
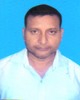
- Open Access
- Authors : Dr. Wakil Ray
- Paper ID : IJERTV9IS070338
- Volume & Issue : Volume 09, Issue 07 (July 2020)
- Published (First Online): 22-07-2020
- ISSN (Online) : 2278-0181
- Publisher Name : IJERT
- License:
This work is licensed under a Creative Commons Attribution 4.0 International License
Vibration and DFT Comparative Study of Pyridine with Cu, Ni and Zn Metals
Dr. Wakil Ray
Department of Physics
D. B.S.D Degree College Kadna, Saran,Bihar, India, 841311
Abstract:-Theoretical study of 3d transition metals Ni, Cu and Zn have been done. The comparasion of pyridine with 3d transition metals Ni Cu and Zn were optimized.. vibrational of spectra give fine changes at molecular levels because the vibration spectra results from the molecular vibration. The metal atoms Ni (II), Cu (II) and Zn (II) coordinated with pyridine through nitrogen atom of the ring. Raman and IR bands belongs to the vibrations of coordination. All studies will lead to better understanding of the effect of three different transition metals Ni, Cu and Zn with thebiological systems. Pyridine has C2V symmetry. The complexes have a symmetry.
Keywords:- Comparative Study of Pyridine ,DFT, Transition metals Cu, Ni and Zn.
-
INTRODUCTION
Gas-phase studies of metal ion-molecule complexes have provided a wealth of valuable information on binding energies, geometries, and the microscopic behaviour of chemical and physical processes. Such information is critical to the understanding of
lone-pair electrons on its nitrogen atom.[11] Because conjugation between a lone pair and the electrons of an aromatic ring has rendered nitrogen the favored basic site, it is reasonable to expect metal cations to orient themselves toward the N atom. The electrostatic model predicts that the metal ions are likely to align with pyridine dipole moment (2.19 D), [12] which is along the C2 axis. Because the lone-pair electrons and dipole moment coexist on the same plane and in the same direction, electron donation from pyridine to metal ions may be important for the pyridine complexes as well. Henson has theoretically studied Co(acacen)(pyridine) and Co(salen)(pyridine) complexes and shown that the nitrogen of the pyridine is bound to Co atoms[9].
Recently, the charge transfer process of Ag+-pyridine have been observed experimentally [13] and this report was later extended to experimental work to Cu+-pyridine and theoretical studies of Cu+ and Ag+ metal-pyridine complexes have been performed [14].
Attractiveness of copper ions in these compounds results mainly from the magnetic properties of Cu + ion, mixed-
heterogeneous catalysis, organ metallic reactions in 2 + +
condensed phase, and electron transfer involved in biological systems [1-4]. In addition to experimental investigations, theoretical studies have characterized detailed interactions between metal ions and molecules as well as provided binding energies. For calibration of experimental measurements, several theoretical methods (namely, HF, MP2, and DFT were applied in an attempt to approach accurate values and to establish trends in the metal ion-ligand systems [5-9]
Pyridine (C5H5N) is an aromatic molecule consisting of a -electron ring and a nitrogen atom. Pyridines (C5H5N) and their derivatives have been widely used in fields ranging from medicinal to industrial applications. The pyridine ring provides a potential binding site for metals and the information on their coordinating properties is important in understanding the role of metal ions in biological systems. Very few studies containing gas-phase interactions of pyridine with metal ions along with the experimental results have appeared so far. The only published results have been the formation of Ag+- (pyridine)n with n =1,2, 3, or 4 through gas-phase clustering reactions by Castle man [10] The binding energies of the third and fourth pyridines attached to Ag+ were deduced from the vant Hoff plot. However, the detailed interaction, geometry, and binding energy of bare Ag+ interacting with one pyridine molecule remain unknown. Mulliken noted that pyridine can be treated as a -type complex or as a -donor-type ligand because of the
valance oxidation-state pairs Cu2 /Cu , photoluminescence, structural features and biological relevance involving the binuclear site in cytochrome oxidases and related model compounds [8]. Having one different donor atoms pyridine ions can coordinate to metals through the nitrogen, giving rise to linkage isomers, dimers or polynuclear species with different dimensionality and nuclearity. According to the HSAB (hard soft acid base) theory, SCN ion coordinates to hard acids (Mn2+, Co2+ and Ni2+) through nitrogen atom, and the uncoordinated sulfur atom is involved in hydrogen bonds and sometimes involved in S. . .S interactions. If the transition metal center is soft acid (Cd2+, Cu+ and Hg2+) SCN ligand binds to central ion through sulfur atom. Cu2+ ion behaves as an intermediate Lewis acid and it is expected to bind the two sides of the SCN ligand, but with higher tendency to coordinate to the nitrogen [9-11]. A search in the CSD (The Cambridge Structural Database, Version 5.33) reveals 270 mononuclear copper(II) complexes containing N-coordinated thiocyanate groups and only 10 compounds with SCN bound to Cu centre via the S end [12].
