
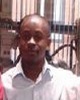
- Open Access
- Authors : Ubong Ukommi , Emmanuel A. Ubom , Idaresit I. Abraham
- Paper ID : IJERTV9IS080104
- Volume & Issue : Volume 09, Issue 08 (August 2020)
- Published (First Online): 15-08-2020
- ISSN (Online) : 2278-0181
- Publisher Name : IJERT
- License:
This work is licensed under a Creative Commons Attribution 4.0 International License
Outdoor 2.4GHz Band Wi-Fi Networks Performance Characterisation
Ubong Ukommi, Emmanuel A. Ubom, Idaresit I. Abraham
Department of Electrical and Electronic Engineering, Akwa Ibom State University, Nigeria.
Abstract:- The task of proper network planning with little or no attenuation has been the major target of communication engineers. To achieve this target, network planners, communications infrastructure vendors and the academia, all over the world have been studying the propagation characteristics of signals to enable them establish networks that suit specific terrain characteristics (such as differences in weather conditions, building structures, trees and other vegetation, which may cause attenuation and/or obstruct the propagated signals from getting to its destination) thereby offering better coverage and quality of service (QoS). To understand these parameters within the Akwa Ibom State University (AKSU) environment, empirical measurements were taken and from the analysis, the path loss exponent for outdoor environments in the 2.4GHz band found to be 1.999. In comparison of the graphical plots of different models, the measured model and COST 231 Walfisch-Ikegami model were adopted for network planning in the terrain.
1 INTRODUCTION
Wireless signal characteristic changes as it travels from the transmitter to the receiver. These changes depend predominantly upon the distance between the two antennas, the path(s) taken by the signal, and the environment (vegetation, Rivers, and buildings) that makes up the path. It is therefore possible to profile the received signal from the profile of the transmitted signal taking into consideration the properties of the medium between the two. These properties of the medium are bundled into what is generally known as channel model. Channel models describe how the channel functions such as Path loss, Shadowing and Multipath affect the properties of received signal that has travelled through the path. This is to say that the performance of any wireless link is a direct function of the terrain it is deployed, as such careful network planning has become increasingly critical with the rising deployment, coverage and congestion issues associated with todays Wireless Local Area Networks (WLAN). The determination of the path properties as well as the proper application of such path properties in the design of wireless networks is therefore very essential. This way a network planner can determine the placement and infrastructure required to meet the demands of the network for a deployment of any size, guaranteeing good Quality of Service (QoS). The issue of networking arose when there was need to transmit data from one system to another most especially in multiple system environments. Networking was introduced either as Local Area Network (LAN), Wide Area Network (WAN) or made to cover the Metropolitan (MAN) [1]. Area Networks (AN) could be wired, wireless or hybrid of both access technologies. Wired ANs involve the use of cables to link one
communicating device to another. Cable enabled LAN is almost impossible in some buildings and expensive due to the high cost of cable requirement. Wireless communication, on the other hand, comes with huge flexibility and mobility, eliminating cable length limitation, difficulties experienced in laying and maintaining structural cabling methods as issues like rodents, cable cuts and damages are very common and most African infrastructures were not designed with network cabling in mind. Other benefits include the dynamic network formation, easy deployment, and low cost in some cases, making wireless technologies an appealing networking technique for broadband services in Africa [2] the demand of which has maintained consistent growth.
The most popular technology used for Wireless Local Area Networks is the IEEE 802.11b/g [3]. 802.11 compliant networks are based on Direct Sequence Spread Spectrum (DSSS) transmission technique or Orthogonal Frequency Division Multiplexing (OFDM) [4]. Using 13 frequency channels with 22MHz bandwidth and 5MHz spacing, 802.11 technology occupies a total bandwidth of 82MHz from 2410MHz to 2483MHz. Taking advantage of CCK-OFDM modulation, 802.11 WLANs are capable to achieve a bit-rate of 54Mbps (802.11g) [5]. With the proliferation of Wireless Local Area Networks (WLANs) and impending congestion that follows, careful network planning and propagation modelling have become essential to help planners determine accurate path loss profiling and coverage plan for the networks. The drive therefore is to study the path-loss characteristics of Wi-Fi signals in rural/ sub-urban Africa Terrain.
