
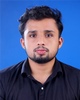
- Open Access
- Authors : Waseem Abdul Wahab, Mrs Saritha Sasindran
- Paper ID : IJERTV13IS060141
- Volume & Issue : Volume 13, Issue 06 (June 2024)
- Published (First Online): 03-07-2024
- ISSN (Online) : 2278-0181
- Publisher Name : IJERT
- License:
This work is licensed under a Creative Commons Attribution 4.0 International License
Seismic Mitigation and Vibration Control of Multi Storey RCC Building with “Aqua- Damp” Concept
Waseem Abdul Wahab
Post Graduate Student Department of Civil Engineering
Sree Narayana Guru College of Engineering & Technology Payyannur, Kannur, Kerala, India
Mrs Saritha Sasindran
Assistant Professor Department of Civil Engineering
Sree Narayana Guru College of Engineering & Technology Payyannur, Kannur, Kerala, India
Abstract- Aqua damper is a type of tuned mass damper (TMD) where the mass is supplement by a liquid (usually water). It is also referred to as tuned liquid dampers (TLD) and is a passive damping device which utilizes the motion of liquid in a container for dissipating the vibration energy from seismic activity. As the sloshing frequency of the water is tuned to the natural frequency of the structure, resonance will occur and this will cause large amount of sloshing and wave breaking hence dissipating a significant
amount of energy. The natural frequency is controlled by adjusting water depth and tank dimensions. Implementing aqua dampers enhances the performance of the structures. The main aim of this project is to study the efficiency of aqua dampers when employed as seismic mitigation measures in a RCC multi storey building.
Keywords:- Tuned Liquid Damper (TLD), Aqua Damp concept, sloshing frequency
-
INTRODUCTION
The evolving landscape of urban architecture continually challenges engineers and researchers to develop innovative solutions to enhance the resilience and safety of multistory RCC buildings against seismic activities and structural vibrations. Earthquakes pose a significant threat to multi- storey buildings, particularly in regions with high seismic activity. The dynamic forces generated during an earthquake can lead to severe structural damage or even catastrophic failure, making seismic mitigation a critical concern in civil engineering. Reinforced Cement Concrete (RCC) buildings, widely used for their durability and load-bearing capacity, are no exception to these challenges. However, their rigid nature can result in substantial inertial forces during seismic events, necessitating effective strategies to enhance their resilience. Traditionally, seismic mitigation in buildings has relied on methods such as base isolation, which decouples the building from ground motion, and the use of various forms of damping systems that absorb and dissipate energy. While these methods have proven effective, they often involve significant alterations to the building's structure and can be costly or impractical, especially for retrofitting existing buildings.
Fig. 1 Working of aqua damper
The Aqua Damping concept in seismic mitigation refers to the use of water-based damping systems, particularly Tuned Liquid Dampers (TLDs), to reduce seismic vibrations in structures such as multi-storey Reinforced Cement Concrete (RCC) buildings. This method leverages the dynamic properties of liquid to counteract the forces induced by seismic activity, thereby enhancing the stability and integrity of the building during an earthquake. Aqua damping operates on the fundamental principles of fluid dynamics and resonance tuning. The core idea is to use a container filled with liquid, usually water, whose movement (sloshing) can be harnessed to generate counteracting forces against the building's motion. This sloshing action
provides a passive and adaptable method of damping that can significantly reduce the amplitude of vibrations. The natural frequency of the liquid sloshing within the tank is tuned to match the buildings primary vibrational frequency. When the building oscillates due to seismic forces, the liquid inside the damper also oscillates at the tuned frequency, creating a phase difference that counteracts the building's motion. As the liquid sloshes, it converts kinetic energy into potential energy and then dissipates it through friction and turbulence within the tank. This process reduces the overall energy of the system, dampening the vibrations and minimizing the buildings sway.
-
OBJECTIVES
-
Implementation of water tank as tuned liquid damper on a multi storey RCC building (G+12) using ETABS SOFTWARE.
-
To study the effect of TLD on regular structure in different conditions by varying water depth, tuning ratio and location of tank.
-
To analyze structures in terms of different parameters like storey displacement, storey drift, storey acceleration, base shear and time period.
-
-
SCOPE OF THE WORK
The scope of this study is to enhance the performance of RCC multi storey structure implementing aqua damper concept.
