
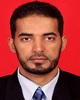
- Open Access
- Authors : Elsadic Salim , Amar Abobkr Amar
- Paper ID : IJERTV10IS030121
- Volume & Issue : Volume 10, Issue 03 (March 2021)
- Published (First Online): 26-03-2021
- ISSN (Online) : 2278-0181
- Publisher Name : IJERT
- License:
This work is licensed under a Creative Commons Attribution 4.0 International License
Potential of Renewable Energy Resources in Aljofra – Libya
Elsadic Salim1, Amar Abobkr Amar2
1 Department of Mechanical & Industrial Engineering, Faculty of EngineeringScience & Technology, Sebha University, Brack-Libya,
2 Higher institute of Sciences and Technology, Aljofra Sokna Libya,
Abstract:- Many scientific researchers have confirmed that solar energy can effectively replace the conventional energy that depend on burning fossil fuels in the sun belt regions. Libya in general, and the southern regions in particular such as Aljofra city, enjoys a high solar radiation intensity as high as 6.4 kWh/m2, and the annual average sunshine duration reaches 3550 hours. The annual average wind speeds were recorded high rates, with an average of 5 m/s, and this speed is suitable for electrical and mechanical applications of wind energy. The use of solar energy systems reduces air pollution, and it also reduces the overall load on the electricity-grid. To assess the technical and economic feasibility of using renewable energies in the selected region, System Advisor Model (SAM) software developed by National Renewable Energy Laboratory (NREL) is used in order to highlighting the potential of renewables in that region and to figure out the most economic choice for utility-scale electricity generation. Photovoltaic (PV) and Concentrated Solar Power (CSP) systems are the most promising technologies in this field. The different types of either CSP or PV have been experienced under hourly climatic data in order to identify the most economically system. The minimum levelized cost of energy (LCOE) was found to be 8 US/kWh that generated by solar trough technology in Aljofra site.
Keywords: Solar energy, wind energy, photovoltaic solar field, concentrated solar energy, Libya, Aljofra
-
INTRODUCTION
The world is heading at the present time to use environmentally friendly renewable energies to produce electrical, mechanical and thermal energy. Mechanical energy is used to pump ether water for agricultural and industrial use in remote areas. Thermal energy is used for symptoms of water desalination [1], cooling [2,3], and heating water and air for industrial applications as well as for domestic use [4, 5]. Thermal energy can also be used to generate electrical energy [6]. Electricity can also be generated directly by using photovoltaic solar panels [7,8]. Or it can provide both thermal and electricity by mains of PV/T systems [9-12].
Softwares are effective tools to inspect all variants under real operation and climatic conditions. Recently, many softwares are available to experience the performance of solar systems. Some of these softwares consider only photovoltaic solar systems such as: PVGIS, PVWatts. Other softwares consider different solar energy technologies including PV, CSP and wind energy, such as SAM (System Advisor Model). In this research, SAM software version (SAM 2017.1.17) developed by NREL is used, which available for free at https://sam.nrel.gov/.
It is a free software developed for predicting the performance of renewable energy systems and analyzing the financial feasibility of residential, commercial, and utility-scale grid-connected projects. SAM is unique in integrating a photovoltaic performance model with a detailed financial model for a given project. It has the ability to compare photovoltaic systems to other renewable energy systems. Moreover, it also offers additional analysis tools for optimization, parametric, and statistical analysis. Furthermore, SAM covers non-solar technologies such as geothermal power, wind power and biomass power [6].
The article is organized as follows: Section 2 gives a brief geographical description of Aljofra district. Section 3 describes the electrical power sector situation of the country. Section 4, presents the role of renewable energy in sustainable development in Libya. Section 5, illustrates the renewable energy sources in Libya, including the global horizontal solar radiation, wind speed and direction and the air temperature. Section 6 outlines the methodology followed in this research. the obtained results and the discussion are highlighted in section 6. In section 7 lists the conclusions drown from the research. the article finished with the bibliography, which includes 37 titles
-
SITE LOCATION AND INFORMATION
Aljofra District (29º 07 16 N, 15º 56 25 E) is an oasis town in the northern Fezzan region of southwest Libya. Figure 1 shows the geographical divisions of Libya and aerial view of Aljofra District.
Fig. 1: The administrative divisions of Libya and aerial view of the targeted City.
Source: (https://zoom.earth/#view=29.12394,15.949424,15z/layers=nolabels).
