
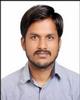
- Open Access
- Authors : Nagendra Rathore , Maheedhar Dubey
- Paper ID : IJERTV10IS050391
- Volume & Issue : Volume 10, Issue 05 (May 2021)
- Published (First Online): 05-06-2021
- ISSN (Online) : 2278-0181
- Publisher Name : IJERT
- License:
This work is licensed under a Creative Commons Attribution 4.0 International License
Modelling of a Multi-array PV Grid System with Energy Storage Systems
Nagendra Rathore
Dept. of Electrical Engg., Vishwavidyalaya Engineering College Lakhanpur,
CSVTU, Bhilai, Chhattisgarh, India.
Maheedhar Dubey
Dept. of Electronics Engg., Vishwavidyalaya Engineering College Lakhanpur,
CSVTU, Bhilai, Chhattisgarh, India.
Abstract – The multi-array grid connected photovoltaic (PV) system studied in the paper includes parallel arrays and power electronic units, each with their own DC-DC and a common DC/AC converter. The proposed configuration can not only boost the usually low photovoltaic (PV) array voltage, but can also convert the solar dc power into high quality ac power for feeding into the grid, while tracking the maximum power from the PV array. Grid voltage-oriented control is employed for VSI inverters and a battery is incorporated for energy storage and performance improvement. Modeling is performed with the MATLAB / Simulink software, such that both the power electronics components, controls and subsystem aspects, and the electric grid power system issues, can be studied during steady-state and transient operation.
Keywords: Photovoltaic systems; power converters; transformer- less; multi-phase inverters
-
INTRODUCTION
Renewable energy generation is friendly power option and which is quick creating and sunlight based photovoltaic (PV) frameworks have outperformed record establishments lately. Writing on PV related themes covers procedures for most extreme force point following (MPPT), which were as of late surveyed for instance in, power quality upgrades and explicit regulators and explicit force electronic circuit geographies for DC-DC converters, DC-AC inverters, and single stage power converters [1]-[2]. As the irradiance disparity between PV boards associated in arrangement may restrict the force yield, the focal point of a portion of the distributed exploration is on exhibit reconfiguration to adjust impacts. Different papers propose module incorporated converters, to such an extent that the MPP for every module can be followed, yielding most extreme energy extraction. Module coordinated DC/DC converters associated with a focal DC-AC converter were proposed [3].
These days, sustainable power has been increasingly more alluring because of the extreme ecological assurance guidelines and the deficiency of regular fuel sources. Photovoltaic CPV) age is the strategy which utilizes photovoltaic cell to change sun-oriented energy over to electric energy [4]. Photovoltaic energy is expecting progressively significant as an environmentally friendly power source due for its unmistakable potential benefits, like basic arrangement, simple assignment, liberated from contamination, low support cost, and so forth Nonetheless, the detriment is that photovoltaic age is discontinuous, contingent on climate conditions. Hence, energy stockpiling component is important to help get steady and dependable
force from PV framework for burdens or utility lattice, and along these lines improve both consistent and dynamic practices of the entire age framework [5]-[6]. On account of it develop innovation, minimal expense and high productivity, battery energy stockpiling framework (BESS) is utilized broadly in conveyance age innovation. BESS can be coordinated into PV age framework to shape a half breed PV/Battery age framework, which can be steadier and more dependable. A basic network associated PV/Battery age framework is made out of PV exhibit, battery, power electronic converters, channels, regulators, nearby loads and utility lattice [7].
The current paper talks about a topology of a multi- array associated sun energy-based PV installations in coordinated battery energy stockpiling. The PV framework under examination is isolated into a few areas, and each part has its own DC-DC converter and a two-level DC-AC inverter with matrix arranged voltage control as found in continuing segments. In the proposed plan, the two-level inverters are fell to acquire a voltage waveform practically identical with that would be accessible from a staggered converter [8]-[9]. The framework and its controls are recreated on a two individual PV power framework utilizing the MATLAB/Simulink programming. A battery energy stockpiling framework (BESS) is utilized to give capacity to the lattice if there should arise an occurrence of fractional or complete concealing after a proposed control plot as per which if the battery's condition of charge is lower than the base permitted the nearby coordinated generators will supply the force deficiency.
-
PROPOSED SYSTEM CONFIGURATION
In grid-connected inverters for PV applications, a number of different approaches have been developed and used over the last 20 years. An excellent review of such systems available in many Literature review [10]. Only the two or more common approaches used in smaller residential scale installations.
