
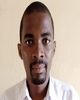
- Open Access
- Authors : S. I. Ibuodinma , O. J. Onojo , L. O Uzoechi
- Paper ID : IJERTV10IS010282
- Volume & Issue : Volume 10, Issue 01 (January 2021)
- Published (First Online): 13-02-2021
- ISSN (Online) : 2278-0181
- Publisher Name : IJERT
- License:
This work is licensed under a Creative Commons Attribution 4.0 International License
Performance Analysis of Utility Scale Photovoltaic Systems Integrated Into An Islanded Nigeria Electric Power Grid
S.I. Ibuodinma, O.J. Onojo, L.O Uzoechi
Department of Electrical and Electronic Engineering Federal University of Technology,
Owerri, NIGERIA.
Abstract:- This paper is on the performance analysis of photovoltaic (PV) system integrated into an islanded Nigeria electricity grid. Electricity supply in Nigeria is abysmal and the effects on the economy and quality of life of Nigerians. This work carried out load flow studies of a part of the existing network, namely Afam Power Substation to Yenegoa Transmission Substation and the network with utility scale PV system integrated. Contingency analysis of the system was carried out using a set of possible contingency scenarios. PowerWorld Simulator was deployed in carrying out these analyses. With the incorporation of PV systems, stability was achieved when there was loss of generation. For example, when Afam Generation station was opened, system violations went from 14 (without PV) to 3 (with PV). Finally, the levelized cost of electricity (LCoE) for combined cycle gas turbines and PV systems was conducted. The LCoE for PV systems was 0.08985$/KWh which is less than LCoE of 0.245$/KWh as at 2013. The results also show that such hybrid generation planning can provide a high degree of redundancy in our fragile network.
Keywords: Utility Scale PV systems, Power Flow Analysis, Contingency analysis, PowerWorld.
1.0 INTRODUCTION
The issue of electric power demand supply in Nigeria has been seemingly intractable over the years. Several government and industry players have done a lot to improve the system, yet outcomes remain abysmal. Sambo (2008) showed that based on the so- called reference scenario, at 7% GDP growth, electricity demand projections for Nigeria is expected to peak at 119,200MW in the year 2030. With a base year of 2005, this would have implied a yearly addition of 4,538MW. It is estimated the installed capacity of off grid generation in Nigeria is about 14 GW (African-EU Renewable Energy Corperation Programme, 2016). This capacity exceeds the overall installed capacity. It has been shown that with average generation of 4000 MW, the per capita consumption is 0.03KW, a very poor performance (Ezirim, Eke, & Onuoha, 2016). Many manufacturing industries rely mostly on off grid generators for their electricity needs. In addition, overall, electricity consumption only accounts for one fifth of our final energy demand today, but contributes about 40% of energy related CO2 emission. (International Energy Agency, 2018). Therefore, to improve generation capacity in Nigeria and reduce carbon emission it will be necessary to deploy as
many as possible renewable energy sources in our energy mix.
Utility scale solar power plants are energy generating facilities capable of producing large amounts of electricity that can be fed into the electricity transmission grid; they range in size from 10 MW to over 200 MW(Xoubi, 2015). The integration of photovoltaic systems into the grid is gaining more traction today as a very important application of PV systems. According to levelized cost studies conducted by Lazard, an international financial advisory and asset management firm, the mean levelized cost of energy of utility-scale PV technologies is down approximately 13% from 2017 and the mean levelized cost of energy of onshore wind has declined almost 7% (Lazard, 2018). Utility scale photovoltaic systems promise to be a viable option in ramping up generation capacity of Nigeria, while protecting the environment.
Nigeria has recorded a total of 18 total system collapse and 6 partial system collapse between 2018-2019. The Nigeria National Grid is highly vulnerable to voltage instability. It was observed that the high rate of system collapse is largely due to faults (technical challenges) on the network, the state of the power system equipment and to some extent political issues (Samuel, Katende, Daramola, & Awelewa, 2014).
