
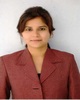
- Open Access
- Authors : Sugandha Singh , G N Tiwari
- Paper ID : IJERTV10IS060026
- Volume & Issue : Volume 10, Issue 06 (June 2021)
- Published (First Online): 14-06-2021
- ISSN (Online) : 2278-0181
- Publisher Name : IJERT
- License:
This work is licensed under a Creative Commons Attribution 4.0 International License
Thermal Analysis of Uneven Span Greenhouse integrated Semitransparent Photovoltaic Thermal System (GiSPVT)
a Sugandha Singh, b G. N. Tiwari
a Centre for Energy Studies, Indian Institute of Technology Delhi, Hauz Khas New Delhi, India -110016
bBag Energy Research Society (BERS), Plot No 51, Mahamana Nagar, Karaudi, Varanasi, UP, India-221005
Abstract:- According to the UN report, world population is estimated to be 9.8 billion by 2050. This demographic alteration will lead to exponential increase in energy and food demands. To meet the food security, aquaculture is one of the promising solutions which has increased at a faster rate in the past years and consequently; next decade is likely to witness a considerable rise in tailor-made and innovative sustainable solutions for aquaculture development. In this paper, an effort has been made to design a self-sustainable hybrid system for aquaculture, which includes uneven span greenhouse integrated semitransparent photovoltaic thermal (GiSPVT) system with aquapond. Energy balance equations as a function of climatic, operational and design parameters have been formulated and solved in MATLAB R2015b for the climatic conditions of New Delhi (Northern India) for a typical day of 15th January (Type a). Based on energy balance equations, analytical expression for solar cell temperature, greenhouse room air temperature, aquapond water temperature as well as solar electrical efficiency have been derived. The range of variation of electrical efficiency is from 10.4% to 11.08% and solar cell temperature varies between 10oC to 48.2oC. Attenuation factor of solar flux is 1.0 at the surface of water mass and decreases as the water depth increases. Temperature of the water of aquapond rises to 23.3oC during sunshine hours and later decreases slowly to a value of 9.03oC for a typical day of January, New Delhi, India. Moreover, temperature of greenhouse air is more than the ambient temperature at night by 5oC.
Keywords: Aquaculture, greenhouse, photovoltaic thermal, solar energy
-
INTRODUCTION
Harnessing of solar power is increasingly growing. The device powered by solar energy is being developed by several researchers. Solar radiation on the ground is 43% IR, 48% VIS and 9% UV radiation [1]. The ever scarcer traditional fuel supplies, especially fossil energy supplies, the requiste to decrease harmful atmospheric emissions, and emergency climatic conditions call for immediate action to introduce more environmentally friendly products to shift towards food security and system production in sustainable agriculture [2].
The most sustainable technology can be derived from the star Sun. Each amount of energy, predominantly or partially, consists of solar energy from Sun. Greenhouse technology practice harnessing solar energy for maintaining a controlled favorable environment for agriculture, aquaculture & algaculture.
Since the early 1960s, the global population has doubled, expected to overtake 9.8 billion people by 2050, leading to the exacerbation of the global "Food Security" issue as one of the most critical dimensions of sustainability. In contrast to the global downward trend in undernourishment, the number of people suffering from hunger has increased since 2014, so that the number of undernourished people has increased.
A growing trend followed, reaching approximately 784 million people in 2015, to reach 821 million people in 2017. Therefore, to ensure food security and reduce global hunger and poverty, investment in the agricultural sector is crucial. Fig.1. shows the number of undernourished people in the world from 2005 to 2018 [3].
Fig.1 Number of undernourished people in the world (2005-2018)
Revealed by the Food and Agriculture Organization (FAO) of the United Nations (FAO, 2019), the Early Warning Early Action (EWEA) study (2019) presented a progressive overview of the leading risks of disaster to the agricultural industry and food security. According to this study, a combined disruptive impact on food production and availability can be observed in many countries around the world with political conflicts and climate-related disasters. Continuing evidence shows that agriculture and food security are already affected by climate change, especially in countries where agricultural systems are more vulnerable to weather instability. Development in the agriculture sector is claimed to be up to 3.2 times more effective in reducing poverty than in other industries [4].
