
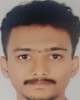
- Open Access
- Authors : Kara Naga Sree Vallabh, Asileti Prashanth, Cheera Vamsikrishna, Kallelapola Sankar, Minjuru Kuma, N. Rama Rao
- Paper ID : IJERTV13IS030078
- Volume & Issue : Volume 13, Issue 03 (March 2024)
- Published (First Online): 22-03-2024
- ISSN (Online) : 2278-0181
- Publisher Name : IJERT
- License:
This work is licensed under a Creative Commons Attribution 4.0 International License
Parametric Analysis and Design of Cantilever Retaining Walls Under Different Soil Conditions
Kara Naga Sree Vallabh, Asileti Prashanth, Cheera Vamsikrishna, Kallelapola Sankar, Minjuru Kuma, N. Rama Rao
UG Students Final Year, Associate Professor, Department of Civil Engineering, NRI Institute of Technology, Agriripalli, India
Abstract The project deals with the parametric analysis of cantilever retaining walls and how it is going to react by varying in bending moment and difference in reinforcement which proportionally varies accordingly while changing the type of soils, all among the types of soils and get our requirements i.e., (bending moment and reinforcement). Here we selected the constant height for the retaining wall as 3m.Here we use different types of soils such as Intact clay, calcareous clay, silt, sand and gravel. KeyWords: Retaining wall, moment, stem, heal, toe, reinforcement.
-
INTRODUCTION
A retaining wall is a structure designed to hold back soil or other materials and prevent erosion or collapse in areas where there is a change in ground elevation. They serve various purposes, including creating usable space on sloped terrain, preventing soil erosion, and providing structural support for roads, buildings, or other infrastructure. Retaining walls come in different types, such as gravity walls, cantilever walls, and reinforced walls, each with its own construction methods and design considerations. Proper engineering, including analysis of soil properties, drainage systems, and loading conditions, is essential for the successful construction and long-term stability of retaining walls. Additionally, factors such as aesthetics, environmental impact, and local regulations play a role in the design and implementation of retaining wall projects. Overall, retaining walls are critical elements of infrastructure and land development, providing both functional and aesthetic benefits in various landscapes.
A retaining wall is designed to hold in place a mass of earth or the like, such as the edge of a terrace or excavation. The structure is constructed to resist the lateral pressure of soil when there is a desired change in ground elevation that exceeds the angle of repose of the soil.
A basement wall is thus one kind of retaining wall; however, the term usually refers to a cantilever retaining wall, which is a freestanding structure without lateral support at its top. These are cantilevered from a footing and rise above the grade on one side to retain a higher level grade on the opposite side. The walls must resist the lateral pressures generated by loose soils or, in some cases, water pressures.
-
LITERATURE REVIEW
Following literature represents the works conducted on analysis and design cantilever retaining wall.
Dembicki et al (1989) Systematic analysis methodologies to evaluate the structural behavior and performance of cantilever retaining walls. Through rigorous calculations and simulations, we investigate factors such as soil properties, loading conditions, and wall geometry to optimize design parameters.
Kerisel (1993) Project deals with history of retaining wall design. In Retaining structures retaining walls are the most useful structure where this retaining wall is founded first nothing but the origin of retaining wall.
Goh (1993) Analyze the behavior of cantilever retaining walls through empirical observation and mathematical modeling. By examining factors such as soil properties, wall geometry, and loading conditions.
Bentler et al (2006). We assess its ability to withstand lateral earth pressure and maintain stability. This involves analyzing factors like deflection, settlement, and potential structural distress. Through monitoring and periodic inspections.
Guler et al (2007) Conduct numerical analyses to evaluate the performance of reinforced soil-retaining wall structures. By considering various backfill materials, including cohesive and granular types, we assess factors such as stability, deformation, and load- bearing capacity to inform design optimization.
Sivakumar et al (2008) It was an optimum design of cantilever retaining walls we chose that to make a upper limit of economical target using target reliability approach.