-
PARAMETRIC STUDY
Multiple aspects were taken into consideration when conducting the investigation on the effect of implementing aqua damp concept in a multi storey RCC building. the horizontal ground motion records of the El Centro have been selected for performing the nonlinear dynamic Time History analysis. The Characteristics of the selected earthquake motion in terms of peak ground acceleration (PGA), moment magnitude (M) are 0.34g and 7.2 respectively. The effect of TLD on regular structure in different conditions by varying water depth, tuning ratio and location of tank are studied. Seismic parameters such as
base shear, storey displacement and top storey acceleration are studied and compared with conventional structure.
Code of references: Indian Standard codes IS 1893 (Part 2) (2014)
-
SUMMARY OF LITERATURE REVIEW
Various literatures have been reviewed including the base journal Use of Water Tank as Tuned Liquid Damper (TLD) for Reinforced Concrete (RC) Structures Muhammad Jamil Ahmad, Qaiser uz Zaman Khan, Syed Muhammad Ali (2021), SPRINGER
Installing water tanks in buildings in accordance with the Aqua- damping concept will help create an inbuilt and cost effective system for the structure to mitigate seismic shocks to the building. New researches in this field promise the development of more efficient and cost effective seismic mitigation techniques for improved efficiency of the buildings.
-
MODELLING
Thirteen storey reinforced concrete moment-resisting frame building is considered. The considered building has a width of 16 m divided into 4 bays and length of 36 m divided into six bays as well. The associated storey height considered is of 3 m. The designed reinforced concrete beams have been set to be of 300 mm × 600 mm. The designed reinforced concrete columns have been set to be of cross sections 300 mm × 900 mm. The dimension of water tank has been set as 12 m × 8 m.
The water tank has been designed as a spring mass model for analysis in ETABS. The calculations for parameters like convective mass, impulsive mass, height of convective mass, height of impulsive mass and stiffness of spring was done in accordance to data from IS 1893 (part 2).
Modelling was done for three conditions:
-
Single tank condition
-
Two tanks condition
-
Three tanks condition
Fig. 2 Spring mass model for water tank from IS 1893 (Part 2)
Fig. 3 Graph data No.1 from IS 1893 (Part 2)
Fig. 4 Graph data No.2 from IS 1893 (Part 2)
Fig. 5 Plan of building model in Etabs
Fig. 6 Beam details of model in Etas
Fig. 7 Water tank model for analysis
Fig. 8 Building elevation model with 1 tank
Fig. 9 Building elevation model with 2 tank
Fig. 10 Building elevation model with 3 tanks
-
-
TEST RESULT AND DISCUSSION
The results obtained from analysis of single tank, two tank and three tank conditions with 20%, 40%, 60% and 80% capacity of water tank filled for each condition has been discussed. The values have been tabulated and trends of parameters in different conditions have been plotted in graphs using values obtained.
Table 1 Tabulated values of parameters considered
The values of parameters have been compared and comparison in changes in values of parameters has been tabulated in terms of percentage.
Table 2 Comparison of parameter values for Displacement and Base shear
Table 3 Comparison of parameter values
14
12
10
8
6
4
2
0
NO TANK
20%
40%
60%
80%
0 50 100 150 200
DISPLACEMENT
STOREY
Drift, time period and acceleration
15
10
5
0
0
0.005
DRIFT
0.01
NO TANK
20%
40%
60%
80%
STOREY