-
THE POWER SECTOR SITUATION
The power system of Libya is almost 100% provided by fossil fuels. The electricity sector relies on 20 conventional generating stations, most of which are located on the coast in the northern region, table 1 updated the status and the capacity of these electrical power stations. The total installed capacity amounted to about 8,051 MW, with the total electrical energy produced in 2012 reaching about 33,980 MWh, Aljofra district consumed 5% of this amount [13]. The steam stations generated 20%, while the share of the gas stations reached 43%, and the rest was by using the joint stations technology. Generation was dependent on domestically produced fossil fuels; The use of heavy fuel in generation reached 20%, and light fuel 40%, and the contribution of natural gas was 40% of the total generation [14]. Figure 2 shows the distribution of the percentages of technologies and the type of fuel used in the electric power plants [15].
Furthermore, the electric power production sector in Libya is considered as the largest sources of pollution in the country [16-19], due to its complete dependence on fossil fuels (heavy oil, light oil, and natural gas) in generating electric power. This is followed by the transportation sector. Figure 3 represents the percentages of carbon dioxide emissions from some sectors in Libya [20].
Table 1: Status of electrical power plants in Libya as of January 2021 [21].
Power plant
Phases
Generation Units
Rated Capacity per Unit (MW)
Date of Operation and Status
Current Output (MW)
Al Khums
Phase 1
4
Steam
127
1982
400
Phase 2
4
Gas
150
1995
560
Phase 3
2
Gas
275
2017–out of service
0
North Benghazi
Phase 1
4
Steam
40
1979-out of service
0
Phase 2
3
Gas
150
1995
240
Phase 3
1
Gas
165
2002
75
2
CC
150
2007
0
Phase 4
2
Gas
285
2009
500
1
CC
250
2012 0
Al Zawiya
Phase 1
4
Gas
165
2000
575
Phase 2
2
Gas
165
2005
270
Phase 3
3
CC
150
2007
185
Phase 4
2
Gas
25
2014
45
Misrata CC
Phase 1
2
Gas
285
2010
530
Phase 2
1
CC
250
2013
225
South Tripoli
Phase 1
5
Gas
100
1994
330
Phase 2
2
Gas
47
2016
0
Sarir
Phase 1
2
Gas
285
2010
400
Phase 2
1
Gas
285
2013
Zwitina CC
Phase 1
4
Gas
50
1994
90
Phase 2
2
Gas
285
2010
500
1
CC
250
Suspended
0
Power plant
Phases
Generation Units
Rated Capacity per Unit (MW)
Date of Operation and Status
Current Output (MW)
Al Jabal Algharbi- Ruwais
Phase 1
2
Gas
156
2005
785
Phase 2
2
Gas
156
2006
Phase 3
1
Gas
156
2010
Phase 4
1
Gas
156
2012
West Tripoli
Phase 1
5
Steam
65
1976-out of service
0
Phase 2
2
Steam
120
1980- out of service
0
Phase 3
4
Steam
350
Contracted
0
Phase 4
3
Gas
25
2014
60
Darna
Phase 1
5
Steam
65
1985
0
Tubruk
Phase 1
5
Steam
65
1985
0
Alkhalij-Sert
Phase 1
4
Steam
350
2014 (1 unit in operation)
280
Alzahra
Phase 1
2
Gas
15
1971
0
Phase 2
4
Gas
47
Under Construction
0
Ubari
Phase 1
4
Gas
165
2019
240
Abu Kammash
Phase 1
6
Gas
15
1982
0
Zliten
Phase 1
3
Gas
15
1975
0
Alfurnaj
Phase 1
2
Gas
15
1971
10
Lamluda
Phase 1
1
Gas
33
1975
0
Alkufrah
Phase 1
3
Gas
25
1975
0
Misrata Kerzaz
Phase 1
3
Gas
15
1984
20
(ii)
(ii)
(i) (iii)
Fig.2: Breakdown of (i) energy consumption by sectors, (ii) electricity generation by fuel consumed, and (iii) generation technology.