Fenton, et al (2008) project deals with the Reliability of the traditional retaining wall and the design particulars what we have used the design first time.
Lee et al (2008) Investigate deformation patterns in earth retaining walls through advanced analytical techniques and field observations. By analyzing factors such as soil mechanics, wall material properties, and external loads.
Liu, et al. (2008) Project deals with the complete structural design of cantilever retaining wall with revealing platform which includes the surcharge for the components.
Abood et al. (2011) We design the cantilever retaining wall with constant height as 4m according to the ACI318 standard for the slab design.
Pei (2012 ) In this method they have chosen to design of reinforced cantilever retaining walls using heuristic optimization algorithms.
Kaveh et al (2014) In this method they used to design a cantilever retaining walls using ray optimization method.
Moon and Ku. (2016) the project deals with empirical estimation of soil unit weight and undrained shear strength from shear wave velocity measurements.
Kumar et al. (2017) Using the differential evolution algorithm, we optimize the dimensions and reinforcement of the cantilever retaining wall for stability and cost-effectiveness. This involves iteratively refining parameters such as wall height, base width, and reinforcement distribution to achieve optimal performance under varying soil conditions.
Dhamdhere et al. (2018) a comprehensive analysis of retaining wall designs, focusing on structural integrity, stability, and cost-effectiveness. Our research explores various construction materials, techniques, and environmental factors to optimize performance and mitigate risks. Through innovative methodologies and empirical studies.
Yadav et al. (2018) we conduct analytical and experimental analyses of retaining walls under both static and seismic conditions. By integrating theoretical models with real-world testing, we assess structural performance, deformation patterns, and failure mechanisms.
POUR et al. (2019) Conduct a parametric analysis of back-to-back reinforced earth retaining walls, exploring variations in factors like reinforcement type, soil characteristics, and wall geometry.
Uray et al. (2019) In the design for concrete cantilever retaining walls in various soils, we analyze soil properties to determine bearing capacity and potential lateral pressures. We explore structural configurations, considering factors such as wall height, base width, reinforcement, and drainage systems, to optimize stability.
Mittal et al. (2021) Focus on the analysis and design of retaining walls incorporating reinforced cohesive frictional backfill. Through comprehensive investigations, we evaluate the interaction between the reinforcement, cohesive soil, and frictional backfill.
Varga et al. (2021) Conduct a multi-parametric analysis of gravity retaining walls. By examining multiple design parameters such as wall height, soil characteristics, and loading conditions, we assess the structural performance and stability of the wall under various scenarios.
-
DIFFERENT TYPES OF SOILS USED IN DESIGN OF CANTILEVER RETAINING WALL
Table- 1 Unit weights of different types of soils
SOILS
UNIT WEIGHT
(kN/m3)Intact clay
15.5
Calcareous clay
17
Silts
18
Sand
20
Gravel
21.5
-
ANALYSIS AND DESIGN OF
CANTILEVER RETAINING WALL
Following analysis and design is conducted for silty soil with the unit weight of 18 kN/m3 and same procedure is followed for remaining types of soil.