Table 4 – Displacement and Drift values for different stories from Etabs analysis with no water tank
15
10
5
0
0
0.005
DRIFT
0.01
NO TANK
20%
40%
60%
80%
STOREY
Fig. 11 Storey drift in x-axis 1 tank condition
Fig. 12 Storey drift in y-axis 1 tank condition
Fig. 13 Storey displacement in x-axis 1 tank condition
14
12
10
8
6
4
2
0
NO TANK
20%
40%
60%
80%
0
100
DISPLACEMENT
200
STOREY
Fig. 14 Storey displacement in y-axis 1 tank condition
15
10
5
0
0
0.002 0.004 0.006
DRIFT
NO TANK
20%
40%
60%
80%
14
12
10
8
6
4
2
0
NO TANK
20%
40%
60%
0
100
DISPLACEMENT
200
80%
STOREY
STOREY
For 2 tank condtiton,
15
10
5
0
NO TANK
20%
40%
60%
0
0.005
DRIFT
0.01
80%
STOREY
Fig. 15 Storey drift in x-axis 2 tank condition
14
12
10
8
6
4
2
0
NO TANK
20%
40%
60%
0
100
DISPLACEMENT
200
80%
STOREY
Fig. 16 Storey drift in x-axis 2 tank condition
STOREY
Fig. 17 Storey displacement in x-axis 2 tank condition
Fig.18 Storey displacement in y-axis 2 tank condition
15
10
5
NO TANK
20%
40%
60%
0
0
0.002 0.004 0.006
DRIFT
80%
STOREY
Fig. 19 Storey drift in x-axis 3 tank condition
14
12
10
8
6
4
2
0
NO TANK
20%
40%
60%
80%
0
0.005
DRIFT
0.01
Fig. 20 Storey drift in y-axis 3 tank condition
14
12
10
8
6
4
2
0
NO TANK
20%
40%
60%
80%
0
100
DISPLACEMENT
200
160
140
120
100
SINGLE TANK
TWO TANKS
80
THREE TANKS
20%
70%
120%
PERCENTAGE OF WATER FILLED INSIDE TANK
STOREY
DISPLACEMENT
Fig. 24 Displacement trends along y-axis
14000
13000
12000
11000
10000
9000
8000
7000
6000
SINGLE TANK
TWO TANKS THREE TANKS
20% 70% 120%
PERCENTAGE OF WATER FILLED INSIDE TANK
BASE SHEAR
Fig. 21 Storey displacement in x-axis 3 tank condition
14
12
10
8
6
4
2
0
NO TANK
20%
40%
60%
80%
0
100
DISPLACEMENT
200
STOREY
Fig. 25 Base shear trends along x-axis
16000
15000
14000
13000
12000
11000
10000
9000
8000
7000
6000
SINGLE TANK
TWO TANKS THREE TANKS
20% 70% 120%
PERCENTAGE OF WATER FILLED INSIDE TANK
BASE SHEAR
Fig. 22 Storey displacement in y-axis 3 tank condition
160
140
120
SINGLE TANK
100
TWO TANKS
80
THREE TANKS
20%
70%
120%
PERCENTAGE OF WATER FILLED INSIDE TANK
DISPLACEMENT
Fig. 26 Base shear trends along y-axis
Fig. 23 Displacement trends along x-axis
0.006
0.0055
0.005
0.0045
0.004
SINGLE TANK
TWO TANKS
0.0035
THREE TANKS
0.003
20% 70% 120%
PERCENTAGE OF WATER FILLED INSIDE TANK
2.4
2.2
2
1.8
1.6
1.4
1.2
SINGLE TANK
TWO TANKS THREE TANKS
20% 70% 120%
PERCENTAGE OF WATER FILLED INSIDE TANK
DRIFT
DRIFT
TIME PERIOD
Fig. 27 Drift trends along x-axis
2.4
2.2
2
1.8
1.6
1.4
SINGLE TANK
TWO TANKS
THREE TANKS
1.2
20%
70%
120%
PERCENTAGE OF WATER FILLED INSIDE TANK
TIME PERIOD
Fig. 28 Drift trends along y-axis
Fig. 29 Time period trends along x-axis
Fig. 30 Time period trends along y-axis
0.006
0.0055
0.005
0.0045
0.004
0.0035
0.003
SINGLE TANK
TWO TANKS
THREE TANKS
20% 70% 120%
PERCENTAGE OF WATER FILLED INSIDE TANK
7000
6500
6000
5500
5000
SINGLE TANK
TWO TANKS THREE TANKS
4500
20% 40% 60% 80% 100%
PERCENTAGE OF WATER FILLED INSIDE TANK
ACCELERATION
Fig. 31 Acceleration trends
-
CONCLUSION
From the results, it can be seen that for single tank condition,
-
On observing the displacement values, providing a water depth ratio of 20% gives the least displacement with 17.46% reduction in x-direction and 21.42% reduction in y-direction while compared to the displacement happening in condition of bare frame without water tank.
-
On observing the base shear values, providing a water depth ratio of 20% gives the least base shear value with 18.01% reduction in x-direction and 18.34% reduction in y-direction while compared to the base shear values of bare frame without water tank.
