
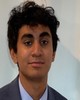
- Open Access
- Authors : Surya Mamidyala , Sundaram Thirukkurungudi
- Paper ID : IJERTV9IS090068
- Volume & Issue : Volume 09, Issue 09 (September 2020)
- Published (First Online): 10-09-2020
- ISSN (Online) : 2278-0181
- Publisher Name : IJERT
- License:
This work is licensed under a Creative Commons Attribution 4.0 International License
Investigating the Effect of the Filamentous Fungus Trichoderma Reesei on the Strength of Cracked Concrete
Surya Mamidyala Academy of Science Academies of Loudoun Leesburg, United States
Sundaram Thirukkurungudi Academy of Science Academies of Loudoun Leesburg, United States
Abstract Small cracks in concrete form due to a variety of reasons. These non-structural cracks can be amplified, however, by freeze-thaw forces, turning what were once small, harmless cracks into a safety concern. The use of a filamentous fungus, Trichoderma reesei (T. reesei), was explored in the self- healing of concrete cracks and its effect on concrete's strength. Concrete samples with model cracks of varying widths between 1mm and 2.5mm were created by placing triangular polyvinyl alcohol (PVA) wedges in the concrete mold and pouring concrete around the wedges. Potato dextrose agar (PDA) growth medium and a fourteen-day culture of T. reesei fungus were inoculated into the cracks of 14-day old concrete samples, which were then incubated for 14 days. The compressive strength of the concrete samples was measured after this 28- day period. These measures of strength were compared with the measures of strength for control groups, which were concrete samples lacking a crack, fungal inoculation, or both.
Based on the data collected and the Mann Whitney U statistical tests performed, it was found that the median compressive strength of cracked concrete samples inoculated with T. reesei were statistically higher than the median compressive strength of cracked concrete samples that were not inoculated with T. reesei for all of the crack widths between 1mm and 2mm except for the 2.5mm crack width. This supports the hypothesis and demonstrates how the inoculation of T. reesei fungus is particularly effective for increasing the strength of cracked concrete with smaller crack widths, especially in the range of 1 2mm.
Keywords Trichoderma reesei, 3D printing, cracked concrete, fungus, compressive strength, biomineralization
-
INTRODUCTION
Concrete is the material used for much of the infrastructure in the past and present [1]. With all of its advantages, however, there are some disadvantages, most notably the fact that cracks form over time. It is time- consuming and costly to fix these cracks. Cracks in concrete cost the United States more than 50 billion dollars annually to maintain, fix, and repair. These cracks are amplified by freeze-thaw cycles. When water gets inside the microcracks of concrete and freezes, it expands. When the ice melts, it leaves a larger crack in the concrete. This continues as long as the temperature fluctuates between below and above freezing points [2]. Another cause of crack formation is external stress. Tensile cracking can occur at stresses of less than 10MPa when they are concentrated around pre-existing microcracks or natural weaknesses such as pores and grain-
boundaries. This agent, combined with freeze thaw forces, can propagate smaller cracks into larger ones [3].
Cracks in concrete decrease its compressive strength. To mitigate this problem and reduce the cost, it is prudent to have a solution through self-healing. While other present research focuses on using methods such as autogenous healing [4], encapsulation of polymeric material [5], and bacterial spores to heal the cracks of concrete [6], this research focuses on using T. reesei to heal the cracks of concrete by assessing whether cracked concrete specimens with the fungus for a period of time have an improved strength compared to that of cracked concrete specimens without the fungus. Current research states that T. reesei secretes CaCO3 crystals when growing on cured concrete samples [7]. However, the actual self-healing of concrete cracks through the use of T. reesei and the effect on the concretes strength, has never actually been put to a practical test.
It was hypothesized that cracked concrete samples inoculated with T. reesei would have significantly higher compressive strength values when compared to concrete samples not inoculated with the fungus since the fungus would be able to biomineralize and precipitate calcium carbonate crystals, which would be able to fill in the concrete cracks and lead to a higher strength. To test this hypothesis, 14-day concrete samples were inoculated with the fungus and strength tested for compressive strength on the 12-ton press on the 28th day. Controls include five concrete samples lacking a crack and inoculation of fungus and twenty cracked concrete samples that were not inoculated with fungus as a negative control.
-
BACKGROUND
Currently, the three main methods of healing the cracks in concrete are autogenous healing of concrete, encapsulation of polymeric material, and the use of microorganisms such as bacteria to heal concrete.