-
Review of Outdoor Signal Propagation
In reality, the amount of power transmitted from the transmitter usually reduces as it passes through a medium, hence, the signal strength is said to be degrading with distance. Path loss is the difference in (dB) between the transmitted power and the received power, and represents signal level attenuation caused by free space propagation, reflection, diffraction and scattering as a result of obstacles on the path of the signal. Figure 1.1 illustrates path loss in a typical wireless communication system [6]. Proper network coverage planning requires a good understanding of the fundamental limitations caused by diverse radio signal propagation mechanisms due to variable environmental conditions. According to [7], these phenomena induces signal scattering, fading and shadowing along the signal path and their effects can best be described (in a large scale) by the path loss exponent which defines the rate of change of
attenuation that the signals suffers as it propagates from the transmitter to the receiver. The average path loss for a transmitter to receiver separation is expressed as a function of distance [8].
Figure 2.1: A Diagram illustrating the Path Loss
-
Review of some related works
In the work done by [9], analytical approach was
S/N Hardware Name Brand Model
1. Laptop
Hewlett Packard (HP)
HP1000
1. Laptop
Hewlett Packard (HP)
HP1000
-
Wi-Fi radio TP-LINK TL-WR940N
-
GPS MAP76CSX
-
Recording
materials
employed using the Okumuras model to determine the propagation range of IEEE 802.11 Radio LANs for outdoor applications. Similarly, to evaluate the performance of 802.11b, [10] used two laptops equipped with Orinoco WLAN cards, and two PCs with D-Link WLAN cards. [11- 12] also used empirical methods to show the coverage area and the signal strength inside the coverage area to reveal the technology that gives better WLAN [13] using computer simulation of a typical 802.11b/e access to an IP core network through an access point in an infrastructure WLAN. [14-16] carried out measurements of transmission on microwaves bands were taken at twelve different locations in the University of Essex campus using laptop, USB adapter, access points and GPS. At each location, 25 signal strengths sampling from the Access Points (APs) were recorded. Simulations of the measured values were carried out using the Excel, XML program, Haversine formula, and Friis propagation law. It was noted that each of the access points degraded in throughput by certain percentage due to interference from other neighbouring access points. Our interest here is on the path loss exponent on 2.4 GHz Wi-Fi.
-
RESEARCH METHODOLOGY
The research work was carried out in Akwa Ibom State University (AKSU) Main Campus located at Ikot Akpaden, Mkpat Enin Local Government Area in Akwa Ibom State. Akwa Ibom is one of th states in South-South region of
-
Test-bed Setup
The test bed consisted of a Wi-Fi radio, GPS receiver, a laptop, pen and recording book. The Received Signal Strength (RSS) on Wi-Fi interface were detected by the InSSIDer software running in the laptop and the measured signal strength recorded as shown below in random position of equal distance increased at interval of 10m from the transmitter location.
The 2.4GHz access point was set up at a fixed position in a 5m height in the university environment and through the use of GPS the exact distance of the mobile device was measured and recorded at intervals of 10m until 100m. At each distance,
20 signal strength measurements were carried out and the same measurement was repeated for different distances ranging from 10m to 100m, the mobile device maintained at an approximate height of 1.5meters. Using the net monitor application of InSSIDer software operated in the active mode and MAP76CSX GPS to determine the distance from Access point, the received signal strength was measured at 2412MHz and the average signal strengths were calculated.
-
Outdoor Empirical Propagation Models
To ensure that proper network planning is carried out in a particular terrain, it is necessary to employ a suitable propagation model that best fit such terrain. Rampantly, installers adopt ad-hoc mode of outdoor Wi-Fi coverage where they place radio infrastructure in small test areas and through measurement surveys verify coverage and then repetitively more and more areas are added until full coverage is achieved. This is in no way a professional method of network design. It is necessary to adopt an adaptive deployment design methodology, known as infrastructure mode. In this mode, a predictive deployment and network calculations are done using an accurate path loss model. There are several models which can be used for predictions in the outdoor environment. Such models are grouped into three major types Empirical models, Semi-deterministic models and Deterministic models. We will briefly discuss some empirical models here.
