
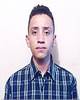
- Open Access
- Authors : Victor Manuel Perdomo Velazco , Sinddy Gabriela Colina Navas , Jose Rafael Ojeda Bracho
- Paper ID : IJERTV9IS120061
- Volume & Issue : Volume 09, Issue 12 (December 2020)
- Published (First Online): 19-12-2020
- ISSN (Online) : 2278-0181
- Publisher Name : IJERT
- License:
This work is licensed under a Creative Commons Attribution 4.0 International License
Performance and Comparative Analysis of Two Mechanical Draft Laboratory Scale Cooling Towers
Subjected to a Continue Open Recirculating Water Cooling System
VÃctor Manuel Perdomo Velazco* Sinddy Gabriela Colina Navas Petrochemical Engineering
UNEFA University
Punto Fijo City 4102, Venezuela
Jose Rafael Ojeda Bracho Energy Department UNEFM University
Punto Fijo City 4102, Venezuela
Abstract This research has been based on the performance and comparative analysis of two mechanical- draft counterflow cooling water towers. Their heat method was by direct contact and was subjected to continue recirculating water cooling system. The main objective of the present work was to determine how laboratory scale cooling towers work and what kind of mechanical-draft is the best for designing, by studying the effects of their structural components and air draft, focused on several factors as tower heights, time of contact between water and air, the filling, the cooling range, the approach to wet-bulb temperature, the effectiveness, the inlet water flow to be cooled and the heat load. The results were: Z = (F: 0,537m; I: 1,36m); R = (F: 12°C; I: 7°C); Ac = (F: 11°C; I: 22°C); = (F: 52%; I: 28%); L = (F: 11201, 13 Kg/h m3; I: 5972,77 Kg/h m3); Q (F: 8272 kW;
I: 6135,8 kW) and the filling used for both was a mesh made of high polyethylene. It was observed that both mechanical drafts are good for laboratory scale design. Parameters are acceptable for working, but water flow rate needs to be shorter than the entering air flow; the heating device must be outside of the tower because if it stays into the tower, it will increase the wet-bulb temperature; engineers/ designers should avoid mixing both draft.
Keywords Mechanical-draft cooling towers; forced-draft;
induced-draft; wet-bulb temperature; filling.
- INTRODUCTION
Cooling towers are devices that have as function, to carry out the conditions that a process demands (commonly used in power generation units, cold water refrigeration, air conditioning and industrial processes), and those conditions are established by the reduction of water temperature, which has passed through equipments to absorb and get heat out from them. Thus, the heat load gained, needs to be removed from that mass of water and could be done by exposing its surface to air of low humidity, to let it enter into the process again [1, 2]. The processes of cooling water are among one of the oldest known [3], and the cooling is achieved thanks to psychometric process, which involves three types of phenomena: interfacial mass transfer, latent heat transfer due to vaporization of a small portion of water and sensible heat transfer due to the difference in temperature of water and air [4, 5].
Currently, cooling towers are designed and manufactured in several types, and numerous sizes (models) available in each type Fig. 1. From critical literature review, cooling towers can be classified by the
Fig. 1 General Classification of cooling towers [6]
following characterization: first of all, they are characterized by method of heat. Depending on the contact between air and water, those devices are classified in direct contact (evaporative), whose primary cooling effect from the evaporation takes place when air and water are brought into direct contact, and the other one is by full utilization of dry surface coil segments, where the heat transfer which occurs between air and water is totally sensible, no direct (no evaporative). Evaporative cooling towers relate sensible and latent heat whereas in no evaporative cooling towers, the heat-transfer between the fluid to be cooled and air, it just sensible [6].
Cooling towers may be also characterized by its construction. They can be factory assembled or field erected. The first type does not usually exceeds 2250 m3/h of fluid capacity, whereas the second one, is up to 80000 m3/h of flow; cooling water circulating can fall vertically downwards and air can move vertically upwards through the filling (package or cell), showing a counterflow process, and air can flow horizontally across the downward fall of water, showing a crossflow process and characterizing devices by air flow.