Present research on autogenous healing of concrete finds that cracked concrete has the ability to self-heal itself in the presence of water. This was investigated by researchers from the University of Michigan in 2013 who put concrete through freeze-thaw cycles and used resonant frequency measurements to determine whether the concrete had self- healed itself. The resonant frequency change indicated the extent of the self-healing of concrete. A drop in the resonant frequency values meant that more cracking had taken place
and/or crack width had increased, while an increase in resonant frequency meant that some of the cracks had been healed or partially healed. It was found that autogenous healing brought cracked concrete between 76% and 100% of its initial resonant frequency. A significant increase in the concrete samples stiffness was recorded. The drawbacks to autogenous healing are that the crack widths must be 50m or less for autogenous healing to have significant healing behavior, and there needs to be a constant supply of water, which is often not the case in real life [4].
The encapsulation of polymeric material is another method that was tested to self-heal concrete cracks. A study conducted by the Magnel Laboratory for Concrete Research in 2015 aimed to determine whether encapsulated polymer precursors would be able to self-heal concrete. The logic behind this idea was that the capsules would rupture when a crack forms, and the polymer precursors would flow to fill the crack and harden, thereby sealing the crack. In total, 8 different polymers were tested, and it was found that the stiffness of the cracked concrete blocks regained a maximum of 30% of its original stiffness. It only had a noticeable effect for cracks with widths of 20m or less. The drawback to this method, however, was that in some situations, crack width increased by 50% to 100% because of the plastic deformation of the polymers and detachment from the crack walls. This means that it could propagate already existing cracks and have an overall detrimental effect on the concrete [5].
Clearly, due to the drawbacks of the autogenous healing and encapsulated polymers methods, a way to use microorganisms such as bacteria and fungi to self-heal concrete is desirable because they can secrete CaCO3 to heal larger cracks. Researchers from the Magnel Laboratory for Concrete Research in 2013 encapsulated bacterial spores into concrete specimens and tested whether the bacterial spores could self-heal the concrete upon cracking. The researchers found that the cracks in the concrete specimens with the bacterial spores were healed 48% to 80% as compared to those without the bacterial spores (18% to 50%), which were healed through autogenous healing. It was also found that the maximum crack width healed by th microcapsules with bacterial spores was about four times that of the microcapsules with no bacterial spores. This was most likely due to the fact that the bacterial spores secreted CaCO3to accelerate the healing process. The disadvantages, however, to using encapsulated bacterial spores were that they created more and larger pores in the concrete, which decreased its compressive strength substantially (33% to 47%) [6].
Researchers from Binghamton University in 2018 tested whether Trichoderma reesei fungi could grow well on concrete and whether it could precipitate carbonate crystals. A fungal strain was placed on concrete plates, and its growth was measured along with the minerals precipitated or lack thereof. It was found that T. reesei fungi grew at rates of 2.6 mm/day and X-ray diffraction analysis of the concrete plate found that the fungi precipitated CaCO3 on the concrete plate and the amount of formed crystals on the fungi-free control plate was much less. This research shows that fungi
has great potential to be used to heal the cracks of concrete [7].
While other present research focuses on using methods such as autogenous healing, encapsulation of polymeric material, and bacterial spores to heal the cracks of concrete, this research focuses on using T. reesei to heal the cracks of concrete and assess whether cracked concrete specimens with the fungus for a period of time have an improved strength compared to that of cracked concrete specimens without the fungus. It is significant to the field because it gives scientists and concrete manufacturers concrete evidence on whether T. reesei can actually improve the strength of cracked concrete.
-
MATERIALS AND METHODS
Trichoderma reesei (ATCC 13631) was purchased on the ATCC website and immediately stored in a freezer at – 80 degrees Celsius to prevent the ampoule from drying out. The recommended procedure for freeze-drying ampoules listed in the product sheet for the organism was then followed to obtain a fungal suspension. Four to five drops of the suspension were added to a petri dish containing dehydrated PDA purchased from BD BiosciencesTM. T. reesei was cultivated on the PDA growth medium for 14 days in a Quincy Lab 12-140 Incubator at 25 degrees Celsius. A CAD model of a 2 in3 concrete mold with each side having a thickness of 2mm was created (Fig. 1). The Ultimaker 3.5 3D printer was used to print 45 of these concrete molds using the polylactic acid (PLA) filament at 20% infill and 0.8mm nozzle width. CAD models of triangular wedges with a length of 2in, width that was specific to the crack width (1mm, 1.5mm, 2mm, or 2.5mm), and a depth of 1in were created (Fig. 2). Ten triangular wedges were 3D printed on the Series 1 3D printer using PVA filament at 20% infill and 0.4mm nozzle width for crack widths of 1mm, 1.5mm, 2mm, and 2.5mm, so in total, 40 triangular wedges were printed.