Mathematical-based models may also explain the thermal behavior of solar greenhouses. The models based on knowledge are correlated with physical parameters and use energy balance equations to explain the thermal behavior of solar greenhouses. The thermal environment of solar greenhouses may also be simulated by widely used software in commercial buildings with dramatically different greenhouse environments. The complex environment of greenhouses can be better defined by machine learning algorithms, but they are not sufficiently accurate since they can only provide the trustworthiest results for the training data set that is not always available for greenhouse [5].
-
Solar Photovoltaic
Also, the most easily usable, renewable, and clean power source available is solar photovoltaic technology (PV) to satisfy human energy demands. [6-8].
Solar photovoltaic has marked its potential in energy sector by contribution of 6000 TWh PV electricity till 2050, approximately 16% of global electricity consumption [9], as projected by the International Energy Agency (IEA). For this task, the absorption of solar energy into the atmosphere requires wide surface areas. Many of these requirements are met via rooftop solar panels or integrated PV (BIPV) building [10-13] and plenty more via PV farms on the field [14-17].
Also, Photovoltaic panels are an appealing technology in renewable energy because during their operation, they avoid large greenhouse emissions, have a long life estimate of 20 to 30 years, and use a reliable and abundant power resource [18]. For an atmosphere temperature of about 25oC the performance of a standard photovoltaic module is between 27-52oC. The whole thermal conduction can be used for greenhouse heating. This thermal energy extraction from photovoltaic energy also increases solar cell performance. Therefore, a photovoltaic device is referred to as PVT device as an optimized framework allowing use of thermal energy and electrical energy.
-
Hybrid photovoltaic system
Hybrid photovoltaic-thermal (PVT) system, which removes Photovoltaic modules heat with air and water as a workable fluid with an increase in monthly energy conversion efficiency by around 2.8 percent to 7.7 percent was introduced by Kern and Russel [19]. Across the world, many researchers have concluded that photovoltaic thermal (PVT) technologies can help in
increasing the solar cell efficiency. [20-26]. A combined hybrid PV/T screen that translates the solar energy into electrical and thermal energy has efficiency of 6.7% (electrical) and 33% (heat) [27].
The conduct of a photovoltaic and therapeutic (PV/T) collector installed into a black-plastic solar thermal absorber (unglazed PV/T system) by placing single crystal silicon cells. It proposed that the combined PV/T principle could be used for reduced temperature applications and to improve the PV system's efficiency level (e.g., building room heating) [28]. Each thermal conductivity of a PV/T hybrid air collector was calculated for New Delhi's composite atmosphere. A glazed PVT water collector's electrical and thermal outut was evaluated. Compared to a regular PV Module, the suggested PVT collector showed greater overall performance. [29].
Energy generation technologies are being developed as renewables becomes more popular, and the need for conventional energy sources is declining. Energy production from renewable energy sources, on the other hand, is dependent on weather conditions. Photovoltaic (PV) and other solar systems rely on solar radiation and ambient temperature, on the speed and direction of wind turbines, on the flow of river hydropower plants [30].
-
Green house photovoltaic system
Greenhouse technology integrated with photovolatic thermal (PVT), is one of the promising solution to exponential food and energy demands. These combination land and food issues may appear unmanageable, but they can be significantly strengthened by using agri-voltaics (dual land usage both for solar photovoltaics and agriculture) ]34-36[, aqua-voltaics (dual water use for solar photovoltaics and aquaculture) and smart international and cross disciplines. This dual use results in greater self- sustainable productivity overall.
Many scientists have investigated the problems of greenhouse heating by active method [37-40] and by the passive method in the recent years [41-42]. By water storage, rock bed storage, north wall, mulching, material shift, movable isolation and a thermal barrier, passive heating is possible. A thermal curtain or thermal shield for passive heating methods is one of the most useful and useful ways of reducing energy usage in greenhouses [43-44].