Another interesting feature of Cu(II) complexes is the coordination geometry around the metal ion. Cu (II) ions can be four- six- and five-coordinate. The last ones usually display intermediate stereochemical environment ranging between trigonal and bipyramidal.
In the present work we have synthesized metal-ligand complexes of pyridine with three major d-block metals, viz. Ni, Cu and Zn and characterized these with the help of standard spectroscopic techniques. A comparative study of pure pyridine and its metal complexes have been done by theoretical DFT calculations [3] and experimental FT-IR and Raman spectra.
-
THEORETICAL
The optimized geometries of pure pyridine as well as of the complexes of pyridine with three different (3d) metals and the harmonic wavenumbers, Raman and IR intensities of different normal modes of pyridine and of all the complexes were calculated using the Gaussian 03 program package [15]. Theoretical DFT calculations were done using the hybrid functional that mixes the Lee, Yang and Parr functional for the correlation part and the Beckes three- parameter functional for the exchange part (B3LYP) [11,12]; employing the 6-31+G(d,p) and LANL2DZ basis sets and the geometries of the pure form of pyridine as well as of the pyridine-metal complexes were optimized. The vibrational wavenumbers of the pyridine were calculated at B3LYP/6-31+G(d,p) theoretical level. For optimizing the geometrical structures of the metal complexes of pyridine M(py)2Cl2 (where M = , Ni(II), Cu(II) and Zn(II)), we have used the B3LYP functional with LANL2DZ split-valence basis set.
-
EXPERIMENTAL
The pyridine liquid was purchased from Merck (Darmstadt, Germany) and directly used without further purification. FT-IR and laser Raman spectra for pure pyridine and three transition metals were obtained. The FT- IR spectrometer used was the PerkinElmer Spectrum 65 FT-IR spectrometer. The FT-IR spectra were recorded in the range 400-4000 cm-1 with the samples kept in the form of KBr pellets.
The laser Raman set-up consists of a 514.5 nm Ar+ laser delivering about 5 mW intensity as an excitation source, an Olympus 50x objective microscope for proper focussing of laser beam on desired portion of the sample and the Raman spectrometer (Renishaw RM 1000) having spectral resolution of ~1 cm-1. The Raman spectra were recorded in the range 200-3500 cm-1. The dispersion element of the spectrometer is a grating whose grating constant is 2400 grooves/mm. Spectrometer scanning, data collection and processing were done by a dedicated computer using Gram Wire software. The rigin 6.1 software was used for further analysis of band shape.
-
RESULTS AND DISCUSSION
In the present work the theoretical study of interaction of Pyridine with transition metal have been done. The structure of Pyridine with Zn Cu, Ni, and pure Pyridine were optimized. The optimization were done using density functional theory (DFT) at B3LYP functional, employing the LANL2DZ and 6-31+G(d,p) basis sets.The optimized structures are given in Figure 1.