Eighty pounds of Sakrete 4000 PSI High-Strength Concrete Mix was then mixed with 3.7 liters of water in a wheelbarrow using a welded garden hoe until an appropriate slump was achieved so that it has a desirable water to concrete ratio. The insides of the 45 printed concrete molds were spray- coated with Kleen Kote water-based industrial concrete non-sticking agent so that once the concrete was poured and had hardened, it could easily be removed (Fig. 3).
Fig. 1. CAD model of PLA mold
Fig. 2. CAD model of 2.5mm PVA triangular wedge
Fig. 3. Photo of concrete samples after being taken out of the mold
Fig. 4. Concrete samples being boiled to dissolve PVA wedges
The 3D printed wedges were placed in the mold so that it connected the median points of two parallel sides and had a depth that went halfway down the mold (1 in). In addition, the wedge lied perfectly flat along the surface of the mold so that when concrete was poured in the mold, there was no piece of the wedge remaining above the concrete. Five of the molds did not have any wedges, but the other 40 molds were split into four different groups, each group having wedges with a similar crack width. The concrete liquid mixture was then poured into the concrete molds around the wedges (for the molds with wedges) and directly into the molds for those without wedges.
After approximately 7 days, the concrete samples were taken out of the molds and the 40 concrete samples with the wedges were placed in a large cooking tray filled with tap water. The cooking tray was placed on top of two hot plates that were set to the maximum heat setting and covered with aluminum foil for approximately 1 hour. Since the wedges were made out of PVA filament, which is water-soluble, the wedges readily dissolved in the boiling water (Fig. 4). This resulted in an artificial and consistent crack formation in the concrete samples. Seven days after the cracked concrete samples were prepared, they were inoculated in the center of their respective cracks with 5.5mm of the mycelial disk of a 14-day culture of Trichoderma reesei collected with an Ajax Scientific Cork borer of the same radius and 10mL of liquid PDA growth medium. The concrete samples were incubated for 14 days in a Quincy Lab 12-140 Incubator at natural daylight conditions at 30 degrees Celsius (Fig. 5).
Before strength testing the concrete samples on the 12- ton press, a ballistic shield was built and placed on the outside of the 12-ton press using metal frames and a rectangular prism of thick plexiglass to prevent the user from injury. Twenty-eight-day concrete samples were strength- tested on the 12-ton press by placing individual concrete samples on the press bed frame over two adjacent metal heel blocks. First, the release valve was turned clockwise until it was firmly closed. A steel rectangular flange (2 in x 2 in x
0.5 in) was used as a pad to cap the
Fig. 5. Concrete samples being incubated in natural daylight conditions
concrete sample to assure uniform distribution of load during testing. The ram head was lowered to barely touch the flange using the hydraulic system of the 12-ton press. The concrete sample was re-aligned and centered under the ram head to ensure center-loading. If the sample had a crack, it was positioned so that the mouth of the crack was horizontal and facing the front of the 12-ton press. The handle was pumped to apply increasing load to the concrete sample until it structurally fractured (Fig. 6). A video was taken of the hydraulic pressure gauge and the breaking load of the concrete sample was recorded for a) the concrete sample without crack, b) the concrete sample with crack lacking inoculation of T. reesei, and c) the concrete sample with crack inoculated with T. reesei. The release valve was turned counterclockwise in small increments to retract the ram from the workpiece. The process for measuring the breaking load was repeated for all 45 concrete samples.
The median compressive strengths of the cracked concrete samples inoculated with T. reesei and cracked concrete samples lacking T. reesei were compared for each crack width. Statistical significances were determined using a Mann-Whitney U test since it was not known whether the distribution of concrete strength was normal and there were relatively few data points (five points) for each group, so it would be generally more accurate and safer to use medians than means since they are unaffected by outliers.
Fig. 6. Concrete samples being strength-tested on the 12-ton press
-
RESULTS OF CONCRETE STRENGTH-TESTING
TABLE I.