In the proposed research work, an uneven span greenhouse is considered which is integrated with semitransparent photovoltaic thermal (PVT) system. Also, a pond is considered inside the greenhouse, which can be used for thermal storage as well as for aquaculture and algaculture applications.
The researchers have done the study on the BIPV, Hybrid thermal energy and on solar PV. In these studies, the applications of photovoltaic have been described.
In this paper, an effort has been made to design a self-sustainable hybrid system for aquaculture, which includes uneven span greenhouse integrated semitransparent photovoltaic thermal (GiSPVT) system with aqua pond. Energy balance equations as a function of climatic, operational and design parameters have been formulated and solved in MATLAB R2015b for the climatic conditions of New Delhi (Northern India) for a typical day of 15th January (Type a).
-
-
SYSTEM DESCRIPTION
-
Uneven Span GiSPVT
The system consists of uneven span which implies that area of south roof is more than area of the north roof. Also, the walls are made up of glass to allow direct heating. South roof has 10kW PV system installed which enables indirect heating of the greenhouse air and also enables system to be self sufficient. Also, the size of the pond is (30*5*0.9)m as shown in fig 2.
Fig. 2: Schematic diagram of GiSPVT system
Table 1: Design Parameters of GiSPVT sytem
Parameters
Value
Parameters
Value
Aw
150.0 m2
Kg
0.78 W/m °C
Ab
150.0 m2
Lb
0.2 m
As
63.0 m2
Ls
0.3 m
Aew
19.0 m2
Lg
1.0 m
Asw
224.0 m2
Mw
75000 kg
Aww
19.0 m2
To
25 °C
Anw
224.0 m2
V
614.40 m3
Asr
121.23 m2
v
0-0.3 m/s
Anr
40.410 m2
w
0.90
Cw
4190 J/Kg o C
g
0.95
-
10kW PV system installed on south roof
To build the self sustainable system, a 10kW PV system is installed on the south roof of uneven span GiSPVT system. This includes 136 semitransparent PV modules whose specifications are summarized in Table 1. The efective area covered by PV system is 98m2
Table 2: Electrical design parameters of GiSPVT
S.No.
Parameters
Value
1
Peak Power
75 Wp
2
Peak Voltage
12 V
3
Effective area
0.72m2
4
Short cicuit current
4.8 A
5
Open circuit voltage
600mV
6
Module efficiency
15%
-
Working Principle
During the sunshine hours, solar radiations are incident on uneven span GiSPVT system, a part of which gets transmitted into the system directly through semitransparent PV modules i.e. direct gain from non packing area as well as the some part of it gets transmitted from indirect gain of the packing area. This thermal energy is partially absorbed by greenhouse room air and then by the water in the pond. After absorption in the water, there are convective, radiative and evaporative heat losses from the water to greenhouse room air. Also, heat losses occur from sides and bottom of the uneven span GiSPVT system by conduction.