This reinforces the need for intentional power system islanding. Islanding refers to the condition in which a portion of the grid becomes temporarily isolated from the main grid but remains energized by its own distributed generation resource(s). Islanding may occur accidentally or deliberately. Traditionally, islanding has been seen by utilities as an undesirable condition due to concerns about safety, equipment protection, and system control(Greacen, Engel, Quetchenbach, & Berkeley, 2013). Islanding operation can be intentional and may be desired in cases where the central grid is prone to reliability problems. This reflects the Nigeria electric power scenario.
There is the need to conduct thorough analysis before integrating alternative and variable PV systems. In doing this, Powerworld a userfriendly and highly interactive power system analysis and visualization platform is employed. It integrates many commonly performed power system tasksContingency Analysis, TimeStep Simulation, OPF, ATC, PVQV, Fault Analysis, SCOPF, Sensitivity
Analysis, Loss Analysis, Transient Stability, etc (PowerWorld Corporation, 2019).
-
METHODOLOGY
-
Procedure for the project.
The algorithm for the project is as stated below;
-
Collection of data: This involves the data of the generation stations, transmission lines, inter-bus transformers and connected loads.
-
Model system in PowerWorld: This will include creating models of the network the system without Utility Scale PV systems and with PV systems integrated.
-
Carry out load flow analysis of the system: This will include load flow analysis of the system
-
Without PV systems integrated
-
With PV systems integrated
-
-
Develop a contingency list.
-
Conduct contingency analysis.
-
Carry out cost analysis of the different plants.
-
-
Data Collection.
The data used for this study are generation Stations, inter- bus transformers and transmission line data. These are shown in Tables 1, 2 and 3.
Table 1: Generation station Voltage and power ratings.
S/N
Generating Stations
Voltage (kV)
Power (MW)
1
AFAM PS
330
1396
2
GBARAIN PS
132
120
Table 2: Data of the inter-bus transformers
S/N
T r a n s f o r m e r s
Power (MVA)
Reactance P,u
% impeda nc e
1
330/132kV Tx @Alaoji (T2)
150
0.07573
11.36
2
330/132kV Tx @Alaoji (T3)
150
0.07573
11.36
3
330/132kV Tx @Alaoji (T4)
300
0.04523
13.57
4
330/132kV Tx @Afam (T1)
164
0.07074
11.46
5
330/132kV Tx @Alaoji (T2)
150
0.07573
11.36
Table 3: Transmission line data.
S/N
Lines
Voltage kV
Power MW
Len KM
R p.u
X p.u
1
Afam—Alaoji L1
330
400
25
0.0009825
0.0073898
2
Afam—Alaoji L2
330
400
25
0.0009825
0.0073898
3
Afam—Alaoji L1
132
300
30
0.0047001
0.058801
4
Afam—Alaoji L2
132
300
30
0.0047001
0.058801
5
AlaojiPH Main L1
132
144
37
0.0002121
0.0770005
6
AlaojiPH Main L2
132
144
37
0.0002121
0.0770005
7
AlaojiAba L1
132
100
7
0.0011204
0.01412
8
AlaojiAba L2
132
100
7
0.0011204
0.01412
9
AlaojiUmuahia L1
132
80
57
0.0022401
0.016845
10
AlaojiUmuahia L2
132
80
57
0.0022401
0.016845
11
AlaojiOwerri L1
132
100
60
0.0112947
0.1412004
12
AlaojiOwerri L2
132
100
60
0.0112947
0.1412004
13
Owerri—Ahoada L1
132
100
74
0.0029084
0.021869
14
Owerri—Ahoada L2
132
100
74
0.0029084
0.021869
15
Ahoada—Gbarain L1
132
50
37.5
0.0014542
0.0109345
16
Ahoada—Gbarain L2
132
50
37.5
0.0014542
0.0109345
17
GbarainYenogoa L1
132
50
37.5
0.0014542
0.0109345
18
GbarainYenogoa L2
132
50
37.5
0.0014542
0.0109345
-
Load Flow Analysis.
The derivation of power flow equations for power system networks are shown in Fig. 1.