Compressive Strength of Concrete (in PSI) for Concrete Samples Differentiated by Crack Width and Presence of Fungus
Concrete Sample #
No Crack No Fungus
1mm Fungus
1mm No Fungus
1.5mm Fungus
1.5mm No Fungus
2mm Fungus
2mm No Fungus
2.5 mm Fungus
2.5mm No Fungus
1
2756.00
1100.00
1000.00
1000.00
850.00
700.00
550.00
600.00
900.00
2
600.00
1000.00
950.00
1000.00
450.00
900.00
800.00
750.00
950.00
3
1500.00
1100.00
950.00
1450.00
800.00
1150.00
650.00
800.00
400.00
4
1300.00
1300.00
1000.00
1100.00
800.00
1050.00
400.00
1100.00
600.00
5
600.00
1100.00
1000.00
1050.00
950.00
1000.00
850.00
1050.00
600.00
Mean Strength
1351.20
1120.00
980.00
1120.00
770.00
960.00
650.00
860.00
690.00
Standard Deviation
790.801
97.980
24.495
169.115
169.115
152.971
164.317
188.149
205.913
Standard Error
353.657
43.818
10.954
75.631
75.631
68.411
73.485
84.143
92.087
TABLE II.
P-values and Test Statistics for Mann Whitney U Statistical Test based on Compressive Strength of Concrete
Test Statistic for Mann Whitney
P-value
1mm Crack Width
2.412
0.016
1.5mm Crack Width
2.655
0.008
2mm Crack Width
2.148
0.032
2.5mm Crack Width
1.016
0.310
-
SAMPLE CALCULATIONS
CS = 2000 * BL / A (1)
In (1), CS is the compressive strength of the concrete (in PSI), BL is the breaking load of the concrete (in US tons), A is the cross-sectional area of each concrete sample in in2, and 2000 is a conversion factor to convert US tons to pounds.
-
LIMITATIONS AND SOURCES OF VARIATION One main source of variation in the experimental setup
was the fact that the wedge was not in a fixed position during the process of curing, which led to the movement of the wedge, making the cracks not identical in all samples of same width. However, the variation was not significant because the average width of the crack across the concrete
samples was the same. The standard error in the measurements for the samples of same crack width can be reduced if this variation is avoided. A second significant source of variation was centering the concrete samples when strength testing under the 12-ton press. In some cases, it was difficult to determine whether the sample was centered under the pressing ram head. This error was mitigated due to the use of the steel flange on the top of the concrete sample. In addition, the perpendicular alignment of the top concrete sample to the ram head was consistent. Finally, the uniformity of the concrete mix could also be seen as a source of variation. Since the concrete was hand-mixed, the consistency in the amount of aggregates in each sample varied, causing variation in compressive strengths for samples of identical cracks. This could be easily fixed in future research by using a concrete mixing machine.
-
ANALYSIS OF RESULTS
The original hypothesis was that cracked concrete samples with T. reesei would have a higher median compressive strength values when compared to their cracked counterparts not inoculated with T. reesei. The hypothesis appears mostly correct from an analysis of the data.
For example, a visual analysis of results table 1 reveals that for each of the different crack widths, cracked concrete samples inoculated with the fungus had higher mean compressive strengths when compared to their counterparts without fungus. This was especially true for the smaller crack widths of 1mm, 1.5mm, and 2mm because the difference in the mean compressive strength for these crack widths for samples inoculated with fungus and samples
lacking fungus was 140PSI, 350PSI, and 310PSI respectively. This supports the hypothesis and demonstrates how the inoculation of T. reesei fungus is particularly effective for increasing the strength of cracked concrete with smaller crack widths, especially in the range of 1 2mm. This could be due to the fact that for larger crack widths, since the same amount of fungus was inoculated for each of the different crack widths, there was not enough biomineralization of the crack in proportion with the size of the remaining unfilled crack to cause the strength of the concrete to increase significantly.
A Mann Whitney U Statistical Test was completed for each of the different crack widths, comparing the compressive strengths of samples inoculated with fungus and those not inoculated with fungus and the results of the test are presented in Table II. For three of the four Mann Whitney U statistical tests run, for the crack widths of 1mm, 1.5mm, and 2mm, the p-values received were 0.016, 0.008, and 0.032. Since these p-values were all less than the significance level of 0.05, there is statistically significant evidence to conclude that there is in fact a difference between the median compressive strengths of cracked concrete samples inoculated with fungus and the median compressive strength of cracked concrete samples not inoculated with fungus for the 1mm, 2.5mm, and 2mm crack widths. Since every other facet of the experiment including preparation of concrete samples, curing environment, and artificial crack formation were controlled for, the only logical explanation for the significantly higher increase in strength for fungus treated cracked concrete samples is T. reeseis ability to biomineralize and help fill in the concrete crack.