2.2.1 Free Space Path Loss Model
The free space path loss considers the reduction in signal strength even when propagated in a vacuum without obstacles. This sounds ideal and the loss are accrued to spreading of the signal, the effective aperture of the receiver antenna and the transmit power. The path loss is provided for isotropic antennas with provisions for others.
For an ideal isotropic antenna, the free space path loss is given by
4 2 4 2
Nigeria and shares boundaries with the Atlantic Ocean, Cross
River State, Abia State and River State. Akwa Ibom State
= [
] = [
] . . (2.1)
University (AKSU) terrain is swampy in nature and consists
The power received is given by
of several vegetation and foliage, which may cause
= [
2
] = [
2
]
. . . (2.2)
obstructions to signal propagation. For the purpose of this
4
4
project, the AKSU main campus was classified as a suburban community which consisted of students and staff, buildings, offices and lecture halls as well as sparsely located storey buildings and trees although located in the rural suburb of the state.
The free space path loss can be calculated in dB thus,
= 1010 ( )
= {2010() + 2010() + 21.98 . . (2.3) 2010() + 2010() 147.56
where,
= wavelength measured in metres(m)
d = Transmitter Receiver Separation (m)
Pt = Transmitted Power f = Carrier frequency
c = Speed of light (3.0 x 108m/s)
For other antennas, considerations must be given to the gain of the antenna, which yields the following free space path loss equation:
2.2.3 Cost 231-Hata Path Loss Model
In order to accommodate for higher frequency and lower antenna heights, Okumura-Hata model for medium to small cities has been extended to cover 1500MHz to 2000MHz in 1999. The purpose was to extend the limitations of the HATA models and apply appropriate correction factors to improve upon their degree of correctness in Europe [18].
= + 10 + . (2.9)
(4)2 ()2
()2
= =
2
= . . (2.4)
2
Where, = 46.3 + 33.910 13.8210 =
0 , For Medium sized Cities and Suburban areas
where,
Gt = Gain of the transmitting antenna Gr = Gain of the receiving antenna
At = Effective area of the transmitting antenna Ar = Effective area of the receiving antenna
Equation (2.4) shows that the gain of an antenna is given by:
4
G = ,
2
where Aeis the effective area…(2.5)
2.2.2 Okumura-Hata Path Loss Model
Okumura path loss model is an empirical based model for irregular terrain. The Okumura-Hata model is the most popular model based on extensive measurements made in and around Tokyo in 1968, where Okumuras observations was formulated into a simple mathematical model of the form ( + ), where A and B are functions of frequency, antenna heights and path loss exponent and D is the distance. Hata model employed the analytical approximation to Okumura model and median path loss empirical model is widely used. The model is suitable for frequency range of
{ 3 , For Metropolitan areas
2.2.4 Walfisch-Ikegami Path Loss Model
Cost 231-WI is a semi-empirical path loss model that takes the characteristics of the city structure under study into account. Such characteristics include: Heights of buildings (hroof), Widths of roads (w), Building separation (b) and Road orientation with respect to the direct radio path (). The model increases accuracy of the propagation estimation but it is more complex when compared with Okumura-Hata model. The model allows estimation from 20m instead of 1km for Okumura-Hata model and mean path loss is suitable for the model. Cost 231 is restricted to the frequency (f) between 800MHz and 2000MHz, transmitter height () between 4
and 50m, receiver height () between 1 and 3m and a transmitter-receiver distance (d) between 0.02 and 5km.