Vi = Vii, V = Vi i and Yik = Yikik, (2.8)
i
i
The real and reactive powers in polar form are expressed
Vi Real power = Pi = V n Yik Vk cos (ik + k i)
yi1 V1
i
(2.9)
k=1
Reactive power = Qi = V n Yik Vk sin (ik + k i)
V2 i k=1
yi2
Ii
yin
yio
(2.10)
Due the non-linearity of the above equations, numerical methods are employed in the evaluation of the solutions to the
Vn equations. Several techniques exist like the Newton Raphson,
Gauss Seidel and the Fast Decouple methods. The Newton Method is employed in this project.
-
PowerWorld Load Flow Simulation
The PowerWorld model of the islanded network and the modified network are shown in Figs. 2 and 3.
Figure 1: Typical Power System Network The current entering the ith bus is given as
Ii = yioVi + yi1(Vi V1) + yi2(Vi V2) + + yik(Vi Vk)
(2.0)
Collecting like terms in equation 2.0, we have,
1
GS
AFAM
PH Main 132kV SS
4
3
T2
T3 T4
5
ABA
6 132kV SS
Owerri 132kV SS
Ahaoda
9 132kV SS Yenogoa 132kV SS
Ii = (yio
+ yi1
+ yi2
+ + yik
)Vi yi1 V1
yi2 V2
T1 T2 PS 330kV
Alaoji 330kV/132kV
10
8 Ahaoda 11 12
132kV SS GS
7
yikVk (2.1)
2
AFAM PS 132kV
Umuahia 132kV SS
GBARAIN PS 132kV
er
d
er
d
The term (yio + yi1 + yi2 + + yik) is the self-admittance and the coefficients of V1, V2, Vk are the mutual admittances.
We simplify equation (2.1) as
Figure 2: Single Line Diagram of the network
PH Main 132kV SS
5
ABA
6 132kV SS
4
k=1
k=1
Ii = n
Yik Vk; i = 1,2 , n (2.2)
1 3 Owerri
T3
T4
T3
T4
T2 132kV SS
Ahaoda
9 132kV SS
Yenogoa 132kV SS
The complex power injected into the ith bus of the pow
system is
i
i
Si = Pi + jQi = ViI ;where i = 1,2 , n (2.3)
Where Vi is the ith bus voltage with respect to the earth an
i
i
I is the conjugate of the current . The power conjugate
GS
AFAM PS 330kV
PVGS
2
AFAM PS 132kV
Alaoji 330kV/132kV
8
7
Umuahia 132kV SS
10
Ahaoda
132kV SS
PVGS
11
12
GS
GBARAIN PS 132kV
therefore becomes,
i
i
Si = Pi Qi = VIi ; where i = 1,2 …, n (2.4) Substituting into eqn 2.4
Figure 3: Single Line Diagram of the modified network
The data of the various part of the network were input in the PowerWorld Simulator. This was used to calculate the voltages, power flows, the voltage angles of the busses. The
S = P jQ = V n Y V ; where i = 1,2 ,, n
PowerWorld base settings include
i i i i k=1 ik k
(2.5)
Separating the real and reactive components of the above equation
-
Base MVA = 100
-
Voltage tolerance = ±5%
Real power = Pi = Re {V n Yik Vk} (2.6)
i k=1
-
Maximum number of iterations = 100
Real power = Si = Im {V n Yik Vk} (2.7)
i k=1
-
Range of Voltage angle= ± 10°
The Voltage and the admittance in polar form as given as
-
Power Factor = 0.8
-
Slack bus voltage and angle = 1.05 0.00°
-
Slack Bus = Afam 330kV
-
Machine models: REG-C (Renewable Energy
+ Fuel Price * Heat Rate (2.11)
CRF = capital recovery factor, turning capital costs into annual values (if capital is financed at discount rate D)
Fixed O & M = Fixed Operation and maintenance Costs. Variable O & M = Variable Operation and maintenance Costs. T= Tax rate, DPv = Depreciation Factor
Generator- Controller) for PV generators and GENTPF for Combined Cycle Gas Turbine.
-
-
Contingency Analysis
CRF = D (1+D)
N
N
(1+D)N1
D = Discount rate
N= Lifetime of System.
(2.12)
The cost components for evaluating LCOE are itemized in Table 4.