Furthermore, classification can be by air draft, and they could be either natural draft (atmospheric) or mechanical draft, where the last characterization, is the most common applied in intermediate sizes of cooling towers [4]. Mechanical draft towers are categorized as either forced draft Fig. 2, on which the propeller or centrifugal blower type fan is mounted on the air stream inlet (on the base or bottom, between filling and collection basin) to force air through the tower, or induced draft Fig. 3, where its propeller type fan is located on the air discharges (on the top) to draw the air through the tower [5, 3].
Fig. 2 Forced-draft, counterflow cooling tower [5]
Fig. 3 Induced-draft, counterflow cooling tower [5]
Even though, there are two mechanical categorizations, induced-draft tower is the most common type, used in the United States, due to the fact that it avoids recirculation of wet air, and moreover, it produces air discharge velocity of from 3 to 4 times higher than its air entrance velocity, allowing a uniform distribution of air stream [3]. References [8] and [9] say that forced-draft tower is subjected to excessive recirculation of the humid exhaust vapors back into the air intakes, due to the low exit-air velocity Fig. 4, therefore, there is an increase in wet- bulb temperature of the exhaust air and consequently a decrease in tower performance.
Fig. 4 Recirculation of humid exhaust vapors [5]
Therefore the focus of this paper is to compare the performance of two mechanical-draft cooling towers, designed for a laboratory and subjected to a continue open water recirculation system, by studying the effects of the structural components, air draft and making emphases focused on the factors that affect those kind of devices that are not linked to tower heights and time of contact between water and air, but also with the filling and the following five parameters: cooling range, approach to wet-bulb temperature, effectiveness, inlet water flow to be cooled and heat load, all of this with the objective of demonstrating which mechanical configuration is the most proper for small sizes and what are the considerations to take when an engineer wants to design one [2, 3, 6, 7].
- COOLING TOWERS BASICS
- Structural components
Cooling tower structure must accommodate long duration dead loads imposed by the weight of the tower components, circulating water, snow and ice, etc. It must maintain its integrity throughout a variety of external atmospheric conditions, and despite a constant internal rainstorm. Wide-ranging temperatures must be accepted, as well as the corrosive effects of high humidity and constant oxygenation [6].
The components to be considered are the following:
- Cold water basin: The cooling tower basin is a chamber below and integrated with the tower where water is collected and directed to the sump or pump suction lineserve.
- Tower framework: The most commonly used materials for the framework of field-erected towers are pultruded fiberglass, wood, and concrete, with steel utilized
infrequently to conform to a local building code, or to satisfy a specific preference. Factory-assembled towers predominate in steel construction, with stainless steel increasingly utilized in locations (or for processes) that tend to promote corrosion.
- Water distributin system: In a general sense, piping and distribution of the water within the envelope of the tower are responsibilities of the tower manufacturer. Site piping, as well as attendant risers, valves and controls, which occur outside the confines of the cooling tower are provided and installed by others.
- Fan deck: The fan deck is considered a part of the tower structure, acting as a diaphragm for transmitting dead and live loads to the tower framing. It also provides a platform for the support of the fan cylinders, as well as an accessway to the mechanical equipment and water distribution systems. In other words, is the surface enclosing the top of an induced draft cooling tower, exclusive of the distribution basins on a crossflow tower.
- Fan cylinder: Is the cylindrical or venturi-shaped structure in which a propeller fan operates. Sometimes referred to as a fan stack on larger towers.
- Filling (Heat transfer surface): That portion of a cooling tower which constitutes its primary heat transfer surface. Sometimes referred to as packing.
- Heating device: A device used in an electrical circuit to provide resistance, in other words, is the device used for heating the water which is going to enter the column.
- Factors affecting cooling towers performance
- Wet-bulb temperature (Twb): It is the dynamic equilibrium temperature attained by a water surface when the rate of heat transfer to the surface by convection equals the rate of mass transfer away from the surface, in other words, is the temperature of the entering or ambient air adjacent to the cooling tower as measured with a wet-bulb thermometer [3, 7].
- Dry-bulb and/or relative humidity (Tdb): It is the temperature of the entering or ambient air adjacent to the cooling tower as measured with a dry-bulb thermometer [7].