-
On observing the drift values, providing a water depth ratio of 20% gives the least drift values with 18.29% reduction in x-direction and 19.99% reduction in y-direction while compared to the drift values of bare frame without water tank.
-
On observing the time period values, providing a water depth ratio of 20% gives the least time period value with 3.76% increment in x-direction and 4.68% increment in y-direction while compared to the time period value of bare frame without water tank.
-
On observing the acceleration values, providing a water depth ratio of 40% gives the least acceleration with 23.1 increment in x-direction while compared with acceleration value of bare frame without water tank.
From the results, it can be seen that for two tank condition,
-
On observing the displacement values, providing a water depth ratio of 20% gives the least displacement with 25.7% reduction in x-direction and 32.15% reduction in y-direction while compared to the displacement happening in condition of bare frame without water tank.
-
On observing the base shear values, providing a water depth ratio of 20% gives the least base shear value with 29.24% reduction in x-direction and 30.65% reduction in y-direction while compared to the base shear values of bare frame without water tank.
-
On observing the drift values, providing a water depth ratio of 20% gives the least drift values with 29.2% reduction in x- direction and 31.76% reduction in y-direction while compared to the drift values of bare frame without water tank.
-
On observing the time period values, providing a water depth ratio of 20% gives the least time period value with 7.51% increment in x-direction and 8.59% increment in y-direction while compared to the time period value of bare frame without water tank.
-
On observing the acceleration values, providing a water depth ratio of 60% gives the least acceleration with 5.56% increment in x-direction while compared with acceleration value of bare frame without water tank.
From the results, it can be seen that for three tank condition,
-
On observing the displacement values, providing a water depth ratio of 20% gives the least displacement with 29.48% reduction in x-direction and 37.82% reduction in y-direction while compared to the displacement happening in condition of bare frame without water tank.
-
On observing the base shear values, providing a water depth ratio of 20% gives the least base shear value with 34.39% reduction in x-direction and 32.93% reduction in y-direction while compared to the base shear values of bare frame without water tank.
-
On observing the drift values, providing a water depth ratio of 20% gives the least drift values with 34.49% reduction in x-direction and 37.74% reduction in y-direction while compared to the drift values of bare frame without water tank.
-
On observing the time period values, providing a water depth ratio of 20% gives the least time period value with 10.52% increment in x-direction and 11.71% increment in y-direction while compared to the time period value of bare frame without water tank.
-
On observing the acceleration values, providing a water depth ratio of 40% gives the least acceleration with 4.15% reduction in x-direction while compared with acceleration value of bare frame without water tank.
-
On analysis of all the conditions, it has been found that the building gives its best seismic mitigation response on placing of three tanks as done in the model with the water tanks filled with 20% of their total capacity with water.
REFERENCES
-
Muhammad Jamil Ahmad, Qaiser uz Zaman Khan, Syed Muhammad Ali(2021) Use of Water Tank as Tuned Liquid Damper (TLD) for Reinforced Concrete (RC) Structures SPRINGER
-
Ali Ashasi-Sorkhabi, Hadi Malekghasemi and Oya Mercan(2013) Implementation and verification of real-time hybrid simulation (RTHS) using a shake table for research and education Journal of Vibration and Control
-
Yanhui Liu, Jinbiao Wu , Marco Donà (2018) Effectiveness of fluid viscous dampers for improved seismic performance of inter-storey isolated buildings Engineering Structures
-
Crowley, S. and Porter, R., (2011) Optimal Screen Arrangements for a Tuned Liquid Damper, School of Mathematics, University of Bristol
-
Chakraborty, S., Debbarma, R., and Marano, G. C., (2012) Performance of Tuned Liquid Column Dampers Considering Maximum Liquid Motion in Seismic Vibration Control of Structures, Journal of Sound and Vibration
-
Lotfollahi-Yaghin,M.,A., Ahmadi, H., and Tafakhor, H., (2016) Seismic Responses of an Offshore Jacket-Type Incorporated with Tuned Platform Liquid Dampers, Advances in Structural Engineering
-
Asma Belbachir, Abdelkader Benanane, Abderrahmane Ouazir, Zouaoui R. Harrat, Marijana Hadzima-Nyarko, Dorin Radu, Ercan Ik, Zouhir S. M. Louhibi, Sofiane Amziane., (2023) Enhancing the Seismic Response of Residential RC Buildings with an Innovative Base Isolation Technique Special issue Concrete structure design and health monitoring: enhancing resilience in face of disasters.