Since the Photovoltaic system is a still a budding development,
it is crucial to explore the behaviour of the system under anTable 4: Cost Components of the LCoE for CCGT and PV unexpected or planned system outage to detect the weakness thatsystems(Roche, Ude, & Donald-Ofoegbu, 2017)
PARAMETERS
UNIT
CCGT
Photovoltaic
Capital Cost
$/KW
1000
1150
Fixed O & M
$/KW-Yr
/td>
15.50
17.30
Variable O & M
$/KWh
0.006
0
Capacity Factor
%
80
19
Discount Rate
%
11
11
Fuel Cost
$/MMBtu
7
0
Depreciation
%
10
10
Tax Rate
%
5
0
Heat Rate
Btu/KWh
11039
0
PARAMETERS
UNIT
CCGT
Photovoltaic
Capital Cost
$/KW
1000
1150
Fixed O & M
$/KW-Yr
15.50
17.30
Variable O & M
$/KWh
0.006
0
Capacity Factor
%
80
19
Discount Rate
%
11
11
Fuel Cost
$/MMBtu
7
0
Depreciation
%
10
10
Tax Rate
%
5
0
Heat Rate
Btu/KWh
11039
0
the network will bear. Contingencies can exist as the result of the outages of the system elements such as: generator, network transmission lines and system transformer for the purpose this work, we focus on the effect of outages of generating stations, particularly with the PV systems integrated and the effect of variability of the photovoltaic source on the system.
-
Contingency List
The contingency list is set in order to identify the effect of contingencies on the system operation and to experience which critical contingencies causes most violation of the system.(Alex, 2015) In this project, the contingencies tested are
-
Open Afam 330kV power station without the photovoltaic systems
Assuming N = 30 years and calculating the LCoE using equation
-
Open Gbarain 132kV power station without th(e3.18)
30
30
photovoltaic systems
-
For combined cycle gas turbine.
-
-
Open Afam 330kV power station with the photovoltaic systems
CRF = 0.11(1.11) = 0.1150
(1.11)301
LCoE =( 10000.1150(1(0.050.1))) + ( 15.50
)+ 0.006 +
87600.8(10.5) 87600.8
-
Open Gbarain 132kV power station with the
photovoltaic systems
( 7
106
* 11039 ) = 0.1027$/KWh
-
Zero Insolation at Afam 132kV Photovoltaic Station
-
For PV systems
LCoE = ( 11500.1150(1(00.1))) + ( 17.30
)+ 0 + (0 * 0 )
-
-
Zero Insolation at Gbarain 132kV Photovoltaic Station
87600.19(10)
= 0.08985 $/KWh
87600.19
The PowerWorld simulator was used for the simulation of each of the contingencies.
-
-
Cost Analysis
The levelized cost of electricity (LCoE) was evaluated for the two different power generation sources namely natural gas and solar energy. The Nigerian Electricity Regulation Council employs the US Department of Energy method of calculating LCOE. The formula is given by equation 11.
-
RESULTS AND DISCUSSION
This chapter presents results and discussion of the of the objectives in chapter 3:
-
Load flow analysis of the network without PV system
-
Load flow analysis of the network with PV system integrated
-
Contingency analysis of the network without PV system integrated.
-
Contingency analysis of the network with PV system integrated.
-
Cost Analysis of the system.
LCOE = Capital CostCRF(1TDpv )
8760Capacity factor(1T)
Variable O &M
+ Fixed O & M +
8760Capacity Factor
-
-
Results from Load Flow Analysis of The Network Without PV System
From the results of the load flow analysis of the system as shown in Table 5, the following is deduced.
-
All the bus voltage magnitudes are within the acceptable limit of ±5%
Voltage p.u
Voltage p.u
-
There are violations of buses 8, 9, 10, 11, 12, which are not within the ± 10° range. This is explained by the fact that Gbarain PS is not sufficient to boost the real and reactive power supply at that end and there are reactors installed at the downstream station for compensation of losses. Hence the need for a system that can boost supply and compensate for losses. The Photovoltaic system comes as a viable option.
