
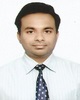
- Open Access
- Authors : Saif Azhar , Ankit Patidar , Siddharth Jaurker
- Paper ID : IJERTV9IS120266
- Volume & Issue : Volume 09, Issue 12 (December 2020)
- Published (First Online): 02-01-2021
- ISSN (Online) : 2278-0181
- Publisher Name : IJERT
- License:
This work is licensed under a Creative Commons Attribution 4.0 International License
Parametric Study of Piled Raft Foundation for High Rise Buildings
Saif Azhar1, Ankit Patidar2, Siddharth Jaurker3
1,2PG Student, Department of Civil Engineering and Applied Mechanics, Shri G.S. Institute of Technology and Science, Indore, India
3 Lecturer, Department of Civil Engineering, Government Polytechnic College, Damoh, India
Abstract The use of piled raft foundation for the high rise structures has increased recently because of its efficiency in reducing high stress concentrations, total and differential settlements of the foundation system economically. The main objective of this comparative study is to investigate the behaviour and performance of the combined piled raft foundation by changing the parameters like raft thickness, pile diameter, pile length and pile spacing. This helps in understanding the variation in total and differential settlement of the raft, maximum moment in raft and pile/raft load sharing ratios by varying the various parameters under consideration. For this purpose, a twenty storey building is modelled and analyzed in ETABS software and then foundation models with different geometrical configurations of piles and raft are prepared in SAFE software to analyze the behaviour of piled raft foundation under the loadings from the superstructure obtained from ETABS software. This parametric study is useful for deciding the suitable configuration of the piled raft foundation and designing it on the basis of various parameters.
KeywordsPiled raft foundation, SAFE software, parametric study, settlement, bearing capacity, load sharing ratio
-
INTRODUCTION
In modern era, the population is increasing rapidly which has led to the construction of high rise structures in order to accommodate large number of people in comparatively lesser land area. The foundations required for such high rise structures have to disperse very heavy loads safely to the soil and are subjected to very high stress concentrations. Moreover heavy loads and limitation of the safe bearing capacity of the soil may lead to the problem of settlement in the high rise structures. Combined Piled Raft Foundation (CPRF) is an efficient solution which is very effective in reducing differential and total settlements, when it is compared to settlements of pile and raft individually. A piled raft is a geotechnical composite construction consisting of piles, raft and soil. It can be differentiated from conventional foundation design where the loads are carried either by raft or by piles.
In piled raft foundation, a part of the load from the superstructure is carried by the raft by direct interaction with the soil beneath and the remaining load is carried by the piles by the skin friction as shown in Fig. 1. Such a foundation system incorporates the advantages of both the raft as well as the pile foundation and the use of this concept results in economical designs, better safety and performance of foundations.
Fig. 1 Load sharing in piled raft foundation
-
LITERATURE REVIEW
Randolph (1994) gave new methods to design piled raft foundations and gave more priority to settlement than capacity and put forward design approaches for finding the optimal number of piles in CPRF in order to reduce the settlement. Russo (1998) provided a numerical method, in which he assumed piles as interacting non-linear spring and raft as a thin plate for the design of piled raft foundation. Prakoso and Kulhawy (2001) used the non-linear plain strain and linear elastic finite element model to analyse piled raft foundation. Poulos (2001) suggested a three stage design method for the piled raft foundation. First stage comprises of approximate analysis to assess the effect of number of piles on load capacity and settlement. Second stage assesses the location of piles and third stage comprises of the detailed design and extensive analysis to confirm the optimum number and location of piles. Sanctis and Mandolini (2006) gave a simple method to investigate ultimate load carrying capacity of piled raft foundation from the individual capacities of its components (raft and piles) on the basis of experimental as well as finite element analysis. They found that the ultimate capacity of piled raft is at least 80% of the cumulative capacities of the individual components. Gehlot and John (2015) suggested that when rafts alone fail to fulfil the design requirements, piled raft foundations come out as an economical foundation option under such circumstances.
Different methods of analysis of piled raft foundations have been developed, but there is only limited information about comparative performances of the piled raft foundation under various parameters. An attempt is made to investigate the behaviour of piled raft foundation by varying various parameters in this study.
-
METHODOLOGY
-
Structural Modelling in ETABS
For the present study, a 20 storeyed RCC building model is considered having the plan dimensions 40m in length and 30m in width at the base. The total height of the building is 69m. The building is supposed to be provided with the combined piled raft foundation (CPRF). For the analysis of the building CSI ETABS Version 16.2.1 is used and for the analysis of piled raft foundation CSI SAFE Version 16.0.2 is used. The details of the building model are as follows:
Overall height of building – 69m Basement height – 3m
Typical storey height – 3.3m
No of storeys – 20
Slab thickness – 150mm
Beam dimensions – 300mm x 450mm
Column dimensions – 600mm x 600mm
Grade of concrete – M20 for slabs and M30 for beams and columns
Grade of steel – Fe500
The plan and 3D view of the ETABS structural model are shown in Fig. 2 and Fig. 3 respectively.