Assume 1 m as foundation. We need to design retaining wall of height 3 m, so total height is 3+1
= 4 m
4.3 DIMENSIONS OF BASE
-
DESIGN CONSTANTS
a = 1 0
2.2g
= 0.37
M 20 CONCRETE ( =7 N/mm2)
For the base width of retaining wall
Fe 415 STEEL (
=230 N/mm2)
= 0.95
(1)(1+3)
= 1.90 m
m = 13.33
Height of wall = 3 m Kc= 0.289
Jc = 0.904
Rc = 0.914
= 18 kN/m3
= 300
SBC = 100 kN/m2
= 0.5
-
DEPTH OF FOUNDATION
Where ka = = 0.33
+
Base width as per consideration of sliding
0 7
= (1 ) = 2.96
This width is excessive. Normal practice is to provide b between 0.4 to 0.6 H Take maximum value of 0.6 H
b = 0.6 H = 2.4 m
It is not sufficient hence provide shear key Let the thickness of toe be 1 = 0.3 m
12
2
2
Y = 0 (1sin ) = 0.62 m
-
THICKNESS OF STEM
3
3
min
1+sin
=
= 50.65 x 106 N-mm
6
Table -2 Table Showing Moments According To the Different Loadings
S
no
Designation
Force (kN)
Lever arm (m)
Moment about roe (kN-m)
1
W1
1 X 0.2 X 3.7 X 25 =
18.5
1.1
20.35
2
W2
½ X 0.1 X 3.7 X 25 =
4.63
0.9
7
4.49
3
W3
1 X 2.4 X 0.3 X 25 =
18.0
1.2
21.60
4
W4
1 X 1.2 X 3.7 X 18 =
79.97
1.8
143.86
W = 121.05
MR = 190.30
2
2
EARTH PRESSURE =>
P= = 48 kN/m
= = 161 mm
1000×
2
Take depth as 200 mm and overall depth as 260
OVERTURNING
Mo = P X
4 = 64 kN -m
mm
Ast =
= 566 mm2
3
Factor of safety against overturning =
> 2 hence safe SLIDING
= = 1.26 < 1.5
190.3
64
= 2.97
Half of the reinforcement of stem has been bent up into the toe
Check for development length Ld = 45 x dia of bar
= 45 x 12
= 540 mm
HENCE UNS
AFE
Take cover 50 mm = 900-50 = 850 > Ld
We should provide shear key
-
PRESSURE DISTRIBUTION
Net moment = = 190.3 64 = 126.3 kN-m
Hence safe DISTRIBUTION STEEL
] = 276 mm2
] = 276 mm2
0.12 X1000 [260+200
= = 1.04 m
100
2
1000×50.3
ECCENTRICITY e = = 0.16 m
2
Should not be less than = 0.4 m
Use 8 dia bars spacing =
c/c
4.7 DESIGN OF HEEL SLAB
276
= 182 ~ 180 mm
6
)
)
Pressure p 1 at toe = (1 + 6 = 70.61 kN/ m2 <
100(SBC), hence safe
)
)
Pressure p2 at heel = (1 6 = 30.26 kN/ m2
Weight of soil = 1.2 x 3.7 x 1 x 18 = 80 kN
Weight of heel slab = 1.2 x 0.26 x 1 x 25 = 7.8 kN Total upward soil reaction = ½ X (50.44+ 30.26) 1.2 = 48.42 kN
=
50.44+2(30.26)
1.2 = 0.55 m
Pressure p at junction of stem with toe slab
[ ]50.44+30.26 3
= 1
12 b = 55.48 kN/ m2
B.M (80 x 0.6) + (7.8 x 0.6) (48.42 x 0.55) =
26.05 kN-m
Pressure p at the junction of stem with heal slab
p = p1
p1p2 X width of heel slab = 50.44 kN/
b
=
1000×
= 169 mm
m2
4.6 DESIGN OF TOE SLAB
Downward weight of slab = 7.5 kN/ m2
Hence net pressure = 70.61-7.5 = 63.11 kN/m2 Whereas at junction 55.48 – 7.5 = 47.98 kN/ m2
Total S.F = ½ X (63.11+ 47.98) 0.9 = 50 kN
Hence keep the depth of stem 260 mm and effective depth as 200 mm
4.8 REINFORCEMENT IN STEM
3
3
Earlier we have assumed thickness of toe slab as 0.3m so reduce the height as 4 0.3 = 3.7 m
M = = 52.31 kN-m
=
47.98+2(63.11)
0.9 = 0.47 m
6
[ ]47.98+63.11 3
B.M = 50 X 0. k m
=
= 239 mm
47 = 23.52 N-
1000×
Depth of toe slab as per its moment
Keep d and 250 mm and D as 310 mm
Ast=
= 113 mm2
= 1007 mm2, use 12 dia bars ,one bar area
Sliding force 3(4+a2)
Weight of soil between base = 2.4a x 18 = 42.3 a Total force = 121.05 + 42.3 a
Spacing = ast / Ast = 112 mm
Provide 12 dia bars @ 90 mm c/c
AST provided = 1000 x 113/90 = 1256 mm2
-
DESIGN OF SHEAR KEY
Pp = kp p= 3 x 55.48 = 166.4 kN/m2
Total passive pressure = 166.4a (here a is height of shear key)
1.5 = + =>2 33 8 + 2 54 = 0
We get a as 0.09 m 90 so provide minimum
as 300 mm shear key
-
-
RESULTS AND DISCUSSIONS
The moments in the different types of retaining walls in which in different soil properties changes there will be change in heel stem and to slabs accordingly whereas the reinforcement in the above slabs will be changing accordingly.