-
The major active and reactive power demand is on Afam 330kV.
-
which will definitely have impact on system stability. Therefore, the system is always close to the tipping point and this explains clearly the partial collapses often experienced in this segment. The graphical representation of the bus voltage and voltage angle profile in Figs. 4 and 5 further explains the results.
-
-
Results from Load Flow Analysis of the Network with PV System
The results in Table 6 and Figs. 6 and 7 show a clear improvement when the PV systems were integrated into the select segment of the grid. The following can be clearly seen.
-
All the bus voltage magnitudes are within the acceptable limit of ±5%, even with more improvement in values.
-
There are no violations of buses angles as all are within the ± 10° range. This is due to the fact that the PV systems assisted in improving the bus angle by supplying more active power.
Bus No
Name
Pu Volt
Ang. Deg
Gen MW
Gen MVar
1
Afam PS
1.05
0.00
537.8
225.97
3
Alaoji TS
1.04238
-0.72
2
Afam PS
1.03063
-2.82
4
Alaoji PS
1.01356
-5.10
7
Umuahia TS
1.01151
-5.39
5
Aba TS
1.01006
-5.68
6
PH Main TS
0.998
-8.24
11
Gbarain PS
0.96317
-12.02
120
30
10
Ahaoda TS
0.96164
-12.07
8
Owerri TS
0.96089
-12.28
12
Yenogoa TS
0.96286
-12.36
9
Ahoada TS
0.95593
-13.12
Bus No
Name
Pu Volt
Ang. Deg
Gen MW
Gen MVar
1
Afam PS
1.05
0.00
537.8
225.97
3
Alaoji TS
1.04238
-0.72
2
Afam PS
1.03063
-2.82
4
Alaoji PS
1.01356
-5.10
7
Umuahia TS
1.01151
-5.39
5
Aba TS
1.01006
-5.68
6
PH Main TS
0.998
-8.24
11
Gbarain PS
0.96317
-12.02
120
30
10
Ahaoda TS
0.96164
-12.07
8
Owerri TS
0.96089
-12.28
12
Yenogoa TS
0.96286
-12.36
9
Ahoada TS
0.95593
-13.12
Table 5: Results of the PowerWorld Load Flow Simulation without PV.
Bus Voltage Profile
1.2
1
0.8
0.6
0.4
0.2
0
Bus Name
Bus Voltage Profile
1.2
1
0.8
0.6
0.4
0.2
0
Bus Name
Ahaoda
Yenogoa Owerri Ahaoda Gbarain PH Main Aba Umuahia Alaoji PS Afam PS Alaoji PS
Afam PS
Ahaoda
Yenogoa Owerri Ahaoda Gbarain PH Main Aba Umuahia Alaoji PS Afam PS Alaoji PS
Afam PS
Figure 4: Bar chart of the Bus voltages without PVsystem.
Voltage Angle Profile
0.00
-2.00
-4.00
-6.00
-8.00
-10.00
-12.00
-14.00
Bus Name
Voltage Angle Profile
0.00
-2.00
-4.00
-6.00
-8.00
-10.00
-12.00
-14.00
Bus Name
Ahaoda 132kV SS
Yenogoa 132kV SS Owerri 132kV SS Ahaoda 132kV SS Gbarain PS 132kV PH Main 132kV SS Aba 132kV SS Umuahia 132kV SS Alaoji PS 132kV Afam PS 132kV Alaoji PS 132kV
Afam PS 330kV
Ahaoda 132kV SS
Yenogoa 132kV SS Owerri 132kV SS Ahaoda 132kV SS Gbarain PS 132kV PH Main 132kV SS Aba 132kV SS Umuahia 132kV SS Alaoji PS 132kV Afam PS 132kV Alaoji PS 132kV
Afam PS 330kV
Angle deg.
Angle deg.
Figure 5: Bar chart of Voltage angles without PV system.
Table 6: Results of the PowerWorld Load Flow Simulation with PV.