Figure 2.6 illustrates the above stated parameters to be taken into considerations when conducting field measurement that is to be analyzed using Cost 231-WI model [19]. The equations are:
Case 1 – LOS:
150-1500 MHz and a distance between 1km and 20km. Hata
divided the prediction area into three set of terrain categories,
()
= 42.6 + 2610
[]namely open, suburban and urban area. The Hata model specifies the Base Station antenna height to be from 30m and
Case 2 – NLOS:
+ 2010[] (2.10)
Mobile Station height from 3m and room for correction factors addition [17]. Okumura-Hata Path Loss Model Equations for different terrains are:
Urban Terrain:
= + 10 . (2.6)
Suburban Terrain:
= + 10 . . . (2.7)
Open Terrain:
() = + (, , , )
+ (, , , , ) (2.11)
It should be noted that,
= Free space path loss
= 32.4 + 2010[]
+ 2010[]
Where,
= + 10
. . . . (2.8)
= Roof to street loss, given by
It should be noted that,
= 69.55 + 26.1610() 13.8210() = 44.9
= 8.8 + 1010[] + 2010( [])
1010([]) +
2
6.5510() = 2 [10 ( )]
+ 5.4 =
= Street orientation function
28
4.78[10()]2 + 18.3310() + 40.94 =
10 + 0.35, 0 < 35°
3.2[10(11.7554)]2 4.97, For Large Cities, f 300MHz = { 2.5 0.075( 35°), 35° < 55°
{ 8.29[10(1.54)]2 1.1, For Large Cities, f < 300
4.0 0.114( 55°), 55° < 90°
(1.110 0.7) (1.5610 0.8), For Medium and Small Cities
Table
Distance (m)
3.1: Measured Received Mean received power (dB)
Power and Path Loss
Mean path loss (dB)
10
-78.75
60.09
20
-79.50
66.10
30
-83.50
69.63
40
-86.00
72.13
50
-87.00
74.06
60
-87.00
75.65
70
-90.00
76.99
80
-89.50
78.13
90
-92.00
79.17
100
-93.00
80.08
Table
Distance (m)
3.1: Measured Received Mean received power (dB)
Power and Path Loss
Mean path loss (dB)
10
-78.75
60.09
20
-79.50
66.10
30
-83.50
69.63
40
-86.00
72.13
50
-87.00
74.06
60
-87.00
75.65
70
-90.00
76.99
80
-89.50
78.13
90
-92.00
79.17
100
-93.00
80.08
= Multi diffraction loss and is given by the following equation,
= bsh + + 10[] + 10[]
910
It should be noted that,
1810(1 + ), >
bsh = {
0,
0,
From Table 3.1, the received signal strength (RSS) was
54, >
54 0.8 , 0.5,
54 0.8 , 0.5,
=
plotted against distance to investigate the performance of the signals. The result is as shown in Figure 3.1 below.
{ 54 0.8
[]0.5
, < 0.5,
-78
-80
Received Signal Strength [dB]
Received Signal Strength [dB]
-82
Graph of RSS against distance in outdoor environment
18, >
= { 15
-84
18
,
-86
-88
= 4 + {
0.7 (
925
1) ,
-90
-92
-94
10
20 30 40 50 60 70 80 90 100
1.5 (
925
1) ,
Distance [m]
Figure 3.1: Graph of RSS against distance.
-
-
RESULTS PRESENTATION
-
Table 3.1 shows the mean received signal strength and the path loss calculated from ten repeated samples for the corresponding distance at random directions from the transmitter in the outdoor environment. The path loss was calculated according to equation:
-
Determination of Path Loss Exponent using Linear Regression
From equation (3.4) the path loss exponent was calculated for outdoor environment within Akwa Ibom State University as:
131.13
From [7]:
=
65.5976
= 1.999
() = [ ( ) ( )]2 . (3.1)
-
Determination of a Shadow Factor using Standard
=1
Deviation Analysis
To compensate for shadow fading as the terrain is composed
Where: () is the measured path loss and () is the
estimated path loss where,
of buildings and other structures the large scale average path loss was predicted at 50m as: () = 60.09 +
(
(
50
10
10
19.9910 )
0
0
() = (0) + 1010 ( ) (3.2)
Substituting equation 3.2 into 3.1, we have
= 74.06 . . (3.5)
Using the predicted path loss value, the squares of mean deviations were generated as shown in Table 3.2.