52.31
52.31
49.4
49.4
Table 3 Moments for Different types of Soil
Moments in components of retaining wall under different soils (kN-m) |
|||||
Type of slab |
Intact clay |
Calcarreous clay |
Silts |
Sand |
Gravel |
Stem |
45.1 |
49.4 |
52.31 |
58.1 |
62.5 |
Toe |
15.18 |
22.49 |
23.52 |
32.19 |
38.22 |
heel |
31.61 |
37.52 |
26.05 |
21.23 |
19.5 |
70
60
50
40
30
20
10
0
58.1
62.5
70
60
50
40
30
20
10
0
58.1
62.5
INTACT CLAY
CALCAREOUS CLAY
STEM
SILTS
SAND
GRAVEL
INTACT CLAY
CALCAREOUS CLAY
STEM
SILTS
SAND
GRAVEL
45.1
45.1
37.52
37.52
38.22
38.22
31.61
31.61
22.49
22.49
23.5226.05
23.5226.05
32.19
21.23
32.19
21.23
15.18
15.18
19.5
19.5
TOE SLAB
TOE SLAB
HEEL SLAB
HEEL SLAB
Figure -1 Moments Graph in Different Soils
942
942
941
941
957
957
941
941
Table-4 Reinforcement Variation in Different Types of Soils
Reinforcement in components of retaining wall under different soils (kN-m)> |
|||||
Type of slab |
Intact clay |
Calcarreous clay |
Silts |
Sand |
Gravel |
Stem |
942 |
957 |
1256 |
1340 |
1675 |
Toe |
470 |
628 |
628 |
941 |
840 |
heel |
941 |
754 |
754 |
628 |
470 |
1800
1600
1675
1800
1600
1675
1400
1200
1000
800
600
400
200
0
1256
1340
1400
1200
1000
800
600
400
200
0
1256
1340
INTACT CLAY CALCAREOUS
CLAY
SILTS
SAND
GRAVEL
INTACT CLAY CALCAREOUS
CLAY
SILTS
SAND
GRAVEL
STEM
TOE SLAB
HEEL SLAB
STEM
TOE SLAB
HEEL SLAB
754
754
754
754
840
840
628
628
628
628
628
628
470
470
470
470
Figure- 2 Reinforcement Graph In Different Soils
CONCLUSIONS
-
Ultimately, the application of parametric analysis empowers engineers to create sustainable and effective retaining wall designs that balance safety, functionality, and cost-effectiveness. By leveraging insights gained from this study, stakeholders retaining wall systems, thereby contributing to the resilience and sustainability of infrastructure worldwide.
-
There will be the periodic increase of steel
provided in the stem and toe slabs in all the retaining walls.
-
There will be an optimum point for the heal slabs reinforcement in the retaining walls
-
The only reason is after application of different soils even though the slab is small the moment which comes through the soil will be transferred to heel slab in the process of overturning.