-
Loading Details
Live load considered is 2kN/m2 and floor finish load is taken as 1kN/m2. A uniformly distributed load of 10.26kN/m is considered for the wall load on the beams. Model is also subjected to seismic loadings. The seismic loads are applied to the building as per IS 1893. The building is designed as per the ductile detailing provisions of IS 13920. Various parameters selected for the seismic loading are,
Seismic Zone – Zone IV Seismic zone factor – 0.24
Type of structure – Special Moment Resisting Frame
(SMRF)
Response reduction factor- 5 Importance factor – 1
Soil type – Type II (Medium-stiff soil)
The load combinations are made as per IS 875 and IS 1893.
-
Soil Profile
Soil layer considered for this study is stiff cohesive soil having the unit weight () of 21 kN/m3, unconfined compressive strength (qu) of 220kN/m2 and average cohesion value (c) of 110kN/m2. The adhesion factor () is taken as 0.4 from the Fig.2 Annex B of IS 2911 (Part1/Sec1): 2010 corresponding to c=110kN/m2. The soil subgrade modulus
(K) is taken as 27000 kN/m3 as a suggestive value from Table 2 Appendix B of IS 2950 (Part 1):1981. Ultimate bearing capacity of the soil calculated just beneath the raft is 628.4kN/m2. Safe bearing capacity (SBC) assuming factor of
safety 2.5 is 251.4kN/m2. However as per IS 1893 SBC can be increased upto 25% for medium-stiff soils if seismic loads are considered. So the increased SBC considered is 314.3kN/m2.
Fig. 2 Plan of ETABS model
Fig. 3 3D view of ETABS model
-
Analysis of Superstructure in ETABS
The analysis of the superstructure is done in ETABS software. Linear static method is used for the seismic analysis of the structure. Slabs are assumed as the rigid diaphragms for the proper union between slabs and beams at one level. The cumulaive base reactions of the supports obtained from the analysis are shown in Table 1.
Table 1 Base reactions of the ETABS model
Load Case
Fx
Fy
Fz
Mx
My
Mz
kN
kN
kN
kN-m
kN-m
kN-m
Dead Load
0
0
168365.69
2525485.39
-3367314
0
Live Load
0
0
43680
655200
-873600
0
Floor Finish Load
0
0
21840
327600
-436800
0
Wall Load
0
0
120657.6
1809864
-2413152
0
Seismic Load in x direction
-2380.97
0
0
0
-120196.73
35714.61
Seismic Load in y direction
0
-2332.95
0
117772.31
0
-46658.97
The design sections for beams are 300mm x 450mm and for columns are 600mm x 600mm uniform throughout the height. Members are checked for the shear and flexural capacity, Beam/Column Capacity Ratios and Column P-M-M Interaction Ratios.
-
Piled-Raft Foundation Model in SAFE
The base reactions for various load cases/combinations of each support are exported to the SAFE software for analysis and design of substructure. A rectangular raft of length 44m and width 34m is chosen for the structure having a clear projection of 2m from the face of edge columns in each side. The minimum thickness of raft for various models is taken as 1m as per IS 2950. The raft is modelled as thick plate to consider the shear effects and M30 grade of concrete is chosen for it. Mesh size of 0.5m is considered for the raft analysis. Soil beneath the raft is modelled as area springs having subgrade modulus K to simulate the effects of the soil layer. The piles are modelled as point springs having the equivalent spring stiffness equal to EA/L where E denotes the modulus of elasticity of concrete, A is the area of cross section of the pile and L is the length of the pile. The grade of concrete chosen for piles is M35. The minimum spacing of
2.5 times the diameter of pile is adopted according to IS 2911. Each location of pile is attached with a point spring to simulate the behaviour of piles. Piled raft model in SAFE software is shown in Fig. 4.
Fig. 4 Piled raft model in SAFE
-
-
PARAMETRIC STUDY
The purpose of this parametric study is to investigate the performance and behaviour of the combined pile raft
foundation by changing the parameters like raft thickness, pile diameter, pile length and pile spacing. This helps to understand the variation in settlement, differential settlement of the raft, maximum moment in raft, pile/raft load sharing ratios etc by varying the parameters. For this study, four types of cases are considered which are as follows:
Case I Raft thickness is varied from 0.5m to 3m for pile lengths of 20m and 30m. The pile diameter is kept constant at 0.6m for all the models. Total 22 models are prepared in this case.