-
-
THERMAL ANALYSIS
Following assumptions are made before thermal modeling of Uneven Span GiSPVT system
-
No stratification in the water column
-
Heat flow is one dimensional in a quasi steady state condition
-
Absorptivity and heat capacity of the enclosed air is neglected
-
Storage capacity of the material of the roof & walls is neglected
-
No radiative heat exchange between walls & roof Energy balance equation for south roof
I (t)A
[U (T _T ) h (TT )]A
I (t)A
g sc
sc s SR
t sc,s a
t sc,s
r sr
sc g
sc s SR
[Rate of solar radiation received at PV module] [Rate of heat loss from PV module to ambient] [Rate of heat transfer from PV module to greenhouse air] [Rate of electrical energy generated by the PV module](1)
Energy balance equation for greenhouse room air
h (T
T )]A
-
h (T T )]A
(UA)
(T T
) 0.33NV
t sc,s
r sr
-
w r w
eff r w
[Rate of heat transfer from PV module to greenhouse air ] [rate of heat transfer from solar pond to greenhouse air] [overall heat transfer from room to water ] [ rate of heat transfer due to inflitration](2)
Energy balance equation for solar pond
4
[ 2 (1 )I (t)A A II (t) A
] '
g sc s
SR g
-
i g n
i1
NR w
M C dTw h (T T )]A
U (T _ T )A
U (T _ T ) A
w w dt w w r w
b w b
s w s
[Rate ofincarese of water temperature]
Also, we know that
[rate of heat transfer from solar pond to greenhouse air] [Rate of heat transfer from bottom of solar pond] [Rate of thermal energy by conduction lost through sides of solar pond](3)
(1 (T T )) (4)
sc 0 o sc,s 0
Using equation (4) in equation (1), we get :
( I ) U T ( I )
t
t
t
t
T eff t a eff
(5)
sc,s
U h ( I )
eff
where, ( I )
eff
(
(
g sc sc
T )I (t
and ( I )
eff
I (t)
r
r
o o o o s
o o o o s
g sc o o s
g sc o o s
calculating for (Tsc,s T ) , we get
( I ) U T ( I ) U Tr h Tr ( I ) Tr
T T
eff t a eff t t eff
(6)
t
t
t
t
sc,s r
U h ( I )
eff
Multiplying by ht on both the sides, we get:
h (T T ) PF ( I )
ULT UL T
t sc,s r
1 eff
1 a 2 r
(7)
where, PF ht , UL htUt ,
t
t
t
t
t
t
t
t
1 h U
( I )
eff
1 h U
( I )
eff
UL
ht (Ut
( I )
eff )
t
t
t
t
2 h U
( I )
eff
using equation (7) & (2), we get
(PF ( I )
ULT UL T )A
-
-
h (T T )A
(UA)
(T T ) 0.33Nv(T T )
1 eff
1 a 2 r sr
w w r w
eff r a r a
On solving, above equation reduces to:
PF ( I )
A (UA)'' T
-
h T A
T 1
eff sr
eff a
w w w
w
w
r (UA)
3eff
-
h Aw
where, (UA)'' UL A
(UA)' & (UA)
UL A
(UA)'
eff 1 sr
Solving for (Tw-Tr)
eff
3eff
2 sr
eff
h (UA)
A T PFh
( I )
A A h
(UA)'' T A
h (T T )A w
3eff w w 1 w
eff sr w w
eff a w
w w r w
(UA)
3eff
-
h Aw
w
w
Multiplying by hwAw on both the sides, we get:
h (UA)
A T PFh
( I )
A A h
(UA)'' T A
h (T T )A w
3eff w w 1 w
eff sr w w
eff a w
w
w
w w r w
(UA)
3eff
-
h Aw
h (T T )A
UL T PF ( I )
UL T
(8)
w w r w
3 w 2
eff 4 a
where,
h A (UA)''
PF h A A
4
4
UL w w
eff
, PF
2 w w sr
hw Aw
-
(UA)
3eff
hw Aw
-
(UA)
3eff
2
2
using equation (8) in (3), we get
4
[ 2 (1 )I (t)A A II (t) A
] '
g sc s
SR g
i i g n
i1
NR w
(9)
M C dTw UL T PF ( I )
UL T U (T _ T )A
U (T _ T )A
w w dt 3 w 2
eff
4 a b w b
4
s w s
let, ( I )
[ 2 (1
)I (t)A
A I
I (t)A
]'
1eff g
sc s
SR g
i i g n
i1
NR w
Simplifying, equation (9), we get:
( I )
U T
A U T
A PF ( I )
M C
dTw (UL UL
U A U A )T
1eff
b b
s s 2
eff
w w dt
3 4 b b
s s w
Above equation can be rewritten as
f (t) dTw aT
dt w
on solving, we get
w
w
T f (t)
a
(1
1 eat
at
) T
1 eat
(
at
) (10)
wo
wo
-
Thermal circuit diagram
Fig. 3 Thermal circuit diagram of Uneven Span GiSPVT system
-
-
METHODOLOGY Heat and mass transfer correlations have been taken for the analysis.
Basic energy balance equations as a function of climatic, operational and design parameters have been written for each component of a given uneven span GiSPVT system.