For high power applications, it is common to divide the PV plant into several sections as seen in in Fig.1. In each section, the PV array is connected to a buck converter, which is used to maintain the PV voltage at its maximum power point. It is reported that a buck converter has an advantage over other DC-DC converters when there are multiple strings connected in the array. The output of the buck converter is connected to a central inverter, which is typically a two-level inverter. The power circuit diagram of one unit is shown in Fig. 1. The battery energy storage
system (BESS) is interfaced with the system such that the
= (
) [
+ (
)] (2)
battery supplies power to the grid through its own inverter
,
when there is loss of power due to shading of the PV panels [11]-[12].
where, S is the real solar radiation (W/m2); Sref ,Tref , Iph,ref is the solar radiation, cell absolute temperature, photo current in standard test conditions respectively; CT is the
PV array – I
MPPT Controller
temperature coefficient (A/K).
Diode saturation current varies with the cell temperature;
DC / DC
Converter
DC / AC
Converter
3 1 1
= ,
( )
[ ( )](3)
Vdc
BESS
DC /AC
where Is,ref is the diode saturation current in standard test conditions; Eg is the band-gap energy of the cell semiconductor (eV), depending on the cell material[14]-[15].
Parameters
Value
Solar Irradiance; Gref
1000 W/m2
Cell temperature; Tref
25ºC
Cell per each module
36
No. of modules connected in Parallel
10
No. of modules connected in Series
10
Open circuit voltage; Voc
64.8 volts
Short circuit current; Isc
5.96 Amps
Maximum voltage; Vm
54.7 volts
Maximum current; Im
5.58 Amps
Temperature co-efficient
6.175%
Parameters
Value
Solar Irradiance; Gref
1000 W/m2
Cell temperature; Tref
25ºC
Cell per each module
36
No. of modules connected in Parallel
10
No. of modules connected in Series
10
Open circuit voltage; Voc
64.8 volts
Short circuit current; Isc
5.96 Amps
Maximum voltage; Vm
54.7 volts
Maximum current; Im
5.58 Amps
Temperature co-efficient
6.175%
Converter
TABLE I: PARAMETERS OF A SINGLE PV MODEL
PV array – II
Vdc
MPPT Controller
Utility Grid
DC / DC
Converter
DC / AC
Converter
Fig. 1 Proposed multi-array PV connected grid system.
-
PV Array
Photovoltaic cell is the most basic generation part in PV system. Single-diode mathematic model is applicable to simulate silicon photovoltaic cells, which consists of a photocurrent source Iph a nonlinear diode, internal resistances Rs and Rsh. as shown in below fig.2.
I
With different temperatures and solar radiations, output characteristics of PV array are simulated as fig. 3 and fig. 4
Rs
Iph D Rsh Vpv
Fig. 2 Single-diode mathematical PV model
The mathematic relationship for the current and voltage in the single-diode equivalent circuit can be described as:
= (
(+)
1)
+
(1)
where, Iph is photocurrent; I, is diode saturation current; q is coulomb constant (1.602e·19C); k is Boltzman's constant (1.38Ie-23 J/K); T is cell temperature (K); A is P-N junction ideality factor; R and Rsh are intrinsic series and shunt resistances [13].
Photocurrent is the function of solar radiation and cell temperature, described as:
Fig. 3 Characteristics curves of a single PV module with different temperature
differential change in power (P) of PV, then the subsequent perturbation is generated in the same (opposite) direction [18]. The variation of duty cycle fed to dc chopper is varied until the maximum power point has been achieved. This variation produces oscillation in system which can be minimized by reducing the step size of perturbation. The various values of irradiance and cell temperatures, the PV array exhibit different characteristic PV curves having different maximum power point. The voltage value at point where maximum power corresponds maximum voltage in
the curve is supplied to the DC-DC converter for its operation. The below figure depicts the P&O MPPT algorithm.
Start P&O Algorithm
Measure V(k), I(k)
P(k) = V(k)*I(k)
P = P(k) P(k-1)
Fig. 4 Characteristics curves of a single PV module with different solar irradiation
As shown in figure 3 and 4, PV array has nonlinear voltage-current characteristics, and there is only one unique operating point for a PV generation system with a maximum output power under a particular environmental condition.
