
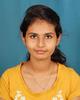
- Open Access
- Authors : Surya Lawrance , Karthika B. S , Logashree K , Kalairoja K, Nithiyanantham K. K
- Paper ID : IJERTV10IS060255
- Volume & Issue : Volume 10, Issue 06 (June 2021)
- Published (First Online): 28-06-2021
- ISSN (Online) : 2278-0181
- Publisher Name : IJERT
- License:
This work is licensed under a Creative Commons Attribution 4.0 International License
Landing Gear Stress Analysis During Rough Landing
*1 Nithiyanantham K.K, #1 Surya Lawrance, #2 Karthika B.S, #3 Logashree K, #4Kalairoja K
*1Assistant Professor (Sr Scale), #1, #2, #3, #4UG Student,
*1, #1, #2, #3, #4Department of Aeronautical and Aerospace engineering, KCG College of Technology, Karapakkam.
Abstract:- Landing gear is the undercarriage of an aircraft or spacecraft, and is used in both take-off and landing. The landing gear shock absorber is an integral component of an aircrafts landing gear. The role of the shock absorber is to absorb and dissipate energy upon impact, such that the forces imposed on the aircrafts frame are tolerable. The shock absorber may be an independent element, or integrated with the landing gear strut. The aircraft may tend to land in a smooth manner or even in a rough manner. The landing gear components must be able to withstand the entire force.
The aim of this paper is to develop a landing gear which is suitable for rough landing too. This is achieved by creating a 3-D model of the Landing gear in CATIA V5 software and subject it to ANSYS to determine the variation in the displacement and Von miss stress behaviour for three materials (steel, Aluminium, Titanium) which is subjected to static loading and plot the results for same loading condition and suggest the suitable material. And then the landing gear is modelled and the modelled component is analysed using ANSYS Software to study its structural performance thereby replacing the material of the landing gear.
Keywords:- Landing gear, Shock absorber, Loads, CATIA V5, ANSYS, Von miss stress
characteristics of the gas produce a pronounced nonlinear spring rate, the slow, isothermal compression curve. During rapid stroking of the shock, hydraulic pressure due to viscous damping across the orifice becomes dominate, giving the strut the ability to react large dynamic loads. Often a second orifice and hydraulic chamber is used to meter the stroke velocity in the opposite (extending) direction.
-
The Design Decision
First step in design process is to decide about internal chamber arrangement and overall size of the piston/cylinder combination, followed by deciding pressure settings and damping characteristics. An efficient landing gear design balances the size and complexity of the landing gear with the load attenuation ability of the gear. This project will consider only a single landing gear, not a full aircraft. As such, aerodynamic effects, and body pitch and roll will not be explored. Also, other landing gear functions such as steering, braking, and retraction/extension for stowage will not be considered, other than how they may affect the mass of the components. [3].
-
INTRODUCTION
An aircraft landing gear shock absorber is a combination of mechanical structure, pneumatics and hydraulic damping used for transmitting impact loads. This project focuses energy based modelling of the shock absorber. A landing gear shock absorber model includes an aircraft mass with
wing lift, shock absorber piston and cylinder structure, three hydraulic chambers representing the air chamber, hydraulic chamber, and rebound chamber, and tire. The design objective is to minimize ground reaction loads. The key design variables are centred on fixed or variable orifice definitions. [1]
-
THE DESIGN DECISION SITUATION
A. System Description
This paper is aimed at design problem. It is centred in the hydro-mechanical domain. [2]. The majority of landing gear shock struts are comprised of a piston and cylinder, pressurized by compressed nitrogen, using hydraulic fluid as the damping medium. The hydraulic chamber is normally separated from the air chamber by an orifice. This orifice may be fixed, or vary with stroke (that is, travel of the piston) or vary with pressure. Other arrangements are often employed, including dividing the air chamber into a low and high pressure volume to provide more complex (and tailor able) compression characteristics. An aircraft is supported entirely on air springs. Compression
-
DEVELOPMENT
The overall fundamental objective is to maximize the landing gear performance. It is basically divided into four fundamental objectives. [4]. The focus is on the energy absorption performance aspects
-
Minimize Landing Loads
-
Minimize Taxi Loads
-
Minimize Shock Absorber Volume, L x D2/4
-
Maximize Composite Reliability and Maintainability
-
-
SIMULATION
-
Model Requirements
-
Fixed inputs are those intend to code into the model to represent physical features, but do not intend to explore for design. [5].