- Heat load: Although appropriate selection of the cooling tower size establishes the equilibrium temperatures at which the tower will reject a given heat load, the actual heat load itself is determined by the process being served.
Everything else being equal, the size and cost of a cooling tower is proportional to the heat load. Therefore, it is of primary importance that a reasonably accurate heat load determination be made in all cases. If the heat load calculations are low, the cooling tower purchased will probably be too small. If the calculations are high, oversized, more costly equipment will result.
- Range (R) and approach (Ac): The range is the difference between the hot-water temperature and the cold water temperature. And the approach is the difference between the cold water temperature and either the atmospheric or the entering wet-bulb temperature.
- Interference: As previously indicated, local heat sources upwind of the cooling tower can elevate the wetbulb temperature of the air entering the tower, thereby affecting its performance. One such heat source might be a previously installed cooling tower on site, or in the immediate vecinity.
- Recirculation: It is an undesirable situation when wet-bulb temperatures are affected by some portion of the saturated air leaving the tower being induced back into the tower air inlets. Although wind is the primary cause of recirculation, the following other aspects of cooling tower design and orientation play an important part in its reduction and control:
- Tower shape.
- Orientation with prevailing wind.
- Air discharge velocity.
- Fan cylinder height and spacing.
- Tower sitting and orientation: It is the responsibility of the owner/ specifier to situate the tower such that these and other thermal performance influencing effects will be minimized. Since the long term capability of a cooling tower is determined by its proper placement on site, the importance of such placement cannot be overemphasized.
The performance of every cooling tower, large or small, depends upon the quantity and thermal quality of the entering air. External influences which raise the entering wet-bulb temperature, or restrict air flow to the tower, reduce its effective capacity. Air restrictions, recirculation and interferences can be minimized, possibly eliminated, by careful planning of tower placement using the following guidelines:
- Air restriction.
- Recirculation.
- Interference.
- Effect of site piping.
- Criteria for performance equations
Equation (1) is the cooling range (R), the difference between the hot-water temperature (TL2) and the cold-water temperature (TL1), in °C. At a fixed heat load, the increasing of the range decreases the circulating water flow. So, the tower needs a small surface of heat transfer, thus, a small tower height.
R = TL2 TL1 (1) Equation (2) is the approach to wet-bulb temperature (Ac), the difference between the cold water temperature (TL1) and the atmosphere wet-bulb temperature (Twb1), in
°C. It is the most important factor in the tower performance. A short approach indicates high effectiveness, but high costs and energy consumption also. Tower height is inversely proportional to the approach; if the approach is small, height is high, and vice-versa.
Ac = TL1 Twb1 (2) Equation (3) is the effectiveness (), in %. Understanding effectiveness such as thermal efficiency of the evaporation process, do not confuse with the mechanical efficiency of the tower, which is relative to the energy required to let air circulates through the tower.
(%) = [R / (R + Ac)]*100 (3) Equation (4) is the heat load to remove (Q), in kW. Where (mL) is the water mass flow rate, in kg/s. (CpL) is the water calorific value, in kJ/kg °C. And (TL) is the difference between hot-water minus cold-water temperature, in °C.
Q = mL * CpL *TL (4) - Structural components
- COOLING TOWERS CHARACTERISTICS
In the simultaneous mass and heat transfer analysis, the transfer direction and the scope of itself, are governed by the balance transfer condition. The conditions of a gas- vapor mixture are shown on a convenient concentration- temperature diagram, designed a constant pressure. On this diagram the gas exhaust concentration versus temperature is designed from details commonly obtained by experimental examination [4].
The air thermodynamic conditions such as dry-bulb temperature, wet-bulb (saturation) temperature, absolute humidity, percent relative humidity, and total mass flow of water per hour through the tower, are the starting point to define laboratory scale cooling tower parameters [11]. This is why it may be necessary to know where the laboratory environment conditions were designed the cooling towers.
Now, there are shown the forced-draft Fig. 5 and induced-draft Fig. 6 towers and their fundamental characteristics in the table I.