Table 3.2: Relationship between measured and
Distance (m) |
() |
( ) () |
( ) () |
10 |
60.09 |
-13.97 |
185.1609 |
20 |
66.10 |
-7.96 |
53.3616 |
30 |
69.63 |
-4.43 |
19.6249 |
40 |
72.13 |
-1.93 |
3.7249 |
50 |
74.06 |
0.00 |
0.0000 |
60 |
75.65 |
1.59 |
2.5281 |
70 |
76.99 |
2.93 |
8.5849 |
80 |
78.13 |
4.07 |
16.5649 |
90 |
79.17 |
5.11 |
26.1121 |
100 |
80.08 |
6.02 |
36.2404 |
. |
( )2 =351.2404 |
Distance (m) |
() |
( ) () |
( ) () |
10 |
60.09 |
-13.97 /td> |
185.1609 |
20 |
66.10 |
-7.96 |
53.3616 |
30 |
69.63 |
-4.43 |
19.6249 |
40 |
72.13 |
-1.93 |
3.7249 |
50 |
74.06 |
0.00 |
0.0000 |
60 |
75.65 |
1.59 |
2.5281 |
70 |
76.99 |
2.93 |
8.5849 |
80 |
78.13 |
4.07 |
16.5649 |
90 |
79.17 |
5.11 |
26.1121 |
100 |
80.08 |
6.02 |
36.2404 |
. |
( )2 =351.2404 |
predicted path loss
() = [
( )
( ) 10
2
( )]
. . . (3.3)
=1
10 0
The maximum value of () would occur if () = 0,
hence differentiating () and equating the result to zero, the path loss exponent was determined as:
1[() ()]
=
=
=1
1010
( )
0
. . (3.4)
Hence, standard deviation was obtained thus:
n (P P )2
= k=1 m r (3.6) N
Where N is the number of measured data points.
351.2404
= = 5.93dB 10
4.0 CONCLUSION
From Figure 3.2, the measured result was compared with existing propagation models. As depicted in the figure, it can be observed that the free space model is much lower than the measured result; Okumura-Hata and Cost 231-WI are much closer to the measured model while Cost 231-Hata model is higher than the measured model. Therefore, it would be better to consider Cost 231-WI model for proper network planning in the terrain as the error between the model and the measured result is lower compared to other models. The indoor Path- loss was characterized to P (dB) = 60.09 + 19.99log ( d ) +
From standard normal curve, the shadow factor, X must not be greater than 11.86dB.
L d0
9.78 with an exponent of 1.999.
Using cumulative density function and considering that the mean value () is 0dB and (z) = 1.65, then
REFERENCES:
-
William Stallings. Wireless Communications and Networks.
0
= 1.65;
= 9.78
Prentice Hall, 2002.
5.93
With this value, the path loss beyond reference distance is
given by,
d
-
Jonathan PINIFOLO, Suvendi RIMER, Babu PAUL, Chomora MIKEKA, Justice SP MLATHO. TV White Spaces Technical rules for Africa to enable efficient Spectrum Management ISSN 2223-7062 Proceedings and report of the 7th UbuntuNet Alliance annual conference, 2014, pp 355-364
-
IEEE standard for wireless LAN Medium Access Control (MAC)
PL(dB) = 60.09 + 19.99log (
d0
) + 9.78 . . (3.7)
and Physical Layer (PHY) specifications, ISO/IEC 8802- 11:1999(E), Aug. 1999.
3.4 Application of Existing Models and Comparison with Measured Result
Using the Free Space Path Loss, Okumura-Hata Cost 231- Hata and Cost 231-WI model equations given in 2.2 and substituting hb = 5m, hm = 1.5m and f = 2412MHz, the model equations shown in Table 3.3 were obtained. Using the model equations and MATLAB, the required graphical plots shown in Figure 3.2 was plotted.