Figure 1 : Optimized structures of (a) Pyridine(Py) (b) Py+Ni (c) Py+Cu (d) PY+Zn .
(a)
(b) (c)
(d)
The binding energy for Py+Zn, Py+Cu, Py+Ni, and Py pure are given in table 1.
Table 1: Dipole moments of pyridine and Different Transition metal complexes calculated by DFT method at B3LYP/ 6-
31+G(d,p) and LANL2DZ level.
Complex/molecule Dipole moment
(Debye)
Pyridine 02.38
Py + Ni 14.09
Py + Cu 13.20
Py + Zn 10.55
The Dipole Moments for Py+Zn, Py+Cu, Py+Ni, and Py pure are are also calculated and presented in table 2.
Table: 2 Theoretical calculated Charge Distribution of Pyridine and the Complex with Ni, Cu and Zn.
S.N. |
Atoms |
Charge Distribution |
|||
PY Pure |
PY + Ni |
PY + Cu |
PY + Zn |
||
1 |
C1 |
-0.260 |
-0.105 |
-0.150 |
-0.202 |
2 |
C2 |
0.180 |
-0.203 |
-0.191 |
-0.182 |
3 |
C3 |
-0.323 |
-0.163 |
-0.158 |
-0.155 |
4 |
C4 |
0.180 |
-0.201 |
-0.204 |
-0.183 |
5 |
C5 |
-0.260 |
-0.129 |
-0.117 |
-0.202 |
6 |
N6 |
-174 |
-0.220 |
-0.248 |
-0.260 |
7 |
H7 |
0.136 |
0.246 |
0.246 |
0.245 |
8 |
H8 |
0.129 |
0.253 |
0.296 |
0.292 |
9 |
H9 |
0.132 |
0.240 |
0.244 |
0.242 |
10 |
H10 |
0.132 |
0.244 |
0.240 |
0.242 |
11 |
H11 |
0.129 |
0.296 |
0.258 |
0.291 |
12 |
C12 |
-0.106 |
-0.117 |
-0.202 |
|
13 |
C13 |
-0.203 |
-0.204 |
-0.182 |
|
14 |
C14 |
-0.163 |
-0.158 |
-0.155 |
|
15 |
C15 |
-0.201 |
-0.191 |
-0.183 |
|
16 |
C16 |
-0.129 |
-0.150 |
-0.202 |
|
17 |
N17 |
-0.221 |
-0.248 |
-0.260 |
|
18 |
H18 |
0.246 |
0.246 |
0.245 |
|
19 |
H19 |
0.253 |
0.258 |
0.292 |
|
20 |
H20 |
0.240 |
0.240 |
0.242 |
|
21 |
H21 |
0.244 |
0.244 |
0.242 |
|
22 |
H22 |
0.296 |
0.296 |
0.291 |
|
23 |
Cl23 |
-0.298 |
-0.335 |
-0.485 |
|
24 |
Cl24 |
-0.298 |
-0.335 |
-0.485 |
|
25 |
Ni25 |
0.081 |
0.235 |
0.714 |
Pyridine has C2v symmetry. All the complexes have C1 symmetry. In vibrational spectra give fine changes at molecular levels because the vibrational spectra results from the molecular vibrations. In order to observe the fine charges, we have calculated the vibrational Raman and IR spectra of pure Pyridine and all the complexes of Pyridine. The Raman spectra of all the complexes and they are presented in Figure 2.
Figure 2: Raman Spectra for Pyridine with different transition metal complexes
The FT-IR spectra are presented in Figure 3.
Figure 3: FTIR Spectra for Pyridine with different transition metal complexes
Intensity / IR activity
Intensity / IR activity
Pyridine + Zn Complex
Pyridine + Cu Complex Pyridine + Ni Complex
Pyridine pure
0 600 1200 1800 2400 3000
Wavenumber / Cm-1
The vibration of harmonic wavenumbers along with Infrared and Raman activity depolar P and dpolar U factors are tabulated in Table 3.