REFRENCES
-
Dembicki, E., & Chi, T. System analysis in calculation of cantilever retaining walls. International journal for numerical and analytical methods in geomechanics, 13(6), 599-610.(1989).
-
Kerisel, J. History of retaining wall design. In Retaining structures (pp. 1-16). Thomas Telford Publishing.(1993).
-
Goh, A. T. Behavior of cantilever retaining walls. Journal of Geotechnical Engineering, 119(11), 1751-1770. (1993).
-
Bentler, J. G., & Labuz, J. F. Performance of a cantilever retaining wall. Journal of geotechnical and geoenvironmental engineering, 132(8), 1062-1070.(2006).
-
Guler, E., Hamderi, M., & Demirkan, M. M. Numerical analysis of reinforced soil-retaining wall structures with cohesive and granular backfills. Geosynthetics International, 14(6), 330-345.(2007).
-
Sivakumar Babu, G. L., & Basha, B. M. Optimum design of cantilever retaining walls using target reliability approach. International journal of geomechanics, 8(4), 240-252.(2008).
-
Fenton, G. A., Grifths, D. V., & Williams, M. B. Reliability of
traditional retaining wall design. Geotechnique, 55(1), 55-62.(2005).
-
Lee, S., & Kim, S. K. A Study on Deformation Analysis of the Earth Retaining Wall. Journal of the Korean Geotechnical Society, 24(2), 27- 36.(2008).
-
Liu, Z., Juang, C. H., & Atamturktur, S. Confidence level-based robust design of cantilever retaining walls in sand. Computers and Geotechnics, 52, 16-27. (2018).
-
Abood, T., Eldawi, E. Y., & Abdulrahim, F. R. E. Design of cantilever retaining wall with 4m height. International Journal of Civil and Structural Engineering Research. ISSN, 3(1), 2348-7607. (2011).
-
Pei, Y., & Xia, Y. Design of reinforced cantilever retaining walls using heuristic optimization algorithms. Procedia Earth and Planetary Science, 5, 32-36.(2012).
-
Kaveh, A., & Khayatazad, M. Optimal design of cantilever retaining walls using ray optimization method. Iranian Journal of Science and Technology. Transactions of Civil Engineering, 38(C1+), 261.(2014).
-
Moon, S. W., & Ku, T. Empirical estimation of soil unit weight and undrained shear strength from shear wave velocity measurements. In 5th International Conference on Geotechnical and Geophysical Site Characterisation, ISC 2016 (pp. 1247-1252). (2016).
-
Kumar, V. N., & Suribabu, C. R. Optimal design of cantilever retaining wall using differential evolution algorithm. Int. J. Optim. Civ. Eng, 7(3), 433-49.(2017)
-
Dhamdhere, D. R., Rathi, V. R., & Kolase, P. K. Design and analysis of retaining wall. International Journal of Management, Technology and Engineering, 8(9), 1246-1263.(2018)
-
Yadav, P. A., Padade, A. H., Dahale, P. P., & Meshram, V. M. Analytical and experimental analysis of retaining wall in static and seismic conditions: A review. International Journal of Civil Engineering and Technology (IJCIET), 9(2), 522-530.(2018).
-
POUR, M. T., & Kalantari, B. Parametric analysis of back-to-back reinforced earth retaining walls. Pamukkale Üniversitesi Mühendislik Bilimleri Dergisi, 25(3), 247-256.(2019).
-
Uray, E., Çarba, S., Erkan, . H., & Tan, Ö. Parametric investigation
for discrete optimal design of a cantilever retaining wall.(2019).
-
Mittal, S., Garg, K. G., & Saran, S. Analysis and design of retaining wall having reinforced cohesive frictional backfill. Geotechnical & Geological Engineering, 24, 499-522.(2021).
-
Varga, R., lender, B., & Jelui, P. Multiparametric analysis of a
gravity retaining wall. Applied Sciences, 11(13), 6233. (2021).