Table 3.3: Propagation Models and Model Equations
Model Model Equation
Measured
() = 60.09 + 19.9910 ( ) + 9.78
Measured
() = 60.09 + 19.9910 ( ) + 9.78
0
0
Free Space = 2010() 79.91
Okumura-Hata = 40.3210 + 135.48
-
Jin-Shyan Lee and Yuan-Ming Wang Experimental Evaluation of ZigBee-Based Wireless Networks in Indoor Environments Received 13 September 2012; Revised 21 January 2013; Accepted 21 January 2013 Research Article Copyright © 2013.
-
Andreas I. Miaoudakis, Dimitrios I. Stratakis, Emmanuel Antonidakis, Vassilios Zaharopoulos, and Radovan Stojanovi, Co-existence Performance Evaluation of Wireless Computer Networks in a Typical Office Environment.
-
Sylvain Ranvier / Radio Laboratory / TKK Path loss models S-
72.333 Physical layer methods in wireless communication systems sylvain.ranvier@hut.fi, 23 November 2004.
-
Ubom, E.A., Idigo, V. E., Azubogu, A.C.O., Ohaneme, C.O. and Alumona, T. L. Path loss Characterization of Wireless Propagation for South South Region of Nigeria, Vol. 3, June, 2011.
-
Seybold, J. S. Introduction to RF propagation John Wiley & Sons, Inc., Hoboken, New Jersey, 2005.
-
G. Ofori-Dwumfuo and S. Salakpi, WiFi and WiMAX Deployment at the Ghana Ministry of Food and Agriculture, Research Journal of Applied Sciences, vol. 3, 2011.
Cost 231-WI = 2610[] + 110.25
Cost 231-WI = 2610[] + 110.25
Cost 231-Hata
= 40.3210
+ 151.23
-
J. J. van Rensburg and B. Irwin, "Wireless Network Visualization Using Radio Propagation Modelling," 2005.
-
Y. Lee, K. Kim, and Y. Choi, "Optimization of AP placement and channel assignment in wireless LANs," 2002, pp. 831-836.
-
A. Mishra, E. Rozner, S. Banerjee, and W. Arbaugh, "Exploiting
150
100
Path Loss [dB]
Path Loss [dB]
50
0
-50
-100
-150
Graph to compare Measured result with Existing models
Measured Model Free Space Model Okumura-Hata Model Cost 231-Hata Model Cost 231-WI Model
partially overlapping channels in wireless networks: Turning a peril into an advantage," 2005, pp. 29.
-
Femi-Jemilohun Oladunni .Juliet, Walker Stuart, Empirical Performance Evaluation Of Enhanced Throughput Schemes of IEEE802.11 Technology In Wireless Area Networks School Of Computer Science and Electronic Engineering University of Essex Colchester, Essex, United Kingdom, pg. 8
-
Wu, J. and Yuan, D., Propagation Measurements and Modeling in Jinan City, IEEE International Symposium on Personal, Indoor and Mobile Radio Communications, Boston, MA, USA, Vol. 3, 1998, pp. 1157-1159.
-
A. Medeisis, A.Kajackas, On the Use of the Universal Okumura- Hata Propagation Prediction Model in Rural Areas, IEEE Vehicular Technology Conference Proceeding, Vol. 3, May 2000, pp. 450-453.
-
COST Action 231, Digital mobile radio towards future generation systems, final report, tech. rep. European Communities, EUR 18957, 1999.
0.01 0.02 0.03 0.04 0.05 0.06 0.07 0.08 0.09 0.1 0.11
Distance [km]
Figure 3.2: Graph to compare the measured result with the existing models
-
R. Vaughan, J. Bach Andersen, Channels, Propagation and Antennas for Mobile Communications, IEE, 2003, 73 p.
-
A. Grilo and M. Nunes, Performance evaluation of IEEE 802.11e in Personal, Indoor and Mobile Radio Communications, 2002, The 13th IEEE International Symposium on, 2002, pp. 511-517.
-
M. J. Ho, M. S. Rawles, M. Vrijkorte, and L. Fei, "RF challenges for 2.4 and 5 GHz WLAN deployment and design," 2002, pp. 783- 788 vol.2.