Bus No
Name
Nom kV
PU Volt
Volt (kV)
Angle (Deg)
1
Afam PS 330kV
330
1.05
346.5
0
2
Afam PS 132kV
132
1.03328
136.392
2.46
3
Alaoji TS 132kV
330
1.04438
344.646
-0.24
4
Alaoji TS 132kV
132
1.0173
134.283
-1.85
5
Aba 132kV TS
132
1.0138
133.822
-2.43
6
PH Main 132kV TS
132
1.0018
132.238
-4.97
7
Umuahia 132kV TS
132
1.01525
134.013
-2.14
8
Owerri 132kV TS
132
0.97648
128.895
-4.00
9
Ahaoda 132kV SS
132
0.97086
128.153
-4.82
10
Ahaoda 132kV TS
132
0.98055
129.433
-2.23
11
Gbarain PS 132kV
132
0.98171
129.586
-1.79
12
Yenogoa 132kV TS
132
0.97948
129.291
-2.12
Bus No
Name
Nom kV
PU Volt
Volt (kV)
Angle (Deg)
1
Afam PS 330kV
330
1.05
346.5
0
2
Afam PS 132kV
132
1.03328
136.392
2.46
3
Alaoji TS 132kV
330
1.04438
344.646
-0.24
4
Alaoji TS 132kV
132
1.0173
134.283
-1.85
5
Aba 132kV TS
132
1.0138
133.822
-2.43
6
PH Main 132kV TS
132
1.0018
132.238
-4.97
7
Umuahia 132kV TS
132
1.01525
134.013
-2.14
8
Owerri 132kV TS
132
0.97648
128.895
-4.00
9
Ahaoda 132kV SS
132
0.97086
128.153
-4.82
10
Ahaoda 132kV TS
132
0.98055
129.433
-2.23
11
Gbarain PS 132kV
132
0.98171
129.586
-1.79
12
Yenogoa 132kV TS
132
0.97948
129.291
-2.12
Voltage
Voltage
1
0.8
0.6
0.4
0.2
0
Bus Low Volts
Bus(1)
0.567
0.95
59.75
Bus Low Volts
Bus(2)
0.567
0.95
59.75
Bus Low Volts
Bus(3)
0.567
0.95
59.75
Bus Low Volts
Bus(4)
0.567
0.95
59.75
Bus Low Volts
Bus(5)
0.561
0.95
59.13
Bus Low Volts
Bus(6)
0.537
0.95
56.61
Bus Low Volts
Bus(7)
0.564
0.95
59.39
Bus Low Volts
Bus(8)
0.829
0.95
87.35
Bus Low Volts
Bus(9)
0.823
0.95
86.64
Bus Low Volts
Bus(1)
0.567
0.95
59.75
Bus Low Volts
Bus(2)
0.567
0.95
59.75
Bus Low Volts
Bus(3)
0.567
0.95
59.75
Bus Low Volts
Bus(4)
0.567
0.95
59.75
Bus Low Volts
Bus(5)
0.561
0.95
59.13
Bus Low Volts
Bus(6)
0.537
0.95
56.61
Bus Low Volts
Bus(7)
0.564
0.95
59.39
Bus Low Volts
Bus(8)
0.829
0.95
87.35
Bus Low Volts
Bus(9)
0.823
0.95
86.64
Bus Name
Gbarain PS
Gbarain PS
Ahaoda
Ahaoda
Owerri
Owerri
Ahaoda
Ahaoda
Umuahia
Umuahia
Yenogoa
Yenogoa
Aba 132kV SS
Aba 132kV SS
PH Main
PH Main
Alaoji TS
Alaoji TS
Afam PS
Afam PS
Figure 6: Bar chart of the Bus voltages with PV System.
0
-2
-4
-6
-8
-10
-12
-14
Bus Name
0
-2
-4
-6
-8
-10
-12
-14
Bus Name
Angle Deg
Angle Deg
Figure 7: Bar chart of the Bus voltages without PV System.
-
-
Results from Contingency Analysis of the Network Without PV System
The contingency lists and the results of the simulations are shown in Tables 7, 8 and 9.