Table: 3 Theoretical calculated Bond Length of Pyridine and the Complex with Ni, Cu and Zn.
Pyridine(PY) |
PY + Ni |
PY + Cu |
PY + Zn |
|||||||||
Atom1 |
Atom2 |
Bond Length |
Atom1 |
Atom2 |
Bond Length |
Atom1 |
Atom2 |
Bond Length |
Atom1 |
Atom2 |
Bond Length |
|
1 |
C1 |
C2 |
1.3976 |
C1 |
C2 |
1.4022 |
C1 |
C2 |
1.4049 |
C1 |
C2 |
1.4036 |
2 |
C1 |
N6 |
1.3405 |
C1 |
N6 |
1.3605 |
C1 |
N6 |
1.3607 |
C1 |
N6 |
1.3616 |
3 |
C1 |
H8 |
1.0881 |
C1 |
H8 |
1.084 |
C1 |
H8 |
1.0852 |
C1 |
H8 |
1.0856 |
4 |
C2 |
C3 |
1.3958 |
C2 |
C3 |
1.4071 |
C2 |
C3 |
1.4067 |
C2 |
C3 |
1.4077 |
5 |
C2 |
H9 |
1.0856 |
C2 |
H9 |
1.0852 |
C2 |
H9 |
1.0852 |
C2 |
H9 |
1.0852 |
6 |
C3 |
C4 |
1.3957 |
C3 |
C4 |
1.4071 |
C3 |
C4 |
1.4085 |
C3 |
C4 |
1.4077 |
7 |
C3 |
H7 |
1.0863 |
C3 |
H7 |
1.0865 |
C3 |
H7 |
1.0866 |
C3 |
H7 |
1.0868 |
8 |
C4 |
C5 |
1.3976 |
C4 |
C5 |
1.4026 |
C4 |
C5 |
1.4022 |
C4 |
C5 |
1.4035 |
9 |
C4 |
H10 |
1.0856 |
C4 |
H10 |
1.085 |
C4 |
H10 |
1.0853 |
C4 |
H10 |
1.0852 |
10 |
C5 |
N6 |
1.3405 |
C5 |
N6 |
1.3622 |
C5 |
N6 |
1.3617 |
C5 |
N6 |
1.3616 |
11 |
C5 |
H11 |
1.0882 |
C5 |
H11 |
1.0824 |
C5 |
H11 |
1.0853 |
C5 |
H11 |
1.0857 |
12 |
N6 |
Ni25 |
1.9563 |
N6 |
Cu25 |
2.0889 |
N6 |
Zn25 |
2.1359 |
|||
13 |
C12 |
C13 |
1.4021 |
C12 |
C13 |
1.4022 |
C12 |
C13 |
1.4035 |
|||
14 |
C12 |
N17 |
1.3606 |
C12 |
N17 |
1.3616 |
C12 |
N17 |
1.3616 |
|||
15 |
C12 |
H19 |
1.0839 |
C12 |
H19 |
1.0854 |
C12 |
H19 |
1.0857 |
|||
16 |
C13 |
C14 |
1.4072 |
C13 |
C14 |
1.4085 |
C13 |
C14 |
1.4078 |
|||
17 |
C13 |
H20 |
1.0851 |
C13 |
H20 |
1.0852 |
C13 |
H20 |
1.0852 |
|||
18 |
C14 |
C15 |
1.407 |
C14 |
C15 |
1.4068 |
C14 |
C15 |
1.4077 |
|||
19 |
C14 |
H18 |
1.0865 |
C14 |
H18 |
1.0866 |
C14 |
H18 |
1.0866 |
|||
20 |
C15 |
C16 |
1.4028 |
C15 |
C16 |
1.4049 |
C15 |
C16 |
1.4034 |
|||
21 |
C15 |
H21 |
1.085 |
C15 |
H21 |
1.0851 |
C15 |
H21 |
1.0852 |
|||
22 |
C16 |
N17 |
1.3624 |
C16 |
N17 |
1.3607 |
C16 |
N17 |
1.3616 |
|||
23 |
C16 |
H22 |
1.0824 |
C16 |
H22 |
1.0853 |
C16 |
H22 |
1.0856 |
|||
24 |
N17 |
Ni25 |
1.9557 |
N17 |
Cu25 |
2.0891 |
N17 |
Zn25 |
2.1361 |
|||
25 |
Cl23 |
Ni25 |
2.2346 |
Cl23 |
Cu25 |
2.2927 |
Cl23 |
Zn25 |
2.3271 |
|||
26 |
Cl24 |
Ni25 |
2.2324 |
Cl24 |
Cu25 |
2.2928 |
Cl24 |
Zn25 |
2.3281 |
Binding energies and structures also are presented and analyzed herein.