Yes
No
V(k) – V(k-1) > 0 No
P > 0
Yes
No
V(k) – V(k-1) > 0
Yes
-
MPPT Algorithm P&O
The variation of the irradiance level, panel temperature and IV characteristic of a PV array, led to variation in MPP, (as it is the function of the irradiance level, ambient temperature, efficiency of the heat exchange process and operating point of the panels). It makes necessary to track
Increase PV Voltage
Decrease PV Voltage
Decrease PV Voltage
Decrease PV Voltage
Update V(k-1) = V(k)
P(k-1) = P(k)
Increase PV Voltage
continuously the MPP to maximize the power output from a PV system, for a given set of operating conditions [16]. The maximum power point tracking (MPPT) can be achieved by different approaches like fuzzy logic, neural networks, pilot cells and DSP based implementations which have been proposed in [17].
A regular solar PV panel has an efficiency of 30 to 40 percent conversion of the incident solar radiation into electrical energy. Hence MPPT techniques are used to improve the PV panel efficiency. In accordance to Maximum Power Transfer theorem, the power output of a circuit is maximum when the source impedance of the circuit matches with the load impedance so by this concept the maximum power point reduces to an impedance matching problem. Hence, MPPT algorithms are used in PV system to maximize power extraction under all atmospheric conditions because of its ease of implementation.
In P&O method, the MPPT algorithm is based on output power the calculation of the PV and change in power by sampling both the PV Array current and voltage. The tracker observes change in voltage (V) and the voltage is incremented or decremented periodically of the PV panel. If the perturbation leads to an increase (decrease) in
Fig. 5 Flowchart of P&O MPPT algorithm
-
Grid-connected Inverter
Grid-connected PV generation system is mainly composed of the PV array, the inverter device with the function of maximum power tracking and the control system. The inverter device with the function of maximum power point tracking can inverse the electric power into sinusoidal current, and connect to the grid [19]. The control system mainly controls the maximum power point tracking of photovoltaic, current waveform and power of the output of grid-connected inverter, which makes the output to the grid correspond with the export by PV array.
Voltage Source Inverter control method
The PV array's working voltage is set to Vo, the standard voltage Vref should be matched with the working voltage Vo when the PV array is in the maximum power output state. The standard current Iref should be kept to sinusoidal while the power factor should be kept to one which can be realized by PWM control method. Sw is a switch which mainly protects the inverter, and cuts the inverter from the system when the system power off [20]. The voltage source inverter and its control method are shown in below fig. 6.
PV
Array
&
DC
—— DC
Side
S1 S5 S3
Vdc
S2 S6 S4
AC Mains
battery voltage that represents the minimum state of charge. The charging current controller outputs a current component corresponding to the amount of power required to increase the battery terminal voltage to a value that represents the maximum state of charge. The net battery current is derived as the sum of discharging and charging currents. The reactive power component Id for this design was maintained at zero so that the battery does not supply reactive power, this component can also be varied.
Vdc
S1 S6
PWM
S1 S6
PWM
Vac / Iac
Pref +
–
+ *Iq_ref
PI
Controller
PI
Controller
*Delta
*Delta
–
Vref
/ Iref
Ppv
0.0
*I
d_ref
Fig. 6 Voltage source inverter and its control method
From Fig. 6, the process of inverter control system is rather complex which used the former class system voltage fluctuations and waveform distortion signal to control the
Vpv
Comparator
Comparator
Vpv_min
PI
Controller
PI
Controller
V
next class system. To ensure power supply, the switch or reenter of inverter output will ake frequency management
pv_max
+
–
Vpv
control complex and difficult. It will increase the complexity of the control system of the main circuit if setting another AC switch, meanwhile the system will have a big power fluctuation.
-
-
BATTERY STORAGE SYSTEMS
The battery is connected to the grid through its own inverter as shown in Fig. 7. The battery is controlled in such a way that it discharges only when the PV panel is unable to supply the required power. At other times, it charges from the grid if its state of charge is lower than the maximum [21]. The battery side inverter is also controlled by a grid voltage-oriented control similar to that of the PV. The active current reference (Iq) is derived as the sum of the charging current component (Iq,charge) and discharging current component (Iq,discharge) as given in equation 6.
Fig. 7 Control diagram for the battery charging and discharging
-
SIMULATION RESULTS
Detailed simulation studies are carried out on MATLAB/Simulink platform, and the results obtained for various operating conditions are presented in this section. The values of parameters used in the model for simulation are listed in Table I. The steady-state response of the system during the MPPT mode of operation is shown in Fig. 8 during slow change in input to system.
= [
(
+
)] (
+ 1 )
,
(4)
When Vb > Vb, minimum;
= [
(
+
)] (
+ 1 )
,
,
(5)
Fig. 8 Varying solar radiations input to the PV system
When Vb < Vb, minimum;
= +
The system response for ramp changes in the source insolation level while operating in the MPPT mode in conjunction with DC/DC boost converter is shown in Fig. 9.