The results above registered 14 violations when Afam PS was suddenly lost. The violations are severe as seen from the 9 bus voltage violations and 5 transmission line violations, thus whenever Afam PS is lost, the segment must collapse. Again, losing Gbarain PS recorded 5 bus voltage violations which exceeded the ±5% voltage limits and overloaded Alaoji-Owerri line with above 30%, this could lead to loss of that transmission line thus compromising the system. Thus, this network as is, requires further redundancies as this contingency tested can trigger a system collapse of the entire grid.
VIOLATION SUMMARY WHEN AFAM 330kV PS WAS OPENED WITHOUT PV
Category
Element
Value
Limit
Percent
Branch MVA
Bus(4)-Bus(8)
247.2
125
197.82
Branch MVA
Bus(4)-Bus(8)
247.2
125
197.82
Branch MVA
Bus(8)-Bus(10)
779.8
125
623.85
Branch MVA
Bus(10)-Bus(11)
404.3
62.5
646.99
Branch MVA
Bus(10)-Bus(11)
404.3
62.5
646.99
VIOLATION SUMMARY WHEN AFAM 330kV PS WAS OPENED WITHOUT PV
Category
Element
Value
Limit
Percent
Branch MVA
Bus(4)-Bus(8)
247.2
125
197.82
Branch MVA
Bus(4)-Bus(8)
247.2
125
197.82
Branch MVA
Bus(8)-Bus(10)
779.8
125
623.85
Branch MVA
Bus(10)-Bus(11)
404.3
62.5
646.99
Branch MVA
Bus(10)-Bus(11)
404.3
62.5
646.99
Table 7: Results of the Power World contingency analysis without PV system
Table 8: Results of the Power World contingency analysis without PV system
VIOLATION SUMMARY WHEN AFAM 330kV PS WAS OPENED WITHOUT PV
Category
Element
Value
Limit
Percent
Bus Low Volts
Bus(12)
0.8661
0.95
96.23
Bus Low Volts
Bus(8)
0.882
0.95
98
Bus Low Volts
Bus(9)
0.8757
0.95
97.31
Bus Low Volts
Bus(10)
0.8712
0.95
96.8
Bus Low Volts
Bus(11)
0.8686
0.95
96.51
Branch MVA
Bus(4)-Bus(8)
173.07
125
138.46
Branch MVA
Bus(4)-Bus(8)
173.07
125
138.46
-
Results from Contingency Analysis of the Network with PV System
The contingency lists and the results of the simulations are shown in Table 9.
The results showed improvements when the Afam 330kV PS was opened. The following could be deduced.
-
There were only 3 violations which is far removed from the 13 violations recorded without PV system.
-
There were no bus voltage violations.
-
The percentage violations of the transmissions are negligible compared to the results got without PV systems. These line overload can be remedied by simply increasing line capacities.
Hence, the PV systems will provide a high degree of stability when there is outage of any of the generation plants. As expected, no violations occurred when Gbarain PS was lost.
Table 9: Results of the Power World contingency analysis with PV system
VIOLATION SUMMARY WHEN GBARAIN 132 kV PS WAS OPENED WITH PV
Category
Element
Value
Limit
Percent
Branch MVA
Ahaoda(10)- Owerri(8)
135.79
125
108.63
Branch MVA
Gbarain(11)- Ahaoda(10)
67.98
62.5
108.76
Branch MVA
Gbarain(11)- Ahaoda(10)
67.98
62.5
108.76
-
-
Results from Contingency Analysis of the Network when there is Zero Insolation at the PV Power Stations
The basic challenge faced with PV systems is the variability of the source. The results here tested the effect of losing any of the PV systems. The violations observed in Table 10 are transmission lines overload occasioned by the demand for more active power from
-
Gbarain PS when there was zero insolation at Afam 132kV PVGS, Owerri- Ahoada line2 and Gbarain-Ahoada Line1&2 being the trunk lines in this case (see appendix for branch flows).
