalpur.
CONFLICT OF INTEREST
It is certified that there is no conflict of interest among authors
AUTHOR CONTRIBUTION
Muhammad Arshad Javid proposed the idea of this study, Muhammad Imran conducted the experiments under supervision of Dr. M. Arshad Javid, Dr.Qurat-ul-Ain Rashid provided us blood samples, contributed to data collection and sampling.
REFERENCES
-
Yu, François TH, and Guy Cloutier. (2007).Experimental ultrasound characterization of red blood cell aggregation using the structure factor size estimator." The Journal of the Acoustical Society of America 122.1: 645-656. https://doi.org/10.1121/1.2735805
-
Samokrutov A, Shevaldykin V, Bobrov V, Kozlov V. (2006) Development of acoustic methods and production of modern digital devices and technologies for ultrasonic non-destructive testing. Ultragarsa . Ultrasound.61(4):12-21.
-
Samokrutov AA. (2003) Modern ultrasonic instruments for the nondestructive inspection of metal products. Metallurgist. 47(7):324-9.
-
Phenix, Christopher Peter et al. (2014) "High intensity focused ultrasound technology, its scope and applications in therapy and drug delivery."
Journal of Pharmacy & Pharmaceutical Sciences 17.1: 136-153 DOI: https://doi.org/10.18433/J3ZP5F
[5 ] Calor-Filho MM, Machado JC. (2006) Measurement of the ultrasonic attenuation coefficient of human blood plasma during clotting in the frequency range of 8 to 22 MHz. Ultrasound in medicine & biology. 32(7):1055-64.-
Jimenez N, Redondo J, Sánchez-Morcillo V, Camarena F. (2015) Nonlinear ultrasound simulations including complex frequency dependent attenuation. Physics Procedia. 1;63:108-13.
-
OBrien Jr WD. (2007) Ultrasoundbiophysics mechanisms. Progress in biophysics and molecular biology. 1;93(1-3):212-55.
-
Amin, V. R. (1989). Ultrasonic attenuation estimation for tissue characterization.
-
Lyons, MARK E., and Kevin J. Parker. (1988): "Absorption and attenuation in soft tissues. II. Experimental results." IEEE transactions on ultrasonics, ferroelectrics, and frequency control 35.4. 511-521.
-
Mazzilli F, Lafon C, Dehollain C. (2014) A 10.5 cm ultrasound link for deep implanted medical devices. IEEE transactions on biomedical circuits and systems. 8(5):738-50.
-
Scheven, B. A. A., et al. (2009) "Therapeutic ultrasound for dental tissue repair." Medical hypotheses 73.4: 591-593 doi.org/10.1016/j.mehy.2009.05.032
-
Bradley E., Treeby, et al. "Measurement of the ultrasound attenuation and dispersion in whole human blood and its components from 070 MHz." Ultrasound in medicine & biology 37.2 (2011): 289-300 https://doi.org/10.1016/j.ultrasmedbio.2010.10.020.
-
Shung, Koping K., Rubens A. Sigelmann, and John M. Reid. "Scattering of ultrasound by blood." IEEE Transactions on Biomedical Engineering 6 (1976): 460-467.
DOI: 10.1109/TBME.1976.324604.
-
Zinin, P. V. "Theoretical analysis of sound attenuation mechanisms in blood and in erythrocyte suspensions." Ultrasonics 30.1 (1992): 26- 34.https://doi.org/10.1016/0041-624X(92)90028-K
-
Zohdi TI, Krone R. (2018) Estimates for the acoustical stimulation and heating of multiphase biotissue. Biomechanics and modeling in mechanobiology.1;17(3):717-25.
-
Kamimura HA, Flament J, Valette J, Cafarelli A, Aron Badin R. (2019) Feedback control of microbubble cavitation for ultrasound-mediated blood brain barrier disruption in non-human primates under magnetic resonance guidance. Journal of Cerebral Blood Flow & Metabolism. 39(7):1191-203.