,
(6)
,
,
Here converter with conjunction two PV systems is able to maintain a constant voltage (i.e.,300V dc) across the inverter
As shown in Fig. 7, the PI controller for battery charging is used to ensure that the sum of the real power produced by the battery and PV is maintained at a specified reference value, such that the battery only supplies the amount of real power lost during shading of the PV panels[22]-[23]. Active power is supplied by the battery as long as the terminal voltage of the battery is above the
for its operation and supply it to grid. The waveform in figure states that the converter can handle the changes very efficiently when dynamics occurs during time interval 0.5 sec to 2sec.
Fig. 9 DC Boost converter output for steady Inverter operation
When the system is in steady state, solar irradiance is 1000 W/m2, and temperature is 298K. From figure 10, it is known that at this condition, the maximum output power of PV system is 2000W but due to switching and operational losses the system produces an output of 1800 W before 0.5 sec. As the insolation level goes down below the STC condition, there is slight fall in in output of the systems and generating output power of 1280W following the MPP region. As the insolation start gaining its level, the PV system with help of MPPT can track the radiations and regains its original MPP point at time 2 sec. This all observation can be seen in below figure which makes the system to rely on different changing environmental conditions without change in its voltage level which is shown in figure 9.
Fig. 10 Power generated from Multi-array PV system with efficient MPP tracking
In Fig. 11 it is shown that the converter three-phase output voltages are under uniform full insolation throughout the system. The powers processed by the cascaded cells are equal in this operating condition. The multi-array converter produces a smooth near-sinusoid output voltage which makes it a low distortion system. When the inverter cells are cascaded, the physical locations of those cells could be far from the output voltage vectors of individual inverter modules are in phase and equal in magnitude.
Fig. 11 VSI converter output voltages in a multi-array PV system under varying insolation
The zoomed view of out voltage waveform of VSI converter is shown in Fig. 12, where it can be noticed that it has high level of multilevel stepped structure making it a pure sine wave in nature.
Fig. 11 Zoomed view of output of 3- VSI converter
Fig. 13 Modulation Index for proper operation of 3- VSI converter
The above waveform shown in figure 13 depicts the steady operation of voltage source inverter under various varying insolation conditions for proposed topology criteria.
-
CONCLUSION
The multi-array PV framework has been stimulated in this paper which incorporates a proposed equal association of two-level inverters to produce a staggered type yield. Thus, related to a grid voltage control for the active and reactive control, the waveforms show the quality has been improved and requirement are reduced. A battery energy storage system (BESS) is incorporated with a control algorithm to minimize the negative transient effects due to dynamics occurring in PV systems. The framework is illustrated through stimulation in the MATLAB. The proposed configuration is capable of supplying uninterruptible power to ac loads, and ensures evacuation of surplus photovoltaic power into the grid.
REFERENCES
-
Luis M. Castro, J.R. RodrÃguez-RodrÃguez and Cecilia Martin- del-Campo, in Modelling of PV systems as distributed energy resources for steady-state power flow studies,International Journal of Electrical Power & Energy Systems, Volume 115, 2020, 105505, ISSN 0142-0615.
-
V. Rallabandi, O. M. Akeyo and D. M. Ionel, "Modeling of a multi-megawatt grid connected PV system with integrated batteries," 2016 IEEE International Conference on Renewable Energy Research and Applications (ICRERA), 2016, pp. 1146- 115.
-
J. Kumar, A. Agarwal and N. Singh, "PV fed Hybrid Energy Storage System Supported DC Microgrid," 2019 IEEE 1st International Conference on Energy, Systems and Information Processing (ICESIP), 2019, pp. 1-6, doi: 10.1109/ICESIP46348.2019.8938295.
-
F. Blaabjerg and D. M. Ionel, Renewable energy devices and systems state-of-the-art technology, research and development, challenges and future trends, Electric Power Components and Systems, vol. 43, no. 12, pp. 13191328, 2015.
-
U. Shahzad, The need for renewable energy sources, International journal of information technology & electrical engineering, pp. 16- 18, August 2015.
-
E. Koutroulis and F. Blaabjerg, Overview of maximum power point tracking techniques for photovoltaic energy production systems, Electric Power Components and Systems, vol. 43, no. 12, pp. 13291351, 2015.