Case II Pile diameter is varied from 0.3m to 2.4m for raft thickness of 1m, 1.5m and 2m. The spacing of piles in all the models is kept constant at 5m centre to centre. Total 24 models are prepared in this case.
Case III Pile spacing is varied from 2.5D to 6D (D is the diameter of pile) for the pile diameters of 0.3m, 0.6m and 0.9m. The raft thickness is kept constant at 1.5m in all the models. Total 24 models are prepared in this case.
Case IV Pile length is varied from 10m to 45m for the pile diameters of 0.6m, 0.9m and 1.2m. The raft thickness is kept constant at 1.5m in all the models. Total 24 models are prepared in this case.
Total 94 models are prepared for this parametric study by changing the configurations and geometry of the piles and raft.
-
RESULTS AND DISCUSSIONS
-
Effect of varying raft thickness
The results of Case I studies are shown in Fig. 5 to 9. Fig. 5 shows the variation in maximum pressure under the raft for pile lengths of 20m and 30m on changing the raft thickness from 0.5m to 3m. It has been found from analysis that maximum pressure decreases upto raft thickness of 1.25m and then it remains somewhat constant on further increment in thickness. Maximum pressure under the raft is well below the safe bearing capacity of soil. Similarly maximum settlement of the raft (Fig. 6) decreases only upto a limit and then the curve flattens up. Fig. 7 shows the variation of maximum differential settlement with change in raft thickness. It is evident that it decreases upto a limit (around raft thickness of 1.75m) and then increases. These results show that increasing raft thickness beyond a certain limit (1.25m to 1.75m) does not influence the studied parameters much and rather results in uneconomical sections. Fig. 8 shows that the maximum moment in the raft increases on increasing the raft thickness. Fig. 9 shows that the variation of Pile/Raft load sharing ratio on increasing the raft thickness remains constant with only slight increment in the beginning. It is evident that majority of load as much as approximately 88% in case of pile length of
20m and approximately 83% in case of pile length of 30m is carried by the piles.
Fig. 5 Effect of raft thickness on maximum pressure
Fig. 6 Effect of raft thickness on maximum settlement
Fig. 7 Effect of raft thickness on differential settlement
Fig. 8 Effect of raft thickness on maximum moment
Fig. 9 Effect of raft thickness on Pile/Raft load sharing ratio
-
Effect of varying pile diameter
The results of Case II studies are shown in Fig. 10 to 14. In this case piles of various diameters are analysed placing them at the fixed spacing of 5m centre to centre. Fig. 10 shows the effect of varying pile diameter on maximum pressure under the raft. Maximum pressure decreases with increase in pile diameter for all the raft thicknesses under consideration. Maximum pressure under the raft for pile groups of 0.3m and 0.6m diameters exceeds the safe bearing capacity of the soil. Maximum settlement and maximum differential settlement of the raft also decreases with the increase in pile diameter as shown in Fig. 11 and 12. Fig. 13 shows that the Pile/Raft load sharing ratio increases with the increase in pile diameter since the equivalent spring stiffness of the piles increases which attract more loads. Fig. 14 shows that the load carried by raft decreases drastically from approximately 85% for pile diameter of 0.3m to as low as 10% for pile diameter of 2.4m and almost 90% of the load is carried by piles with diameter 2.4m.
Fig. 10 Effect of pile diameter on maximum pressure
Fig. 11 Effect of pile diameter on maximum settlement
Fig. 12 Effect of pile diameter on maximum differential settlement
Fig. 13 Effect of pile diameter on Pile/Raft load sharing ratio
Fig. 14 Effect of pile diameter on load carried by raft
-
Effect of varying pile spacing
The results of Case III studies are shown in Fig. 15 to 19. In this case the centre to centre spacing of the piles is varied from 2.5D to 6D which is 0.75m to 1.80m, 1.50m to 3.60m and 2.25m to 5.40m for the pile diameters of 0.3m, 0.6m and 0.9m respectively. Fig. 15 shows that the maximum pressure under the raft increases almost linearly with increasing pile spacing from 2.5D to 6D but is well below the safe bearing capacity of the soil in all the cases. Similarly the maximum settlement an maximum differential settlement of the raft also increases linearly with increase in pile spacing. Fig. 18 shows the variation of Pile/Raft load sharing ratio with increase in pile spacing. The ratio varies from approximately
0.88 to 0.55 with increasing pile spacing for all the pile diameters under consideration. Fig. 19 shows that the load carried by the raft increases from average value of 12% for 2.5D spacing to the average value of about 45% for 6D spacing.