Based on basic energy balance equations, analytical expressions for solar PV module, greenhouse room air, and water temperature have been derived.
For the proposed system, hourly variations in the temperature of water in pond, greenhouse air and solar cell temperature has been determined using IMD Pune weather data for New Delhi India
-
RESULTS AND DISCUSSION
Computational software MATLAB R2015b, has been used to solve the derived thermal model. The physical design considerations of Uneven Span GiSPVT system with aquapond have been given in Table 1. Also, ambient air temperature (Ta), solar intensity (It), wind velocity (v) and relative humidity inside greenhouse are the climatic parameters.
The hourly variations of solar intensity and ambient air temperature are shown in Fig. 4. Also, Fig.5 shows the hourly variations of greenhouse room air temperature, solar cell temperature, aquapond water temperature and ambient air temperature for a typical day of January, New Delhi (Type a weather conditions). This depicts that during sunshine hours, solar cell temperature increases gradually and reaches maximum to a value of 48.2oC at 1 pm and then gradually decreases. After sunshine hours, solar cell temperature is less than greenhouse air temperature and aquapond water temperature due to zero solar intensity. Also, greenhouse air temperature reaches its maximum value of 26.19oC at 2 pm while aquapond water temperature reaches its maximum value of 23.3oC at 3 pm. After sunshine hours, greenhouse air temperature is more than ambient air temperature by approximately 5oC.
Fig. 4 Hourly variations of solar intensity & ambient temperature
Fig. 5 Hourly variations of temperatures of Uneven Span GiSPVT
Solar cell temperature and electrical efficiency are inversely related as depicted from Fig. 6. The solar cell temperature varies from 10oC to 48.2oC while electrical efficiency ranges from 10.2% to 11.08% for a typical day of January, New Delhi (Type a weather conditions).
Fig. 6 Hourly variations of solar cell temperature & electrical efficiency
-
CONCLUSIONS
-
A thermal model has been developed for Uneven Span Greenhouse integrated Semitransparent Photovoltaic Thermal (GiSPVT) system
-
The PV modules electrical efficiency decreases with increase in average solar cell temperature.
-
Attenuation factor of solar flux is 1.0 at the surface of water mass and decreases as the water depth increases.
-
Temperature of the water of solar pond rises to 23.3oC during sunshine hours and later decreases slowly to a value of 9.03oC for a typical day of January, New Delhi, India.
-
Moreover, temperature of greenhouse air is more than the ambient temperature at night by 5oC
REFERENCES
[1]. B.A. Keating, M. Herrero, P.S. Carberry, J. Gardner, M.B. Cole, Food wedges: framing the global food demand and supply challenge towards 2050, Glob. Food Secur. 3 2014;125-132. [2]. J.Bundschuh, G.Chen, T.Yusaf, S.Chen, J.Yan, Sustainable energy and climate protection solutions in agriculture, Appl. Energ 114 2014; 735-736. [3]. Porter, John R., Liyong Xie, Andrew J. Challinor, Kevern Cochrane, S. Mark Howden, Muhammad Mohsin Iqbal, David B. Lobell, and Maria Isabel Travasso. "Food security and food production systems." (2014): 485-533. [4]. Gorjian, Shiva, Hossein Ebadi, Francesco Calise, Ashish Shukla, and Carlo Ingrao. "A review on recent advancements in performance enhancement techniques for low-temperature solar collectors." Energy Conversion and Management 222 (2020): 113246. [5]. Gorjian, Shiva, Francesco