Fig. 5 Forced-draft cooling tower subjected to a continue recirculating water cooling system [12].
Fig. 6 Induced-draft cooling tower subjected to a continue recirculating water cooling system [13].
TABLE I. MECHANICAL-DRAFT COOLING TOWERS´ CHARACTERISTICS
Cooling Towers´ Characteristics Parameter Symbol Forced Draft Induced Draft Unit Hot (inlet) water temperature TL2 42 48 °C Cold (outlet) water temperature TL1 30 41 °C Dry-bulb air temperature Tdb1 p>21 21 °C Wet-bulb air temperature Twb1 19 19 °C Enthalpy of air stream HG1 59 —- kJ/ kg d.a. Inlet percent relative humidity Y1r% 87 —- % Ambient pressure P 100,977 71,98 kPa Air mass-flow rate Gs 5788,92 1194,55 Kg/h m2 Water mass-flow rate L 11201,13 662,63 Kg/h m2 Tower height Z 0,537 1,36 M Dry-bulb (outlet) air temperature Tdb2 37,7 44,5 °C Wet-bulb (outlet) air temperature Twb2 37 —- °C Enthalpy of air stream HG2 151,54 —- kJ/ kg d.a. Outlet percent relative humidity Y2r% 99 —- % In this case, the towers used a filling made of high density polyethylene. Forced-draft tower used a trickle pack filling 5mm*5mm, and induced-draft tower used a horizontal filling of 1mm*1mm, set with spaces between the top and the bottom of the columns, all of these defined by their height of unit transfer.
Cooling Towers´ Characteristics Parameter Symbol Forced Draft Induced Draft Unit Number of transfer unit NTU 3,14 5,01 Dimensionless Height of a unit transfer HTU 0,17 0,2 m Global mass- transfer coefficient Kya 16967,39 5972,77 Kg d.a./ h m3 Filling type —- High polyethylene High polyethylene —- Cooling Towers´ Characteristics Parameter Symbol Forced Draft Induced Draft Unit Number of transfer unit NTU 3,14 5,01 Dimensionless Height of a unit transfer HTU 0,17 0,2 m Global mass- transfer coefficient Kya 16967,39 5972,77 Kg d.a./ h m3 Filling type —- High polyethylene High polyethylene —- TABLE II. FILLING SECTION´CHARACTERISTICS
The range showed by forced-draft tower was 12 degrees of difference, knowing that hot water temperature was 42°C for a reduction of 30°C Fig. 7. Induced-draft tower range was seven points under inlet water temperature which was 48°C Fig. 8. Forced-draft tower got a bigger difference between inlet and outlet water temperatures, due to the fact that it worked with a short hot water flow and with a bigger air flow. Notwithstanding, the results gotten in temperature reduction were acceptable, because both kept an average of 16 degrees.
Fig. 7 Forced-draft cooling tower performance curve
Fig. 8 Induced-draft cooling tower performance curve
Forced-draft tower approach got a value of 11°C, closer than induced-draft one which was 22°C, Fig. 9. In actual practice, cooling towers are seldom designed for approaches closer than 2.8°C and the magnitude of approach to the wet-bulb temperature is dependent on tower design. So wide approaches (understanding as short difference between cold-water temperature and entering air wet-bulb temperature) suppose a large tower, and the inverse, short approach (understanding as big difference between cold-water temperature and entering air wet-bulb temperature) reduces tower height, therefore, approach is inversely proportional to tower height, (i.e. the approaches were good).
Fig. 9 Differences between mechanical-draft cooling towers
Mechanical-draft cooling towers effectiveness were: 52% for forced-draft, whereas 24% for induced-draft. Now, the values showed by each tower are the real performance conditions, but it should know that both are subjected to a continue open recirculation water cooling system and they do not obey to a demand of refrigerant fluid by another device, in other words, they were designed only for demonstrating cooling water process in unit operation processes laboratories.