-
V. Vekhande and B. G. Fernandes, Module integrated dc-dc converter for integration of photovoltaic source with dc microgrid, in IECON 2012 – 38th Annual Conference on IEEE
-
Z. Wang ad H. Li, "Integrated MPPT and bidirectional battery charger for PV application using one multiphase interleaved three-port dc-dc converter," 2011 Twenty-Sixth Annual IEEE Applied Power Electronics Conference and Exposition (APEC), 2011, pp. 295-300, Industrial Electronics Society, Oct 2012, pp. 56575662.
-
S. Essakiappan, H. S. Krishnamoorthy, P. Enjeti, R. S. Balog and S. Ahmed, "Multilevel Medium-Frequency Link Inverter for Utility Scale Photovoltaic Integration," in IEEE Transactions on Power Electronics, vol. 30, no. 7, pp. 3674-3684, July 2015, doi: 10.1109/TPEL.2014.2350978.
-
Yongheng Yang and Frede Blaabjerg, Overview of Single- Phase Grid-Connected Photovoltaic Systems, Electric Power Components and Systems, Volume 43, 2015 – Issue 12: Renewable Energy Devices and Systems – State-of-the-Art and Future Trends.
-
G. R. Walker and J. C. Pierce, "Photovoltaic DC-DC module integrated converter for novel cascaded and bypass grid connection topologies Design and optimization," 2006 37th
IEEE Power Electronics Specialists Conference, 2006, pp. 1-7, doi: 10.1109/pesc.2006.1712242.
-
Fei Ding, Peng Li, Bibin Huang, Fei Gao, Chengdi Ding and Chengshan Wang, "Modeling and simulation of grid-connected hybrid photovoltaic/battery distributed generation system", CICED Proceedings, 2010, pp.1-10.
-
Yanqing Li, Cheng Chen and Qing Xie, "Research of an improved grid-connected PV generation inverter control system," 2010 International Conference on Power System Technology, 2010, pp. 1-6.
-
Narasimha Prasad Tulasi and Lakshmi Devi Aithepalli, Droop Control of Bi-Directional DC-DC Converter for Improved Voltage Regulation and Load Sharing in DC Microgrid, in International Journal of Intelligent Engineering and Systems, Vol.12, No.3, 2019, pp. 228 – 243. DOI: 10.22266/ijies2019.0630.23
-
Yogesh Tiwar and Gokul Kumar Dewangan, A Review on Grid Connected Multi Array PV Battery Based Bi-Directional DC DC Converter, International Research Journal of Engineering and Technology (IRJET), Volume: 06 Issue: 06 | June 2019.
-
Nicola Femia: et. Al, Optimization of Perturb and Observe Maximum Power Point Tracking Method, IEEE Transactions On Power Electronics, VOL. 20, NO. 4, JULY 2005.
-
K. Irisawa, T. Saito, I. Takano, and Y. Sawada, Maximum power point tracking control of photovoltaic generation system under non-uniform insolation by means of monitoring cells, in Proc. 28th IEEE Photovoltaic Specialists Conf., Sep. 2000, pp. 17071710.
-
C. Hua, J. Lin, and C. Shen, Implementation of a DSP- controlled photovoltaic system with peak power tracking, IEEE Trans. Ind. Electron.,vol. 45, no. 1, pp. 99107, Feb. 1998.
-
Jacob James Nedumgatt, Kothari D P; et.al, Perturb and Observe MPPT Algorithm for Solar PV Systems-Modeling and Simulation, Published in Annual IEEE India Conference 2011,DOI:10.1109/indcon.2011.6139513.
-
S. Carreon-Bautista, A. Eladawy, A. Nader Mohieldin and E. Sánchez-Sinencio, "Boost Converter With Dynamic Input Impedance Matching for Energy Harvesting With Multi-Array Thermoelectric Generators," in IEEE Transactions on Industrial Electronics, vol. 61, no. 10, pp. 5345-5353, Oct. 2014, doi: 10.1109/TIE.2014.2300035.
-
S. A. Zabalawi, G. Mandic, and A. Nasiri, Utilizing energy storage with PV for residential and commercial use, 34th Annual Conference of IEEE, pp.10-13, 2008.
-
Y. Zhang, ;. Jiang, and ;. X. Yu, Control Strategies for Battery/Supercapacitor Hybrid Energy Storage Systems, Energy 2030 Conference, pp.1-6, 2008.
-
F. S. Garcia, A. A. Ferreira, and J. A. Pomilio, Control Strategy for Battery-Ultracapacitor Hybrid Energy Storage System, pp.826-832, 2009.