The metalligand binding modes of the theoretically calculated as well as experimentally observed spectra of the complexes confirmed that the metal(II) atom coordinated with pyridine through the endocyclic nitrogen atom. This observation was further verified by observing the Raman and IR wavenumber shifts corresponding to C- H stretching and ring stretching, bending and out of plane bending vibrations.
CONCLUSION
Theoretical study of interaction of Pyridine with transition metal have been done. The structure of Pyridine with Zn Cu, Ni, and pure Pyridine were optimized. Pyridine has C2v symmetry. All the complexes have C1 symmetry. In vibrational spectra give fine changes at molecular levels because the vibrational spectra results from the molecular vibrations. Binding energies and structures also are presented and analyzed herein. The metal atoms of Ni(II), Cu(II) and Zn(II) coordinated with pyridine through nitrogen atom of the ring. On coordination Raman and IR bands belonging to vibrations of coordination region were observed in the DFT derived spectra. The bands belong to the ring and C-H group appearing in the higher wavenumber region also shifted in the experimental Raman and IR spectra. This study and further similar study will lead to better understanding of the effect of coordination of metals with the biological systems.
ACKNOWLEDGEMENT
I express my profound sense of Gratitude and sincere thank to my supervisor Pro. Achyuta Nand Singh, Professor Department of physics J.P.U Chapra. I am grateful to Pro-
Ranjan Kumar Singh Professor Department of physics BHU for being a source of constant encouragement and for giving his valuable suggestion during the whole research work. I am also thankful to all my colleagues and other staff of DBSD Degree College Kadna .
REFERENCES
[1] Sauer, J. Chem. Rev. 89 199 ( 1989) .-
Ba¨ckvall, J.E., Bo¨kman, F. Blomberg. J. Am. Chem. Soc. 114
534 (1992)
-
Dougherty, D. A. Science 271 163 (1996)
-
Szilagyi, R. K.; Frenking, G. Organometallics 16 4807 (1997)
-
Bauschlicher, C. W., Partridge, Langhoff, S. R. J. Phys. Chem.
96 3273 (1992)
-
Luna, A., Amekraz, B.,Tortajada, J. Chem. Phys. Lett. 266 31 (1997)
-
Ma, N. L., K. M., Tsang, C. W.nChem. Phys. Lett., 277 306 (1997)
-
Nicholas, N. J., Hay, B. P., Dixon. J. Phys. Chem. A, 103 1394 (1999)
-
Henson, N. J., Hay, P. J., Redondo, A. Inorg. Chem. 38 1618 (1999)
-
P. M., Castleman, A. W. J. Chem. Phys. 76 4195 (1982)
-
Mulliken, R. S. J. Am. Chem. Soc. 64 811 (1952)
-
Lide, D. R. CRC Handbook of Chemistry and Physics, 79th ed., CRC Press: London (1998)
-
Yuh-Sheng Yang, Wen-Yi Hsu, Hsiu-Fang Lee, Ya-Chien Huang, and Chen-Sheng Yeh, J. Phys. Chem. A, 103 11287- 11292 (1999)
-
M.J. Frisch, G.W. Trucks, H.B. Schlegel, G.E. Scuseria, M.A. Robb (2003)