-
Luca Zuccolini, Eleanora Bruschi, Sara Cattaneo, Virginio Quaglini (2023) Current trends in Fluid viscous dampers with semi-active and adaptive behaviour Appl. Sci. 2023
-
Kamgar, R., Gholami, F., Sanayei, H. R. Z., and Heidarzadeh, H., (2019) Modified Tuned Liquid Dampers for Seismic Protection of Buildings Considering SoilStructure Interaction Effects, Iranian Journal of Science and Technology, Transactions of Civil Engineering
-
Bhattacharjee, E., Halder, L., Sharma R.P.(2013) An Experimental Study on Tuned Liquid Damper for Mitigation of Structural Response, Int. J. Adv. Struct. Eng. 5(3)
-
Das, S. and Choudhury, S., (2017) Seismic Response Control by Tuned Liquid Dampers for Low-Rise RC Frame Buildings, Australian Journal of Structural Engineering, ISSN: 1328-7982, 2017.
-
Chang, Y., Noormohamed, A., and Mercan, O., (2018) Analytical and Experimental Investigations of Modified Tuned Liquid Dampers (MTLDs), Journal of Sound and Vibration.
-
S. Javadinasab Hormozabad, SM Zahrai., (2019) Innovative adaptive viscous damper to improve seismic control of structures Journal of Vibration and Control.
-
Francisco J Martinez Martin, Victor Yepes, Fernando gonzalez Vidosa, Antonio Hospitaler, Julian Alcala., (2022) Optimisation design of RC elevated water tanks under seismic loads Appl. Sci. 2022, 12(11)
-
Rigoberto Nava Gonzalez, Adrian Pozos Estrada, Roberto Gomez Martinez, Oscar Pozos Estrada., (2024) Experimental investigation to evaluate the dynamic properties of a scaled rectangular tuned liquid damper using high speed videos Buildings 2024
-
Ayman Mohammad Mansour, Moustafa Moufid Kassem, Fadzli Mohamed Nazri., (2021) Seismic vulnerability assessment of elevated water tanks with variable staging pattern incorporating the fluid- structure interaction
Elsevier
-
Tanmoy Konar, Aparna Dey Gosh., (2020) Flow Damping Devices in Tuned Liquid Damper for Structural Vibration Control: A Review Archives of Computational Methods in Engineering.
-
Yonghui An, Zhongzheng Wang, Ge Ou, Shengshan Pan, and Jinping Ou., (2019) Vibration Mitigation of Suspension Bridge Suspender Cables Using a Ring-Shaped Tuned Liquid Damper 2019 American Society of Civil Engineers.
-
J.S. Love, T.C. Haskett, B.Morava, (2018) Effectiveness of dynamic vibration absorbers implemented in tall buildings Elsevier-Engineering Structures
-
Andrew S Ross, Ashraf El Damatty, Ayman M. El Ansary., (2015) Application of tuned liquid dampers in controlling the torsional vibration of high rise buildings Wind and Structures
-
Marius Tarpo, Christos Georgakis, Anders Brandt, Rune Brinker., (2021) Experimental determination of structural damping of a fullscale building with and without tuned liquid dampers Structural Control and Health Monitoring
-
JiuRong Wu, WenKun Zhong, JiYang Fu, Ching Tai Ng, LianYang Sun,
Peng Huang (2021) Investigation on the damping of rectangular water tank
with bottom- mounted vertical baffles: Hydrodynamic interaction and
frequency reduction effect Elsevier – Engineering Structures
-
Ali Pabarja, Moahmmadreza Vafaei, Sophia C. Alih, Mohd Yazmil Md Yatim, Siti Aminah Osman., (2019) Experimental study on the efficiency of tuned liquid dampers for vibration mitigation of a vertically irregular structure Elsevier – Mechanical Systems and Signal Processing
-
Fengwei Shi, Haipeng Wang, Liang Zong, Yang Ding, Junsheng Su., (2020) Seismic behavior of high- rise modular steel constructions with various module layouts Elsevier Journal of Building Engineering.
-
Amirahmad Fathieh, Oya Mercan (2016) Seismic evaluation of modular steel buildings. Elsevier Engineering Structures