These include:
-
Tire characteristics (stiffness, stroke length),
-
Fluid characteristics (hydraulic oil)
-
-
Design variables are parameters (varied in comparative analysis).
These are:
-
Stroke length, S, cm
-
Piston diameter, D, cm
-
Shock pressure, P, kPa
-
Shock volume, further divided into air volume, Va, and fluid volume, Vf, cm*3
-
Orifice characteristics, (units tbd)
-
-
Variation variables will be used to explore randomness or uncertainty in the model:
-
Friction (piston to cylinder)
-
Gas compression characteristics (real gas or ideal gas).
-
-
Usage variables are parameters (varied to exercise the model in realistic usage scenarios).
These will include:
-
Aircraft mass: Only a proportional amount of mass will be applied to the mode
-
Aircraft forward speed, m/sec
-
Vertical speed (landing cases only), m/sec
-
Bump profile (height as a function of distance).
-
-
-
Assumptions and Simplifications
-
Model rigid structure for the piston and cylinder, neglecting bending and expansion under load
-
Will model a single strut with 1-D motion
-
Neglect gas-fluid mixing
-
Assume constant fluid properties
-
Neglect high frequency dynamic behaviour.
-
-
-
LANDING GEAR DESIGN
The Landing Gear was modelled with standard dimensions derived from various literature surveys. By using that dimensions, the parts of Landing Gear was modelled. After all individual part creation, assembly of all parts is done to create a single component. The assembled model of the landing gear is shown in fig.1. The three view diagram and isometric diagram of the model is shown.
-
Material Selection
In general the landing gear is made of metal substrate which is a Titanium alloy Ti-6 Al 4V. The SAE 1035 steel and 7075-
76 aluminium alloy is replaced to improve the structural behaviour of the Landing Gear assembly. [6]. The properties of these materials are listed in below Table:1.
Table: 1 Material property
Property
Ti-6 Al 4V
Titanium Alloy
SAE 1035
Steel
7075-76
Aluminium alloy
Density
7.87 g/cm3
4.43
g/cm3
2.81 g/cm3
Youngs Modulus
196 GPa
113.8
GPa
71.7 GPa
Coefficient of Thermal Expansion
11.9 µC-1
8.6 µC-1
23.6 µC-1
Reference Temperature
20°C
20°C
20°C
Poissons Ratio
0.29
0.342
0.33
Tensile Yield Strength
550 MPa
880
MPa
503 MPa
Tensile Ultimate Strength
620 MPa
950
MPa
572 MPa
-
Geometry Creation
In this process, geometry for the Landing Gear assembly is created. The Landing Gear and its components are modelled earlier which is now a 3D model. The Landing Gear assembly has to be imported as an IGS file format to get a proper structure. The imported Landing Gear assembly model is as shown in figure.2.
Fig.1: Assmbled model of Landing gear
-
Meshing
Fig.2: Piston assembly
-
-
NUMERICAL ANALYSIS
-
FEA:
Finite Element Analysis (FEA) uses numerical methods and algorithms to solve and analyse problems that involve analysis of structures or objects using computers.
-
Outline Finite Element Analysis Process
The three basic elements are
-
Pre-processor ,
-
Solver ,
-
Post-Processor
Different meshing characteristics were used for the domain. In the simulations, the piston component surfaces were discretized with triangular mesh elements.
-
Boundary Condition
The problem is a structural analysis problem. The boundary condition for the problem is Force of 10 KN with the arm to be fixed.
-
Numerical Results
The structural behaviour of the Landing Gear has been studied for the above mentioned boundary conditions. The results obtained by these are shown in below. The Table. II shows the structural behaviour of the Landing Gear assembly. From the table its clear that the SAE 1035 Steel material holds a very
good structural property. The stress concentration over the SAE 1035 Steel is more than the Titanium alloy 6A1-4V and 7075-76 Aluminium alloy.
Table: 2 Structural Behavior
Loading Condition @ 10000 N
Deformation(m m)
Stress(Mpa)
Strain
Titanium alloy 6A1-4V
1.7734
232.03
0.00211
7075-76
Aluminum alloy
2.8162
232.7
0.00336
SAE 1035
Steel
1.0318
235.05
0.00125
-
-
SIMULATION OUTCOMES
Fig.3: Deformation for case-1
Fig.4: Deformation for case-2
Figure.5: Deformation for case-3
From figure 3, 4, and 5, the values of deformation for three cases were observed. The deformation contour clearly shows that the deformation is high for the aluminium alloy when compared to other two materials. The SAE 1035 holds a less deformation even blow that of titanium. So in terms of deformation the SAE 1035 holds a better performance than the other two materials.