Fig.3: The annual CO2 (ton/year) emitted by sectors and the share of each sector in total CO2 emission
-
THE ROLE OF RENEWABLE ENERGY IN SUSTAINABLE DEVELOPMENT IN LIBYA
There is a relationship between the environment, energy, and development, and this relationship is one of the most important findings that we have reached in the study of our current research. This relationship clarifies the concept of sustainable development, and the extent of its interest in protecting energy resources, and rationalizing their use, taking into account the environmental aspect. One of the most important and prominent steps towards the harmonization between economic growth and
sustainable development approaches and their contributions to reducing greenhouse gas emissions and climate change, and to what extent are the adequacy of the measures in presenting the concept of energy policy governance aimed at moving to the environmental economy by integrating the traditional energy sectors within the pattern. Modern economic and clean development system. Obtaining information enables us to understand the relationship between studying the environmental impact of many projects and protecting natural resources to preserve the share of future generations, achieving social justice, and acquiring skills to analyze the relationship between environment, energy, development, and the essential role that the fossil fuel sector plays in The development of financial returns, which are the basis of development policies in Libya, and on which renewable energy can be included in the energy policy in Libya.
-
RENEWABLE ENERGY SOURCES IN LIBYA
Renewable energy has advantages over conventional fossil fuel sources to save energy and its resources are always renewable, such as solar radiation and wind. It is more economically effective in many applied fields, environmentally friendly, and energy sustainable supply [22]. Libya can generate electricity all demands by mains of hybrid system (solar energy and wind energy).
-
Climate data
In the absence of weather stations and the absence of reliable weather measurements, the information banks available in the International Information Network were used. There are many free-web services for weather information, but in this research the Solar Radiation Database (SoDa) was chosen [www.soda-pro.com]. The web service SoDa is a database for Europe and North Africa countries that is reliable and is recommended by many researchers [23].
-
Global solar radiation (GHI)
Figure 4 shows the average the time series hourly global horizontal solar radiation data over three years (2004-2006) for the site under concern. These data are obtained from SoDa.
Fig.4: Hourly global solar radiation on Aljofra, W/m2
-
Wind speed and direction (WS, WD)
The hourly wind speed and direction for the site under concern are shown in Figure 5. They are obtained from SoDa. The wind rose is obtained via the free program WRPLOT 7.0.0 [https://weblakes.com/products/wrplot/index.html]. The wind-rose is a graphic tool used to give information about the wind speed frequency and the dominate direction at a certain site [24].
Fig.5: Hourly wind speed and wind-rose
Figure 5 shows that average wind speed in the site under concern is 5 m/s. This is sufficient for producing electricity. The dominant direction of the wind is determined from the wind rose. This is to direct the blade of the wind farm [24].
-
Temperature
-
The temperature is the most important parameter in the climatic data. The temperature has direct impact on the characteristics of the load and PV modules [7]. Figure 6 presents hourly temperature profile for Aljofra District.
Fig.6: Hourly air temperature
Figure 6 shows that the temperature at Aljofra district varies from less to 5ºC to more than 40ºC; the average temperature is around 22ºC. The sizing procedure has to considered the slight reduction in the output of PV modules due to temperature increase above Standard Test Conditions (STC) [8].
-
-
METHODOLOGY
There is an imperative that must be taken into account when choosing the database platform and the software that will use to estimate the potential of renewables, which is to verify that the solar irradiance transposition model used is the same as the recommended model for the area under study. Nassar et. al. provided lists of the transposition models that used by numerous of softwares and databases [25]. In the present study, many models have been tested, and studies have shown that Liu and Jordan's model showed acceptable accuracy in representing the incident solar irradiation on an inclined surface to the southern regions of Libya [26- 28]. The second thing is to make sure the solar collector tilted to the optimum angle to avoid energy losses [29,30] and shadow specially in case of PV solar fields [31-36]. However, some of softwares suggested an optimum tilt angle as well.
Although most of the solar energy softwares calculate the inclined global solar irradiance. Many solar energy textbooks are documented several approaches to calculate the global solar radiation incident on an inclined surface [37- 39]. Fig. 7 demonstrates the methodology adopted by the present research.
In order to identify the feasibility of utility scale solar-electrical technique, the research compares between values of LCOE produced by SAM software. SAM calculates LCOE based on hourly climatic data. The update economic data are also collected from the websites and tabulated in Table 2.