Calise, Karunesh Kant, Md Shamim Ahamed, Benedetta Copertaro, Gholamhassan Najafi, Xingxing Zhang, Mohammadreza Aghaei, and Redmond R. Shamshiri. "A review on opportunities for implementation of solar energy technologies in agricultural greenhouses." Journal of Cleaner Production (2020): 124807. [6]. Granovskii M, Dincer I, Rosen M. Greenhouse gas emissions reduction by use of wind and solar energies for hydrogen and electricity production: economic factors. Int J Hydrog Energy 2007; 32(8):92731. [7]. Pearce, Joshua M. "Photovoltaicsa path to sustainable futures." Futures 34, no. 7 (2002): 663-674. [8]. Barker PP, Bing M. Advances in Solar Photovoltaic Technology: An Applications Perspective, IEEE Power Engineering Society General Meeting; 2, 19551960; 2005. [9]. A.M. Pringle et al. Technology Roadmap: Solar Photovoltaic Energy, 2014. 581. Renewable and Sustainable Energy Reviews 80 2017; 572584. [10]. Alsema EA, Nieuwlaar E. Energy viability of photovoltaic systems. Energy Policy, viability Sol Photovolt 2000; 28(14):9991010. [11]. Wiginton, L. K., Ha T. Nguyen, and Joshua M. Pearce. "Quantifying rooftop solar photovoltaic potential for regional renewable energy policy." Computers, Environment and Urban Systems 34, no. 4 (2010): 345-357. [12]. Nguyen HT, Pearce JM, Harrap R, Barber G. The Application of LiDAR to assessment of rooftop solar photovoltaic deployment potential on a municipal district unit. Sensors 2012; 12:453458. [13]. Nguyen HT, Pearce JM. Automated quantification of solar photovoltaic potential in cities. Int Rev Spat Plan Sustain Dev 2013; 1(1): 5770. [14]. De Wild-Scholten MJ, Alasema EA, ter Horst EW, Bachler M, Fthenakis VM. A Cost and Environmental Impact Comparison of Grid-Connected of Rooftop and Ground Based PV Systems, 21st European Solar Photovoltaic Energy Conference; 2006. [15]. Nguyen HT, Pearce JM. Estimating Potential Photovoltaic Yield with r.sun and the Open-Source Geographical Resources Analysis Support System Solar Energy 84, 2010; 831-843. [16]. Bolinger M. Utility-Scale Solar 2012: An Empirical Analysis of Project Cost, Performance, and Pricing Trends in the United States; 2012. [17]. Fairley P. Big solar's big surge. Spectr, IEEE 2015; 52(1):414. [18]. M. Goe, G. Gaustad, Strengthening the case for recycling photovoltaics: an energy payback analysis, Appl. Energ 120 2014;41-48. [19]. Kern Jr, E.C., Russell, M.C., Combined photovoltaic and thermal hybrid collector systems, In: Proceedings of the 13th IEEE Photovoltaic Specialists.Washington, DC, USA, 1978; 11531157.
[20]. Ibrahim, A., Fudholi, A., Sopian, K., Othman, M.Y., Ruslan, M.H. Efficiencies and improvement potential of building integrated photovoltaic thermal (BIPVT) system. Energy Convers. Manag. 2014 ;77 527534. [21]. Dupeyrat, P., Ménézo, C., Fortuin, S.,. Study of the thermal and electrical performances of PVT solar hot water system. Energy Build. 2014 ;68, 751 755. [22]. Tiwari, G.N., Mishra, R.K., Solanki, S.C.Photovoltaic modules and their applications: A review on thermal modelling. Appl. Energy 88, 22872304. [23]. Atheaya, D., Tiwari, A., Tiwari, G.N., Experimental validation of a fully covered photovoltaic thermal compound parabolic concentrator system. Eng.Sci. Technol. Int. J. 19, 18451856. https://doi.org/10.1016/j.jestch.2016.06.014.
[24]. Lari, M.O., Sahin, A.Z.,. Design, performance and economic analysis of a nanofluid-based photovoltaic/thermal system for residential applications.Energy Convers. Manag. 2017; 149, 467484.