Fig.6: Stress for case-1
Figure.7 Stress for case-2
Figure.8 Stress for case-3
From figures 6, 7 and 8, the value of stresses are determined. The stress concentration over the SAE 1035 is higher than the other cases. The SAE 1035 holds a higher yield value than the other materials. So the higher stress doesnt affect the SAE 1035, so in terms of stress concentration over the model its recommended to us SAE 1035 material.
Fig.9: Strain for case-1
Fig.10 Strain for case-2
Fig.11: Strain for case-3
From figures 9, 10 and 11, the value of strain are observed. Since the stress is directly proportional to strain the performance of the strain also will be better for the SAE 1035 material. So in terms of all structural parameters the SAE 1035 material holds a good performance.
-
CONCLUSION
The landing gear is modelled and assembled using SOLIDWORKS. The assembled CAD model has been considered to perform structural analysis by finite element approach using the ANSYS package. In general the accuracy of the solution depends on the mesh quality. Each component has been checked for its mesh quality to ensure the solution accuracy. Also before the meshing process model has been simplified. After these processes the structural analysis has been carried for the landing gear assembly for three different materials Titanium alloy 6A1-4V, 7075-76 Aluminium alloy and SAE 1035 Steel. The results show that the SAE 1035 steel holds a good performance when compared to other materials. The result has been compared on the basis of the parameters like deformation, stress and strain. The SAE 1035 steel has a less deformation when compared to other materials; around 35
% of the deformation has been reduced when compared. So the implementation of this material would help to avoid the landing gear damage and also it can have a better life than the other materials due to its less damage.
-
REFERENCE
-
Kuldip Ganorkar1, Prof. Vishal Deshbhratar, Design optimization of Landing Gear of an Aircraft – A Review, IOSR Journal of Mechanical and Civil Engineering (IOSRJMCE) e-ISSN: 2278-1684, p-ISSN: 2320-334X , PP 01-04
-
Aravind , Saravanan, Mohammed D Rijuvan, Structural Analysis Of Landing Strut made up of carbon fiber composite material, International Journal of Mechanical and Production Engineering,
ISSN: 2320-2092, Volume-1, Issue-1, July-2013
-
Achuth Rao, Realistic Simulation of a Flexible Realistic Simulation of a Flexible Mechanism using ANSYS Solutions Mechanics using ANSYS Solutions, NAFEMS World Congress, Vancouver, Canada,
May 22nd 25th, 2007
-
A, Abhay B T , Design and Analysis Aircraft Nose and Nose Landing Gear, Rajesh The International Journal Of Engineering And Science (IJES), Volume- 4, Issue-10, Pages-PP -74-80,2015, ISSN (e): 2319 1813 ISSN (p): 2319 1805
-
Janardhan B Hunusnale, C. Daksheeswara Reddy, Dr. P H V Sesha Talpa Sai, Fatigue Analysis of Piston Rod Used In Aircraft Nose Landing Gear, International Journal & Magazine of Engineering, Technology, Management and research, ISSN No: 2348-4845,
Volume No:2 (2015), Issue No:7 (July), Page 1701-1708
-
James N. Daniels, A Method for Landing strut Modeling and Simulation with Experimental Validation, NASA Contractor Report 201601, 1996. Norman, S. C, Aircraft Landing strut Design: Principle and Practices, AIAA Education Series, AIAA, Washington, D.C., 1988.
-
-
ACKNOWLEDGEMENT
-
We thank our management and our Principal Dr. P. DEIVASUNDARI for the opportunities given to us for our career development.
We feel indebted to the Head of the Department Dr. K. VIJAYARAJA, Department of Aeronautical and Aerospace Engineering, KCG College of Technology, for all his encouragement, which has sustained our labor and efforts. We express our deepest gratitude to our internal guide Mr. NITHIYANANTHAM. K. K. Assistant Professor, Department of Aeronautical and Aerospace Engineering, KCG College of Technology, for his valuable guidance, ideas and support.
We would like to thank all other faculty members for their help and advice throughout our life in this campus.
Finally, we are thankful to all our friends and all others who encouraged us and helped us in doing this paper.