Table 10: Results of the Power World contingency analysis without PV system
Violations when there is Zero insolation at AFAM PVGS
Category
Element
Value
Limit
Percent
Branch MVA
Ahaoda TS ( 10) –
Owerri TS (8)
135.79
125
108.63
Branch MVA
Gbarain PS
(11) –
Ahaoda SS (10)
67.97
62.5
108.76
Branch MVA
Gbarain PS
(11) –
Ahaoda SS (10)
67.97
62.5
108.76
Violations when there is Zero insolation at GBARAIN PVGS
Branch MVA
Alaoji TS(4)
– Owerri TS (8)
163.88
125
131.1
Branch MVA
Alaoji TS (
4) – Owerri TS (8)
163.88
125
131.1
-
Afam PS when there was zero insolation at Gbarain PVGS, Alaoji-Owerri transmission lines.
electricity tariff because while deploying the system, cheaper electricity costs are likely going to be achieved.
-
-
Results from Cost Analysis
The LCoE calculation in chapter three was based on 2017 variables. The tax rate was based only on the VAT for gas supply. The calculated LCoE for the combined cycle gas turbines is 0.1027$/KWh, while LCoE for PV system is 0.08985$/KWh. This implies that the PV systems over its lifetime will provide cheaper electricity than the conventional sources.
4.0 CONCLUSION
The performance analysis of Nigeria electricity network was effectively carried out with the clear set objectives in focus throughout the study. Using PowerWorld simulator, the load flow analysis and contingency analysis of the modified network, that is the network with PV system integration, were studied. The results showed that PV systems whose source is in abundance in Nigeria can help us achieve power system adequacy and stability to reasonable extent.
This work is recommended for all power generation companies in Nigeria (Gencos). Once Nigeria power network is strengthened, the next challenge will be low generation
capacity. This will lead to more electricity supply challenges in Nigeria. Generation companies can use the PV systems as relief stations when there is good insolation and gas supply is low. They can also use PV systems integrated to the grid to control
REFERENCES
-
African-EU Renewable Energy Corperation Programme. (2016). Captive Power in Nigeria Comprehensive Guide to Project Development. Retrieved from https://www.ctc-n.org/sites/
-
Alex, M. (2015). Power Flow And Contingency Analysis: Case Of Rwanda High Voltage Power System.
-
Ezirim, G., Eke, O., & Onuoha, F. (2016). The Political Economy of Nigerias Power Sector Reforms: Challenges and Prospects, 2005- 2015. Mediterranean Journal of Social Sciences MCSER Publishing, 7(4), 443453. https://doi.org/10.5901/mjss.2016.v7n4p
-
Greacen, C., Engel, R., Quetchenbach, T., & Berkeley, L. (2013). A Guidebook on Grid Interconnection and Islanded Operation of Mini
– Grid Power Systems Up to 200 kW.
-
International Energy Agency. (2018). World Energy Outlook 2018 The gold standard of energy analysis. Retrieved from https://www.iea.org/weo2018/electricity/
-
Lazard. (2018). Levelized Cost of Energy and Levelized Cost of Storage 2018. Retrieved May 15, 2019, from https://www.lazard.com/perspective/levelized-cost-of-energy-and- levelized-cost-of-storage-2018/
-
PowerWorld Corporation. (2019). PowerWorld
-
The Visual Approach to Electrical Power Systems. Retrieved May 18, 2019, from https://www.powerworld.com/
-
Roche, M., Ude, N., & Donald-Ofoegbu, I. (2017). True Cost of Electricity: Comparison of Costs of Electricity Generation in Nigeria. Retrieved from https://ng.boell.org/sites/default/files/true_cost_of_power_technical
_report_final.pdf
-
Sambo, A. (2008). Matching electricity supply with demand in Nigeria. International Association for Energy Economics, 3236. Retrieved from
https://www.iaee.org/documents/newsletterarticles/408sambo.pdf
-
Samuel, I., Katende, J., Daramola, S. A., & Awelewa, A. (2014). Review of System Collapse Incidences on the 330-kV Nigerian National Grid. International Journal of Engineering Science Invention, 3(4), 5559. Retrieved from www.ijesi.org55%7C
-
Xoubi, N. (2015). Viability of a Utility-Scale Grid-Connected Photovoltaic Power Plant in the Middle East. Journal of Applied Sciences, 15(11), 12781287.
https://doi.org/10.3923/jas.2015.1278.1287