Fig. 7: The flowchart of the methodology
Table 2: Key economic cost of utility scale of renewable energy technologies [6]
PV (standard module) utility scale |
Capital cost ($/kW) |
Fixed O&M ($/kW-y) |
Irradiation at optimal angle |
Photovoltaic – Fixed |
1,450-2,850 |
24.69 |
3030kWh/m2/year |
Photovoltaic – single tracking |
2,300-3,300 |
23.4 |
3030 kWh/m2/year |
Photovoltaic – Dual tracking |
2,350-3,350 |
23.9 |
3030 kWh/m2/year |
CPS technologies |
Direct normal irradiation (DNI) |
||
CPV |
2,100-3300 |
50 |
2560 kWh/m2/year |
Parabolic trough storage of 8h |
2,700-4,000 |
50 |
2560 kWh/m2/year |
Solar tower with storage of 8h |
2,500-4,400 |
50 |
2560 kWh/m2/year |
Fresnel |
2,500-5,500 |
50 |
2560 kWh/m2/year |
Dish |
1,300-12,600 |
50 |
2560 kWh/m2/year |
Wind energy |
Minimum wind speed |
||
Wind farm |
1,300-2,300 |
60 |
5 m/s [24] |
6. RESULTS AND DISCUSSIONS
Although SAM has many useful outputs, we have previously chosen LCOE as an optimizing parameter for figure out the most economically renewable – electricity generation technology. Figure 8 demonstrates the LCOE for the seven renewable technologies considered in the present article.
Fig. 8: The LCOE of several types of utility-scale solar energy according to the location
Figure 8 clearly shows, with minimum LCOE cost, the competition is decided in favour of trough solar energy technology. In fact, it was expected to reach such a result for several reasons:
-
The study area falls within the "solar belt region", and this area is preferred to use thermal applications of solar energy due to the high rates of DNI in addition to the high air temperature, which negatively affects the performance of the PV solar technology.
-
Selection of the giant European energy project Desertec project energy from desert; the trough technology to generate electrical energy from this region in advance [17].
-
These results coincide with the results of the research for Saudi Arabia, which climate completely matches the climate of the studied area [6].
7. FUTURE INVESTIGATIONS
In fact, there is a lot of work to reach the best option for electrical generation from renewable energies. The best option may be a hybrid of several technologies as it depicted in Figure 9. Thus, the future plan for research in this area includes:
-
Gathering climatic information for several regions in Libya.
-
Conducting field experiments and measurements.
-
Choosing the best technology in the populated areas, especially the southern regions.
-
Use solar energy software such as HOMER (Hybrid Optimization of Multiple Energy Resources) to obtain the optimum size for each component of the hybrid system.
Fig. 9: Solar PV-wind-diesel hybrid system. source [40]
7. CONCLUSIONS
The current study showed that the use of solar energy technologies to generate electric energy can be a solution to the problem of the electricity crisis in the Libyan state, which it has been suffering from since 2011. Building small stations around urban zones, especially in the southern region, which is very far from places of electricity generation, will generally increase the efficiency of generation and distribution of electricity due to the large losses in the process of transmitting electricity through thousands of kilometres from the coast to the depth of the Sahara Desert. The decentralized hybrid generation can be a viable solution and constitute economic wealth in addition to the crude oil, especially with the presence of the public electricity grid that connects all the country to each other and to neighbouring countries as well.
BIBLIOGRAPHY
[1]. Nassar, Y., Yousif, S., Salem, A., 2007. The second generation of solar desalination systems, Desalination 209, 177181. https://doi.org/10.1016/j.desal.2007.04.039. [2]. Nassar, Y., Salem, A., 2002. Solar energy & energy conservation in buildings, 1st International Congress of Mechanics-Constantine Faculty of Engineering Department of Mechanical Engineering, University of Constantine, Algeria 14-16 December 2002. [3]. Nassar, Y., ElNoaman, A., Abutaima, A., Yousif, S., Salem, A., 2006. Evaluation of the underground soil thermal storage propertes in Libya, Renewable energy 31:5, pp. 593-598. https://doi.org/10.1016/j.renene.2005.08.001 [4]. Amer, K., Fakher, M., Ahmad, S., Irhouma, M., Altahbao, S., Salem, E., 