[25]. Preet, S., Bhushan, B., Mahajan, T.,. Experimental investigation of water based photovoltaic/thermal (PV/T) system with and without phase change material (PCM). Sol. Energy 155, 11041120. https://doi.org/10.1016/j.solener.2017.07.040. [26]. Sathe, T.M., Dhoble, A.S.,. A review on recent advancements in photovoltaic thermal techniques. Renew. Sustain. Energy Rev. 2017; 76, 645672. [27]. Zondag, H.A., de Vries, D.W., van Helden, W.G.J., van Zolengen, R.J.C. and Steenhoven, A.A.. The thermal and electrical yield of a PV-thermal collector. Solar Energy; 2002; 72 (2): 113-128. [28]. Sandnes, B. and Rekstad,. A. Photovoltaic/thermal (PV/T) collector with a polymer absorber plate: Experimental study and analytical model. Solar Energy, 72 (1): 2002; 63-73. [29]. Tiwari, A. and Sodha, M.S.. Performance evaluation of solar PV/T system: an experimental validation. Solar Energy, 2006, 80 (7), 751-759. [30]. Lokar, Jan, and Peter Virti. "The potential for integration of hydrogen for complete energy self-sufficiency in residential buildings with photovoltaic and battery storage systems." International Journal of Hydrogen Energy 45, no. 60 (2020): 34566-34578. [31]. Tawalbeh, Muhammad, Amani Al-Othman, Feras Kafiah, Emad Abdelsalam, Fares Almomani, and Malek Alkasrawi. "Environmental impacts of solar photovoltaic systems: A critical review of recent progress and future outlook." Science of The Total Environment (2020): 143528. [32]. Al-Ibrahim, A., Naif Al-Abbadi, and Ibrahim Al-Helal. "Pv greenhouse system¿ system description, performance and lesson learned." In International Symposium on Greenhouses, Environmental Controls and In-house Mechanization for Crop Production in the Tropics 710, pp. 251-264. 2004. [33]. Herez, Amal, Hicham El Hage, Thierry Lemenand, Mohamad Ramadan, and Mahmoud Khaled. "Review on photovoltaic/thermal hybrid solar collectors: Classifications, applications and new systems." Solar Energy 207 (2020): 1321-1347. [34]. Dupraz C, Marrou H, Talbot G, Dufour L, Nogier A, Ferard Y. Combining solar photovoltaic panels and food crops for optimising land use: towards new agrivoltaic schemes. Renew Energy Renew Energy: Gener Appl 2011; 36:272532. [35]. Marrou H, Wery J, Dufour L, Dupraz C. Productivity and radiation use efficiency of lettuces grown in the partial shade of photovoltaic panels. Eur J Agron 2013; 44:5466. [36]. Dinesh H, Pearce JM. The potential of agrivoltaic systems. Renew Sustain Energy Rev 2016; 54:299308. [37]. Connellan, G.. Solar greenhouse using liquid collectors. In: Proceedings of Solar Energy Society, Atlanta, GA, 1986. [38]. Santamouris, M., Mihalakakou, G., Balaras, C.A, Lewis, J.O., Vallindras, M. and Argiriou, A. Energy conservation in greenhouses with buried pipes.Solar Energy, 1996; 21 (5): 353-360.
[39]. Bargach, M.N., Tadili, R., Dahman, A.S. and Boukallouch, M.. Survey of thermal performances of a solar system used for the heating of agricultural greenhouses in Morocco. Renewable Energy, 2000; 20: 415-433. [40]. Jain, D. and Tiwari, G.N.. Modeling and optimal design of ground air collector for heating in controlled environment greenhouse. Energy Conservation and Management, 2003; 44 (8): 1357-1372. [41]. Tiwari, G.N. and Dhiman, N.K.. Design and Optimization of a winter greenhouse for the Leh-type climate. Energy Conservation and Management, 1986; 26(11): 71-78. [42]. Abak, K.A., Bascetincelik, A., Baytorun, N., Altuntas, Qu. and Ozturk, H.H. Influence of double plastic cover and thermal screens on greenhouse temperature, yield and quality of tomato. Acta Horticulturae, 1994; 369:149-154. [43]. Bailey, B.J.,. The evaluation of thermal screens in greenhouse on commercial nurseries. Acta Horticulture, 1981; 115: 663-670. [44]. Barral, J.R., Galimberti, P.D., Barone, A. and Miguel, A.L.. Integrated thermal improvements for greenhouse cultivation in the central part of Argentina. Solar Energy, 1999; 67 (1-3): 111-118.