-
Arshad MJ, Saddique AM, Zahid M, Niama A, Fayyaz H, Buzdar SA, Naeem MA, Imran M. (2019) Synthesis, Characterization and Blood Based Toxic Effects of Superparamagnetic Nanoparticles. Materials Science. 1;25(4):359-64.
-
Calor-Filho Marcos M, M, Machado JC. (2006) Measurement of the ultrasonic attenuation coefficient of human blood plasma during clotting in the frequency range of 8 to 22 MHz. Ultrasound in medicine & biology. 32(7):1055-64.
-
Krishna, G. Gopala, and A. Ahmad(1990). "Absorption of ultrasound in human blood." Le Journal de Physique Colloques 51.C2: C2- 311,https://doi.org/10.1051/jphyscol:1990275.
-
Mehrpour M, Shakeri-Zadeh A, Basir P, Jamei B. (2016) Effects of low-intensity continuous ultrasound on hematological parameters of rats. Journal of Biomedical Physics & Engineering. 6(3):195.
-
Salim sup, M. S., et al. (2011): "A new measurement method of separation percentage for human blood plasma based on ultrasound attenuation."
International Journal of Physical Sciences 6.30 6891-6898, DOI: 10.5897/IJPS11.1454
-
Amin, Viren R. "Ultrasonic attenuation estimation for tissue characterization." (1989).
-
Hendee, W.R. and Ritenour, E.R., 2003. Medical imaging physics. John Wiley & Sons.
-
Carstensen EL, Schwan HP. (1959) Absorption of sound arising from the presence of intact cells in blood. The Journal of the Acoustical Society of America. 31(2):185-9.
-
Bamber JC. (1986) Attenuation and absorption. Physical Principles of Medical Ultrasonics. 2:93-166.
-
Rico LG, Juncà J, Ward MD, Bradford JA, Bardina J, Petriz J. (2018) Acoustophoretic Orientation of Red Blood Cells for Diagnosis of Red Cell Health and Pathology. Scientific Reports. 24;8(1):15705.
-
Johnston, R. L., Goss, S. A., Maynard, V., Brady, J. K., Frizzell, L. A., O'Brien Jr, W. D., & Dunn, F. (1979). Elements of tissue characterization. Ultrasonic tissue characterization II, 19-27.
-
Szabo, Thomas L. Diagnostic ultrasound imaging: inside out. Academic Press, 2004 & Duck, F. A. "Physical Properties of Tissue: A Comprehensive Reference Book (London/San Diego: Academic)." (1990). https://doi.org/10.1016/C2009-0-02755-X
-
Smith D, Franco R, DiCesare CA, Schneider DK, McGill C, Smith QD, Myer GD. (2018) Red blood cell response to blast levels of force impartations into freely moveable fluid surfaces inside a closed container. Frontiers in Physics.6:93.
-
Williams AR, Sykes SM, O'Brien Jr W. (1977) Ultrasonic exposure modifies platelet morphology and function in vitro. Ultrasound in Medicine & Biology.1;2(4):311-7.
-
Treeby BE, Cox BT.( 2010) Modeling power law absorption and dispersion for acoustic propagation using the fractional Laplacian. J Acoust Soc Am;127:27412748
https://doi.org/10.1121/1.3377056.
[32 ] Lunan KD, Wen AC, Barfod ET, Edmonds PD, Pratt DE. (1978) Decreased aggregation of mouse platelets after in vivo exposure to ultrasound.Thrombosis and Haemostasis. 40(01):568-70.
[33 ] Mehrpour M, Shakeri-Zadeh A, Basir P, Jamei B. (2016) Effects of low-intensity continuous ultrasound on hematological parameters of rats. Journal of Biomedical Physics & Engineering. 6(3):195. [34 ] Kasmawan IG, Sutapa GN, Yuliara IM. (2018 ) Treatment of 40 kHz continuous ultrasound towards blood cells of mice (Mus musculus L).International Journal of life Sciences. 19;2(3):66-74.
[36] Oleshkevich, A. A. (2017). Various in vitro effects of continuous and modulated ultrasound on blood cells of different animal species. Biophysics, 62(4), 603-615.