Forced-draft effectiveness was higher than induced- draft one, due to the fact that the latter one put the resistor into the column (on the bottom). Even so, this one was designed for working with 5 propeller type fans, that helped out to maintain a uniform heat transfer through the column, notwithstanding, those fans where set 1 on the top and 4 on the sides, but those ones were put at the same height and with the same direction (without angle, no inclination). So as to, it got a mixture of air flows on the bottom, increasing wet-bulb temperature and reducing its effectiveness.
TABLE III. COOLING TOWERS PERFORMANCE CHARATERISTICS
Cooling Towers´ Characteristics Parameter Symbol Forced Draft Induced Draft Unit Range R 12 7 °C Approach Ac 11 22 °C Effectiveness 52 24 % Heat load Q 8272 6135,8 kW - CONCLUSION
In a brief, based on reference [14], the design of cooling towers is completely related to tower characteristics and different types of losses generated in cooling towers. Hence, both configurations are good for a laboratory scale design. Designer should select one of them, but they have to be clear that resistor must be outside of the column to avoid affecting entering air wet-bulb temperatures, and the variation in the water flow, air flow and others factors such as the colocation of the fan and the mixing of both drafts, can affect cooling effectiveness [15, 16].
REFERENCES
- Ç. Yunus, Heat and Mass Transfer. A Practical Approach. Mass Transfer, Mexico City: McGraw Hill, 2007, pp. 773-840.
- B. Bhavani, I. Swatchi, K. Prasanna and K. Srinivasa, Design of Cooling Tower, in IJSER, vol. IV, 2013, pp. 1560-1563.
- R. Perry, Perry´s Chemical Engineers´ Handbook 7th ed. United States of America: McGraw Hill, 1997.
- A. Foust, L. Wenzel, C. Clump, L. Maus and B. Andersen, Principles of Unit Operations, 2nd ed. Mexico City: CECSA, 2006.
- Instituto para la Diversificación y Ahorro de la EnergÃa de España, GuÃa técnica de torres de refrigeración, Madrid, 2007. Online http://Guia_Tecnica_de_Torres_refrigeracion.pdf
- SPX Cooling Technologies Staff (edited by John C. Hensley), Cooling Tower Fundamentals,2nd ed. 2009
- Gas Processors Suppliers Association, Egineering Data Book, 12th ed. Vol I & II. Tulsa City, USA. 2004.
- V. Del Olmo, Diseño y Simulación de Torres de Refrigeración Húmedas, 2013. Legánes, España. unpublished.
- R. E. Treybal, Operations of Mass Transfer, 2nd ed. Mc Graw Hill, 1967.
- C.J. Geankoplis, Unit Operation Process, 3rd ed. Mexico:CECSA, 1998.
- P. Martinez de la Cuesta and E. Rus, Separation Processes in Chemical Engineering, Madrid: PEARSON, 2004.
- S. Colina and V. Perdomo, Construction of a Cooling Tower Prototype, unpublished. Venezuela, 2018.
- V. Portero and S. Valarezo, Design and Construction of a Induced- Draft Cooling Tower, unpublished. Ecuador, 2012. Online http://dscape.espoch.edu.ec/bitstream/123456789/1972/1/96T00151. pdf
- Shashank Tiwari, Prof. Mahendra Agrawal, 2015, Analysis of Counter Flow Induced Draft Cooling Tower using Tagucgi Method, INTERNATIONAL JOURNAL OF ENGINEERING RESEARCH AND TECHNOLOGY (IJERT) Volume 04, Issue 06 (June 2015).
- Rohan Kapdi, Dr. Naveen Shrivastava, 2019, Design and Perfomance Analysis of Cross Floss and Froced Draft Cooling Towers, INTERNATIONAL JOURNAL OF ENGINEERING RESEARCH AND TECHNOLOGY (IJERT) Volume 08, Issue 07 (July 2019).
- CP Santhosh Ananda, N. Sekar, S. Senthil Kumar, S. Sheik Hyder,
S. Vignesh, 2017, Study of Cooling Towers and Comparision of Conventional Cooling Tower and Spray Cooling Pond. INTERNATIONAL JOURNAL OF ENGINEERING RESEARCH AND TECHNOLOGY (IJERT) ETDM -2017 (Volume 5, Issue 07).