Fig. 15 Effect of pile spacing on maximum pressure
Fig. 16 Effect of pile spacing on maximum settlement
Fig. 17 Effect of pile spacing on maximum differential settlement
Fig. 18 Effect of pile spacing on Pile/Raft load sharing ratio
Fig. 19 Effect of pile spacing on load carried by raft
-
Effect of varying pile length
The results of Case IV studies are shown in Fig. 20 to 25. In this case pile lengths are varied from 10m to 45m for a constant raft thickness of 1.5m. Further three different pile diameters of 0.6m, 0.9m and 1.2m are considered in this case for studying the variation in parameters. Fig. 20 shows that the maximum pressure under the raft decreases with the increase in pile length. Similarly it is clear from Fig. 21 and 22 that the maximum settlement and maximum differential settlement of the raft decreases almost linearly with the increase in pile length. Also these parameters vary almost in the similar manner for all the pile diameters under consideration and their curves are very close to each other. Fig. 23 shows the variation of maximum moment in raft with change in pile length. It has been observed that the moment decreases only by a small amount on increasing pile length for a specific pile diameter. Also the moment is found to be maximum in case of pile diameter of 0.9m. Fig. 24 shows that the Pile/Raft load sharing ratio increases with increase in pile length. This means piles of larger length carries more load from the superstructure than the piles of smaller length. Fig. 25 shows the decrease in load carried by the raft on increasing pile length. However the decrease in load is from 18.92% to 5.32%, 24.61% to 7.14%
and 24.42% to 7.31% only for pile diameters of 0.6m, 0.9m and 1.2m respectively on increasing the pile length from 10m to 45m. This means an increase in pile length of 35m results in a mere increase in load carried by the pile group.
Fig. 20 Effect of pile length on maximum pressure
Fig. 21 Effect of pile length on maximum settlement
Fig. 22 Effect of pile length on maximum differential settlement
Fig. 23 Effect of pile length on maximum moment
Fig. 24 Effect of pile length on Pile/Raft load sharing ratio
Fig. 25 Effect of pile length on load carried by raft
-
-
CONCLUSIONS
An extensive comparative study is performed by analyzing 94 combined piled raft foundation models in SAFE software.
Based on the results of this parametric study, the following conclusions can be drawn:
-
Maximum pressure, settlement and differential settlement decreases only upto a limit on increasing raft thickness. After that increasing raft thickness has no significant benefit. Rather the maximum moment increases with increasing raft thickness and may lead to uneconomical sections and higher steel demand.
-
The load carried by the raft decreases prominently with the increase in pile diameter.
-
Larger pile diameter reduces maximum pressure, settlement and differential settlement. However the reduction in these values is very less as we go on increasing the pile diameter beyond a limit. Larger diameter will therefore result in higher cost as compared to its benefits.
-
Increasing pile spacing results in increase in maximum pressure, settlement and differential settlement. The resulting curves are almost similar for all the pile diameters considered. Thus smaller diameter must be selected to reduce the cost.
-
Settlements and maximum pressure under the raft decreases with the increase in pile length. The load carried by pile group increases with increase in pile length but this increment is not much significant as compared to the added cost of larger pile length.
-
Piled raft foundation is a better solution to control settlements however the most economical and optimum geometrical configuration must be adopted for the enhanced performance of such foundation system.
REFERENCES
-
Reul, O., and Randolph, M.F. (2004) Design strategies for piled rafts subjected to nonuniform vertical loading J. Geotech. Geoenviron. Eng, 130, Issues 1, pp 113.
-
Poulos, H. G. (2001) Piled raft foundation: design and applications. Geotechnique, 51(2), pp. 95-113.
-
Prakoso, W. A. and Kulhawy, F.H. (2001) Contribution to piled raft foundation design. Journal of Geotechnical and Geoenvironmental Engineering, ASCE, 127(1), pp.17-24.
-
Randolph, M. F. (1994) Design methods for pile groups and piled rafts. 13th International Conference for Soil Mechanics and Foundation Engineering, New Delhi, 5, pp. 61-82.
-
Sanctis, L. D. and Mandolini, A. (2006) Bearing capacity of the piled rafts on soft clays. Journal of Geotechnical and Geoenvironmental Engineering, ASCE, 132(12), pp. 1600-1610.
-
Russo, G. (1998) Numerical analysis of piled rafts. International Journal for Numerical and Analytical Methods in Geomechanics, 22(6), pp. 477-493.
-
Gahlot, R and John, R.J. (2015) Approximate analysis of piled raft. International Journal of Scientific & Engineering Research, Volume 6, Issue 12, December-2015.