2020. Performance of domestic solar heating system with thermal storage using phase change materials. International Journal of Engineering Research and Development, vol. 16(9), 2020, pp 01-11. http://www.ijerd.com/current%20issue.html [5]. Nassar, Y., 2015. Thermodynamics analysis and optimization procedure for domestic solar water heating system, AASCIT, American Journal of Energy and Power Engineering, 2(6), 92-99. http://ww.aascit.org/journal/ajepe [6]. Hafez, A., Nassar, Y., Hammdan, M., Alsadi, S., 2019. Technical and Economic Feasibility of Utility-Scale Solar Energy Conversion Systems in Saudi Arabia, Iranian Journal of Science and Technology, Transactions of Electrical Engineering, Transactions of Electrical Engineering https://doi.org/10.1007/s40998-019-00233-3 [7]. Salem, A., Nassar, Y., Yousif, S., 2004. The Choice of Solar Energy in the Field of Electrical Generation-Photovoltaic or Solar Thermal – For Arabic Region, World Renewable Energy Congress VIII (WREC 2004). [8]. Nassar, Y., Salem, A., 2007. The reliability of the photovoltaic utilization in southern cities of Libya, Desalination 209, 8690. https://doi.org/10.1016/j.desal.2007.04.013. [9]. Nassar, Y., Salem, A., Sergievsky, E., 2001. A creation of mathematical model for photovoltaic/thermal (PV/T) solar flat-plate collector, International conference on renewable energy for regional development CIGA, Bogor Indonesia, 2831. http://www.witpress.com. [10]. Fathi, N.Y., Alamen, S. M., 2014. Economic and Energetic analysis for optimizing the length of flat-plate solar air heating collectors, Applied Mechanics and Materials, vols. 446-447, pp 810-816. https://doi.org/10.4028/www.scientific.net/AMM.446-447.810 [11]. Nassar, Y., Alsadi, S., Amer, K., Yousef, A., Fakher, M., 2019. Numerical Analysis and Optimization of Area Contribution of The PV Cells in the PV/T Flat-Plate Solar Air Heating Collector, Solar Energy Research Update 6, 43-50. http://www.zealpress.com/jseruv6a5 [12]. Nassar, Y., Sergievsky, D., 2000. Heat transfer in flat-plate solar air heating collector, Heat Transfer 2000 "the sixth international conference on advanced computational methods in heat transfer", held from 26-28 June 2000 in Madrid, Spain, pp. 575-584. http://www.witpress.com [13]. Task A: sector performance and structural sector reform – deliverable five: final report (English). Washington, D.C.: World Bank Group. 2020. http://documents.worldbank.org/curated/en/616071527050024484/ [14]. S. Yousif, A. Salem, Y. Nassar, I. Bader, 2006. Investigation of pollutants dispersion from power stations, International journal of energy research 30:15, 1352-1362. [15]. Amer, K., Fakher, M., Ahmad, S., Irhouma, M., Altahbao, S., Salem, E., 2020. Performance of domestic solar heating system with thermal storage using phase change materials. International Journal of Engineering Research and Development, vol. 16(9), pp 01-11. http://www.ijerd.com/current%20issue.html [16]. Y. Nassar, K. Aissa, S. Alsadi, 2017. Air Pollution Sources in Libya. Research & Reviews: Journal of Ecology and Environmental Sciences, 5, 63-79. [17]. Y. Nassar, K. Amer, M. Irhouma, S. Ahmad, 2015. Economical and environmental assessment of electrical generators: A case study of southern regionof Libya, International Journal of Energy Policy and Management 1(4): 64-71
[18]. Y. Nassar, S. Alsadi, 2016. Economical and environmental feasibility of the renewable energy as a sustainable solution for the electricity crisis in the Gaza Strip, International Journal of Engineering Research and Development, Volume 12, Issue 3, PP.35-44 [19]. Nassar, Y.F., Salem, M.A., Iessa, K.R. et al., 2021. Estimation of CO2 emission factor for the energy industry sector in libya: a case study. Environ Dev Sustain. https://doi.org/10.1007/s10668-021-01248-9 [20]. Y. Nassar, K. Aissa, S. Alsadi, 2017. Estimation of Environmental Damage Costs from CO2e Emissions in Libya and the Revenue from Carbon Tax Implementation. Low Carbon Economy, 8, 118-132. [21]. Almaktar, Mohamed et al. 2021. Revitalizing operational reliability of the electrical energy system in Libya: Feasibility analysis of solar generation in local communities. Journal of cleaner production vol. 279: 123647. https://doi:10.1016/j.jclepro.2020.123647 [22]. Nassar, Y., Alsadi, S., 2019. Assessment of solar energy potential in Gaza Strip-Palestine, Sustainable Energy Technologies and Assessments 31, 318- 328. https://doi.org/10.1016/j.seta.2018.12.010 [23]. Yasser F. Nassar, Mohammad J. Abdunnabi, Mohamed N. Sbeta, Ahmad A. Hafez, Khaled A. Amer, Abdulaziz Y. Ahmed, Basim Belgasim, 2021. Dynamic analysis and sizing optimization of a pumped hydroelectric storage-integrated hybrid PV/Wind system: A case study, Energy Conversion and Management 229. https://doi.org/10.1016/j.enconman.2020.113744 [24]. Nassar, Y., Alsadi, S., 2018. Wind energy potential in Gaza Strip-Palestine state, Solar Energy and Sustainable Development, Volume (7), No (2), pp.41-57, http://www.jsesd.csers.ly/images/pdf/vol-007-02/vol-007-02-04.pdf
[25]. Nassar, Y., Hafez, A., Alsadi, S., 2020. Multi-factorial comparison for 24 distinct transposition models for inclined surface solar irradiance computation in the state of Palestine: a case study. Front. Energy Res. 7:163. https://doi.org/10.3389/fenrg.2019.00163 [26]. Alsadi, S., Nassar, Y., 2017. Estimation of solar irradiance on solar fields: an analytical approach and experimental results. IEEE 16011608. https://doi.org/10.1109/TSTE.2017.2697913 [27]. Nassar, Y., Alsadi, S., 2016. View factors of flat solar collectors array in flat, inclined, and step-like solar fields. J. Solar Energy Eng. ASME Trans.138, 18. https://doi.org/10.1115/1.4034549
[28]. Nassar, Y. F., 2020. Analytical-numerical computation of view factor for several arrangements of two rectangular surfaces with non-common edge,International Journal of Heat and Mass Transfer 159. https://doi.org/10.1016/j.ijheatmasstransfer.2020.120130
[29]. Alsadi, S., Nassar, Y., 2016. General polynomial for optimizing the tilt angle of flat solar energy harvesters based on ASHRAE clear-sky model in mid and high latitudes, Energy and Power, Vol. 6, No. 2, http://www.sapub.org/journal/currentissue.aspx?journalid=1018 [30]. Alsadi, S., Nassar, Y., 2017. Energy demand based procedure for tilt angle optimization of solar collectors in developing countries, Journal of Fundamentals of Renewable Energy and Applications,7 : 225. https://doi.org/10.4172/2090-4541.1000225 [31]. Alsadi, S. Y., Nassar Y. F., 2017. A Numerical Simulation of a Stationary Solar Field Augmented by Plane Reflectors: Optimum Design Parameters,Smart Grid and Renewable Energy 8(7), 221-239. http://www.scirp.org/journal/sgre/
[32]. Nassar, Y., Hadi, H., Salem, A., 2008. Time Tracking of the Shadow in the Solar Fields, J. Sebha Univ., 7(2), pp. 5973. [33]. Appelbaum, J., Bany, J., 1979. Shadow effect of adjacent solar collectors in large scale systems. Sol. Energy 23, 497507. [34]. Alsadi, S., Nassar, Y., 2019. A general expression for the shadow geometry for fixed mode horizontal, step-like structure and inclined solar fields, Solar Energy 181, 5369. https://doi.org/10.1016/j.solener.2019.01.090 [35]. Amer, K., Fakher, M., Salem, A., Ahmad, S., Irhouma, M., Altahbao, S., Salim, E., 2020. Power Losses on PV solar fields: Sensitivity analysis and a critical review, International Journal of Engineering Research & Technology (IJERT), vol.9 no. 9, pp. 1000-1007, http://www.ijert.org/research/IJERTV9IS090439 [36]. Suliman M. Ahmad, Mukhtar A. Irhouma, Khaled A. Amer, Massuod A. Fakher, Abdallah Salem, Salah Aldeen S. Altahbao, Elsadic Salim, 2021. Instantaneous Shadow Estimation in Stationary-Horizontal PV Solar Fields, Peer Rev J Sol Photoe Sys. 1(4), 1-8. https://crimsonpublishers.com/prsp/pdf/PRSP.000520.pdf [37]. Nassar, Y. Solar energy engineering active applications, Sabha University, Libya, 2006. [38]. Duffie, J.A. and Beckman, W.A. (2013) Solar Engineering of Thermal Processes. 4th Edition, Wiley, New York. https://doi.org/10.1002/9781118671603 [39]. Alsadi, S.Y. and Nassar Y.F., 2016. Correction of the ASHRAE Clear-Sky Model Parameters Based on Solar Radiation Measurements in the Arabic Countries. International Journal of Renewable Energy Technology Research, 5, 1-16. [40]. Sumon R., S. Rana, S.K.A. Shezan, S. K., Shamim A., 2016. Optimized Design of a Hybrid PV-Wind-Diesel Energy System for Sustainable Development at Coastal Areas in Bangladesh, Environmental Progress & Sustainable Energy 36(1), 1-8. https://doi.org/10.1002/ep.12496