Future studies would determine the elastic limit, which would be more beneficial since it would give the stress value of permanent deformation for a concrete sample and it would seem to be more affected by the presence of the crack. Even though there was some movement of the wedge during curing, this source of variation did not significantly affect the results of the experiment because the distance that the wedge moved from the blocks center (<0.2in) is very small compared to the overall width of the block (2in). In addition, even with the source of variation, concrete samples prepared with the same wedge had approximately equal crack widths. The same line of reasoning could be applied to the other source of variation, which was centering the concrete sample under the ram head. Since the distance the concrete sample was off centered under the ram head (<0.04in) is significantly less than the width of the concrete block (2in), the source of variation had very little effect on the results of the experiment. In addition, the source of variation was mitigated by the steel flange, which helped uniformly distribute the load of the 12-ton press on each concrete sample.
-
CONCLUSION AND FUTURE WORK
The method of self-healing of concrete tested in this research with the filamentous fungus Trichoderma reesei has been found to significantly increase the compressive strength of concrete samples with crack widths of 1, 1.5, and 2mm. Despite the previously discussed sources of variation, the healing strategy using Trichodrma reesei has shown
marked improvement over other forms of self-healing since they only effectively self-healed concrete samples with cracks widths up to 0.5mm.
As for future work, incubation time of T. reesei in the cracked concrete samples and its effect on the strength of concrete samples could be tested. Additionally, the volume of T. reesei inoculated into cracked concrete samples could be tested for its impact on strength of concrete samples. To minimize the effect of the uneven distribution of aggregates in concrete, mortar could be tested instead since it is concrete without the aggregates. Another avenue of research could be testing the effect of T. reesei on cracked reinforced concrete samples since it is yet to be tested how well the fungus would grow in that environment and whether it would have any adverse reactions with the steel bars in reinforced concrete. Additionally, even though T. reesei is a BSL 1 fungus and it is a known non-pathogen to humans, it is important to conduct a thorough investigation of the environmental impacts of T. reesei. Finally, the crack shape and other crack widths could be tested, as well as different variants of concrete.
ACKNOWLEDGMENT
I would like to sincerely thank Mr. Paul Coates, the Masonry teacher at the Academies of Loudoun, for helping me with my project by guiding me with the process of making concrete blocks and removing the concrete blocks from their molds. I would also like to thank Kieran Lynch, a student at the Academies of Loudoun, for his idea to incorporate water-soluble PVA filament in the creation of the wedges. Additionally, I would like to thank Dr. Julie Sohl, a research teacher at the Academies of Loudoun for allowing me to use her lab to culture and incubate the fungus. Finally, I would like to thank Mr. Josh Ajima, the Academies of Loudoun, and their administrators for providing me access to cutting edge facilities like the Makerspace and Masonry Lab.
REFERENCES
-
Highways and Bridges. (2013). Retrieved from https://www.nace.org/Corrosion-Central/Industries/Highways-and-
Bridges/
-
CFA: Concrete Cracking Overview. (2018). Retrieved from http://www.cfawalls.org/foundations/cracking.htm
-
Macroscopic Aspects of Rheology. (1997). Retrieved from http://www.geosci.usyd.edu.au/users/prey/ACSGT/EReports/eR.200 3/GroupE/Report1/tensile_cracking.html
-
Yang, Y., Lepech, M. D., Yang, E., & Li, V. C. (2013). Autogenous healing of engineered cementitious composites under wetdry cycles. Cement and Concrete Research, 39(5), 382-390. doi:10.1016/j.cemconres.2009.01.013
-
Feiteira, J., Gruyaert, E., & Belie, N. D. (2016). Self-healing of moving cracks in concrete by means of encapsulated polymer precursors. Construction and Building Materials,102, 671-678. doi:10.1016/j.conbuildmat.2015.10.192
-
Wang, J., Soens, H., Verstraete, W., & Belie, N. D. (2014). Self- healing concrete by use of microencapsulated bacterial spores. Cement and Concrete Research,56, 139-152. doi:10.1016/j.cemconres. 2013.11.009
-
L Luo, J., Chen, X., Crump, J., Zhou, H., Davies, D. G., Zhou, G., & Jin, C. (2018). Interactions of fungi with concrete: Significant importance for bio-based self-healing concrete. Construction and Building Materials,164, 275-285.
doi:10.1016/j.conbuildmat.2017.12.233